Chapter 22 Marco Senzolo1 and A.K. Burroughs2 1Multivisceral Transplant Unit, Gastroenterology, Department of Surgical and Gastroenterological Sciences, University Hospital of Padua, Padua, Italy 2The Royal Free Sheila Sherlock Liver Centre and University Department of Surgery, Department of Intensive Care, Royal Free Hospital, London, UK The liver has a key role in blood coagulation being involved in both primary and secondary hemostasis [1]. It is the site of synthesis of most coagulation factors and their inhibitors except for von Willebrand factor (vWf) [2]. Liver failure is accompanied by multiple changes in the hemostatic system, because of reduced plasma levels of procoagulative and anticoagulative clotting factors synthesized by the intact liver [3]. Therefore, the hemostatic system is in a delicate balance between prothrombotic and antithrombotic processes, aiming to prevent excessive blood loss from injured vessels and prevent spontaneous thrombosis. Moreover, during liver failure, there is a reduced capacity to clear activated hemostatic proteins and protein inhibitor complexes from the circulation. Thus, the global effect of liver disease with regard to hemostasis is complex, so that patients with advanced liver disease can experience severe bleeding or even thrombotic complications. Finally, when marked portal hypertension develops with secondary splenomegaly, thrombocytopenia develops due to splenic sequestration, but thrombocytopenia may also be due to decreased hepatic thrombopoietin synthesis. Demonstrated decreased platelet function could be balanced by the concomitant increase in vWf. This delicate hemostatic balance can be easily be tipped towards bleeding or thrombosis by superimposed conditions such as infections. The liver is the site of synthesis of fibrinogen and factors II, V, VII, IX, X, XI, and XII [4]; vWf is synthesized by the endothelium [5]. Factor VIII is synthesized mainly by the hepatic sinusoidal endothelial cells [6,7], but also by endothelial and nonparenchymal cells in the kidney, spleen, lungs, and brain [8]. Thus, the plasma concentration of factor VIII, which is one of the most procoagulant factors, is not decreased with liver disease, but it has been shown to be increased [9]. This occurs possibly because of increased endothelial synthesis and reduced clearance via low density lipoprotein receptor-related protein [5] and increased vWf [10]. However, the biologic activity of the synthesized molecule is lower than the plasma concentration [11]. Vitamin K is an essential cofactor for the production of biologically active forms of the coagulation factors II, VII, IX, and X. It promotes hepatic post-ribosomal conversion of certain glutamic acid residues in the protein precursors, to gamma-carboxyglutamic acid (Gla). These active forms of the clotting factors chelate calcium at the Gla site resulting in effective hemostatic function. In chronic liver disease, the gamma-carboxylation is impaired due to deficiency or antagonism of vitamin K, and inert precursors are synthesized (known as proteins induced by vitamin K absence, PIVKA) and released into the bloodstream [12]. The clinical significance of these precursors is not clear. In cholestasis, reduction of vitamin K absorption from the small intestine due to decreased bile salt production can be compensated by parenteral administration of 10 mg/day vitamin K for 24–48 hours, but in parenchymal liver disease, decreased levels of coagulation factors are dependent on a decreased synthesis, so that there is no improvement with vitamin K administration [13]. This therapeutic trial can be used to assess the eventual vitamin K deficit. Nevertheless, 25% of patients with acute liver injury have a subclinical deficit of vitamin K that may benefit from parenteral administration with corresponding improvement of the international normalized ratio (INR) [14]. However, this approach is not recommendend due to alterations in prognostic scores in liver failure and possible changes in indications to liver transplantation. In acute liver failure, the first factors whose plasma concentrations fall are those with the shortest half-life, factors V and VII (12 hours and 4–6 hours, respectively), and factors II, VII, and X will subsequently also be found to be low [15]. Factor VIII, together with vWf, is usually elevated, whereas there is only a slight reduction of factors IX and X. The differential effects on clotting factor levels during acute liver failure occur because high levels of cytokines increase levels of tissue factor which activate factors II, V, VII, X, whereas any thrombin generated is inhibited by antithrombin III, preventing activation of factors VIII, XI, and consequently XI, thus preserving their plasma levels [9]. Higher plasma des-gamma-carboxy (PIVKA) prothrombin concentrations are found in patients with hepatocellular carcinoma, due to local production by tumor cells. This abnormal prothrombin is thought to be a growth factor for this tumor and to be associated with poor prognosis [16]. Plasma concentration of vWf is substantially increased in patients with acute liver failure, due to its increased synthesis as an acute phase protein in response to tissue injury [17–19]. With liver injury this may also be secondary to endotoxemia associated with endothelial dysfunction [5]. In chronic liver disease, endothelial shear stress related to portal hypertension may also contribute to the high plasma levels of vWf via a nitric oxide (NO) stimulus [20]. Elevated levels of vWf seem to support platelet adhesion despite their reduced functional capacity [21]. Moreover, increased levels of high molecular weight vWf multimers have been found to be increased in end-stage liver disease, due to the concomitant decrease in ADAMTS13 (a disintegrin-like and metalloproteinase with thrombospondin type-1 motifs 13) which specifically cleaves multimeric vWf between Tyr1605 and Met 1606 [22]. These high molecular weight multimeric forms may be responsible for microthrombi formation in the terminal branches of portal venules, further aggravating cirrhosis. A correlation between severity of liver disease, hepatic venous pressure gradient (HVPG) and vWf plasma antigen levels has been documented and vWf higher than 315% has been shown to be a good predictor of liver decompensation and death in a cohort of 286 patients [23]. Plasma fibrinogen is an acute-phase reactant, and remains normal or increased in patients with liver disease [24]. Lower levels due to decreased synthesis, yet above 100 mg/dL, have been reported only in patients with very severe liver disease [25]. However, this synthesized form is a nonfunctional fibrinogen. About 60–70% of patients with liver disease have nonfunctional forms of fibrinogen due to an increased activity of sialyltransferase expressed by immature hepatocytes generated during hepatic injury. This leads to a low molecular weight fibrinogen with abnormal α chains and higher sialic acid content [26]. This counterbalances the high fibrinogen concentrations found in patients with chronic hepatitis, cholestatic jaundice, and hepatocellular carcinoma, which does not result in increased clotting ability. Clinically, this results in an abnormal thrombin time (TT), despite almost normal prothrombin time (PT) and partial thromboplastin time (PTT), with an apparently normal concentration of fibrinogen. This abnormality is reversed following recovery of liver function and after liver transplantation [27]. Abnormalities in both number and function of platelets are common in patients with liver disease and contribute to the impaired hemostasis seen in these patients. About 70% of patients with chronic liver disease develop thrombocytopenia, which is usually mild to moderate (70–90 × 109/L), and worsens with disease progression and increased hypersplenism which increases platelet sequestration [28–30]. Severe thrombocytopenia defined as platelet count less than 20 × 109/L occurs in only 1% of patients [31]. Thrombocytopenia has not been associated with an increased risk of bleeding from esophageal varices (i.e., structural bleeding) or other sources, although there are only few studies evaluating this, but it has been shown to be correlated with blood loss during surgery (i.e., hemostatic bleeding) [32]. A higher spleen diameter : platelet count ratio has been shown to have a high predictive value for the presence of esophageal varices in patients with cirrhosis [31]. The issue of sequestration versus other causes of thrombocytopenia in cirrhosis has been evaluated recently by comparing platelet number in extrahepatic portal hypertension, with that of cirrhotic patients having a similar-sized spleen. This has shown less severe thrombocytopenia in the noncirrhotic patients [33]. Synthetic function of the liver is essential for platelet production via thrombopoietin, which regulates platelet production in the bone marrow [34]. Although thrombopoietin is increased in patients with thrombocytopenia due to a homeostatic response [35], this occurs to a lesser degree with severe or chronic liver diseases than in those subjects with a normal liver [36]. Lower thrombopoietin mRNA levels in cirrhotic liver tissue [37] have been shown, confirming impaired thrombopoietin synthesis. In addition, a low platelet production from the bone marrow in cirrhotic patients has been shown [38]. Hepatitis C virus (HCV) infection [39], acute viral infection, alcohol abuse, and folate deficiency can result in myelosuppression [40], further lowering platelet counts. Consumption coagulopathy is not common in cirrhosis and even if disseminated intravascular coagulation (DIC) is present at a chronic low level, it does not influence platelet count [41,42]. Thrombocytopenia may also be contributed to by immune-mediated mechanisms due to an increase in production from B cells of antibodies binding platelet surface antigen GPIIb-IIIa and GPIb/I, which has been shown in viral-related cirrhosis B and C [43] and cholestatic liver diseases (primary biliary cirrhosis, PBC; primary sclerosing cholangitis, PSC) [44]. Among 368 patients with viral-induced cirrhosis, elevated titers of platelet-associated immunoglobulin G were observed in 88% with HCV and 47% with hepatitis B virus (HBV) infection [39]. Platelet function, as well as decreased platelet number, has been shown to be impaired in patients with liver disease with several laboratory activation tests [45]. Platelet aggregation in response to ADP, arachidonic acid, collagen, and thrombin is subnormal, probably due to a defective signal transduction mechanism [46]. Intrinsic defects [47] and abnormal plasma factors [48] have also been shown to contribute to platelet function abnormalities. An abnormal content of arachidonic acid in platelet membranes is found in liver disease and induces less aggregation ability mediated by thromboxane A2 [49]. In plasma from cirrhotic patients, the presence of an abnormal high-density lipoprotein (HDL) may alter NO content in the cell, leading to abnormal aggregability [50]. However, more recent data have questioned this hypothesis. Urinary excretion of 11-deydro-thromboxane (tx) B2, a stable metabolite of TxA2, was increased in cirrhosis suggesting activation of platelets [51]. Moreover, recent flow cytometry analysis of platelet activation, as well as platelet-monocyte aggregates, is consistent with hyperactivation of platelets in cirrhosis [52]. These recent findings could be explained by the activation of platelet adhesion by thrombin which is increased in cirrhosis and elevated levels of vWf, especially its high molecular multimeric forms. Cholestatic liver diseases that can demonstrate a normal or hypercoagulable state by thromboelastography [53] have normal or hyperactive platelet function when assessed by platelet function assay (PFA-100) closure time and flow cytometric study of receptors [54]. When the platelet number is too low, cytometry or aggregation studies may be difficult to interpret; thromboelastography, which is a global test of clot formation and dissolution, measures both platelet function and number by maximum amplitude parameter [55], and can be used to assess platelet function. Splenectomy is generally contraindicated in patients with cirrhosis, because of the high mortality rate and risk of secondary portal vein thrombosis, leading to increased risk of bleeding from esophageal-gastric varices and more difficult surgery during subsequent liver transplant [56]. Splenic embolization with 30–50% reduction in flow can normalize or significantly improve platelet number in some patients with cirrhosis [57] and may be helpful if invasive procedures such as embolization of hepatocellular carcinoma or interferon therapy for viral hepatitis are required. Insertion of transjugular intrahepatic portosystemic shunt (TIPS) has been shown to increase, but not to normalize, platelet number in some cases [58,59]. Antithrombin III is a non-vitamin-K-dependent glycoprotein synthesized by the liver but also by the endothelium [60]. Its plasma concentration decreases in patients with liver disease, in parallel with the severity of liver dysfunction, probably due to reduced synthesis and/or increased consumption due to hyperfibrinolysis [61]. Usually, the antithrombin III deficit is mild and thrombosis as a complication is very rare, reported only sporadically [62]. The decrease in antithrombin III may be responsible for the demonstrated low plasma levels of anti-Xa activity found in patients with cirrhosis undergoing therapy with low molecular weight heparin [63]. However, the decreased level of anti-Xa activity does not correspond to a reduced anticoagulation response in patients with liver disease compared with control subjects [64]. Proteins C and S are vitamin-K-dependent glycoproteins synthesized mainly by hepatocytes [65]. Therefore, during acute or chronic liver disease, their concentrations can be decreased, but usually not below 20% of normal values [66]. In patients with liver disease who also have genetic deficiency, plasma concentration is often lower than 20%. When there is severe liver disease, it can be difficult to exclude coexistent genetic deficiency as levels may be very low, due to very depressed synthesis [67]. In this situation a concomitant finding of a normal level of factor II and protein C: factor VII ratio can help to confirm a coexistent genetic deficit [68]. In acquired deficiency of vitamin K, a defective protein C lacking gamma-carboxyl (PIVKA) is produced [1]. In cirrhotic patients, the decreased level of protein C causes resistance to thrombomodulin activation which increases thrombin generation compared with control subjects, especially in Child C patients in whom the imbalance between factor VIII and C is greater. Protein C deficiency is not associated with extrahepatic portal vein thrombosis [69]. Genetic deficiency of protein S is extremely rare, yet accounts up to 7% of patients with Budd–Chiari syndrome (BCS) or portal vein thrombosis, especially in series from Asia [70]. All the proteins involved in fibrinolysis, except for tissue plasminogen activator (tPA) and plasminogen activator inhibitor-1 (PAI-1), are synthesized in the liver, and indeed reduced plasma levels of plasminogen [71], α2-antiplasmin, histidine-rich glycoprotein [72], factor XIII [73], and thrombin-activable fibrinolysis inhibitor (TAFI) [71] are documented in patients with cirrhosis. Conversely, tPA levels are increased in liver disease, due to decreased clearance, whereas its inhibitor PAI-1 is normal or only slightly increased in plasma. Therefore, the insufficient inhibitor concentrations accounts for net increased fibrinolysis [74]. Hyperfibrinolysis is correlated with the severity of liver dysfunction in cirrhosis as assessed by Child–Pugh score [75]. Ascitic patients show a greater degree of fibrinoslyis, because ascitic fluid has increased fibrinolytic activity: up to 20 L is reabsorbed daily, with fibrinolysis being correlated with endotoxin levels [76]. Increased levels of D-dimers, prothrombin fragments 1+2 (F1+2) fibrin degradation products and plasmin–α2-antiplasmin complexes are found [77]. Many studies using different methodologies demonstrate hyperfibrinolysis (thromboelastography [78], diluted whole blood clot lysis assay [79], and euglobin clot lysis time [80]). Recently, TAFI has been evaluated: it is decreased by an average of 26% in cirrhosis and by 50% in acute liver failure [81,82]. However, there is some controversy regarding hyperfibrinolytic activity in cirrhotic patients, as not all studies have confirmed this. This may due to the particular in vitro assay used. In contrast, in vivo correlation between hyperfibrinolysis and an increased risk of variceal bleeding, compatible with the circadian rhythm of hyperfibrinolytic activity has been shown [83]. In addition, increased fibrinolytic activity during liver transplantation and hepatic resection correlates with blood loss [84]. Interestingly, patients with cholestatic liver disease are characterized by a normal or hypercoagulable state: higher PAI-1 concentrations are seen than with other etiologies, balancing the increased tPA activity. This results in less hyperfibrinolysis in the reperfusion phase during liver transplantation, and antifibrinolytic therapy is not usually administered [85]. Thus, the clinical issue is whether cirrhotic patients when under “stress” (e.g., during infection, surgery, or bleeding) exhibit increased fibrinolysis, resulting in an increased bleeding tendency, which is not manifest when patients are stable. DIC is characterized by intravascular fibrin deposition due to activation of the clotting cascade, which overwhelms the anticoagulation pathway. DIC causes consumption of coagulation factors and platelets, and secondary fibrinolysis occurs, causing an increased bleeding tendency [86]. Hemostatic abnormalities that are present in cirrhotic patients share common laboratory features with DIC (i.e., an prolonged PT and PTT, low fibrinogen level, elevated fibrin-degradation product and D-dimer, and thrombocytopenia) [87–89]. However, despite DIC-like laboratory abnormalities (so-called pseudo-DIC) being observed, autopsy studies in cirrhotics have shown little evidence for fibrin deposition and clinically manifest DIC is very rare [87]. More sensitive tests, quantifying proteolytic cleavage products of the coagulation reaction (i.e., fibrinopeptide A, F1+2, and fibrinolysis reactions (fibrin D-dimer, high molecular weight fibrin–fibrinogen complexes or soluble fibrin)), demonstrate an abnormal profile called the accelerated intravascular coagulation and fibrinolysis phenomenon (AICF) in about 30% of cirrhotic patients [78,90]. However, Ben Ari et al. [78] analyzed 52 patients with stable liver disease showing that despite normal F1+2 thrombin–antithrombin III complex and D-dimer levels, thromboelastographic studies were able to detect hyperfibrinolysis. AICF may be important in the portal venous system, as this phenomenon is more pronounced there than in systemic blood. This could be related to higher levels of endotoxemia in portal blood, which can trigger release of interleukin 6 (IL-6) and tumor necrosis factor α (TNF-α), thus activating intravascular coagulation [91]. Thrombotic complications can paradoxically occur in cirrhotic patients even if clinically they are regarded as having hemorrhagic tendencies. Despite prolonged coagulation tests, these patients cannot be viewed as being “anticoagulated,” due to the demonstratated normal thrombin generation due to the contemporary decrease in synthesis of both pro- and anticoagulant factors [3]. Wanless et al. [92] contend that portal vein and hepatic vein microthrombi cause disease progression in cirrhotic patients. Hepatic vein and portal vein thrombosis was found in at least 70% and 36%, respectively, of explanted livers and were associated with regions of confluent fibrosis (focal parenchymal extinction). The formation of microthrombi could be due to the increased platelet aggregation due to increased high weight multimeric forms of vWf [21] or a combination of increased blood flow and imbalance between pro- and anticoagulation factors in patients with advanced cirrhosis, which results in increased thrombin generation [93]. Moreover, increased thrombin generation due to factor V Leiden mutation was associated with a 3.28-fold increased risk of rapid fibrosis progression in a Caucasian hepatitis C cohort [94]. The proposed pathogenic mechanism lies in thrombin’s wide range of biologic activity, which includes amplification of Par-1 signaling leading to activation of stellate cells [95].
Coagulopathy and Clotting Disorders
Introduction
Hemostatic Factors
Procoagulant Factors
Von Willebrand Factor
Fibrinogen
Platelets
Anticoagulant Factors
Antithrombin III
Proteins C and S
Disorders of the Fibrinolytic System
Disseminated Intravascular Coagulation and Accelerated Intravascular Coagulation
Assessment of the Risk of Thrombosis and Anticoagulation
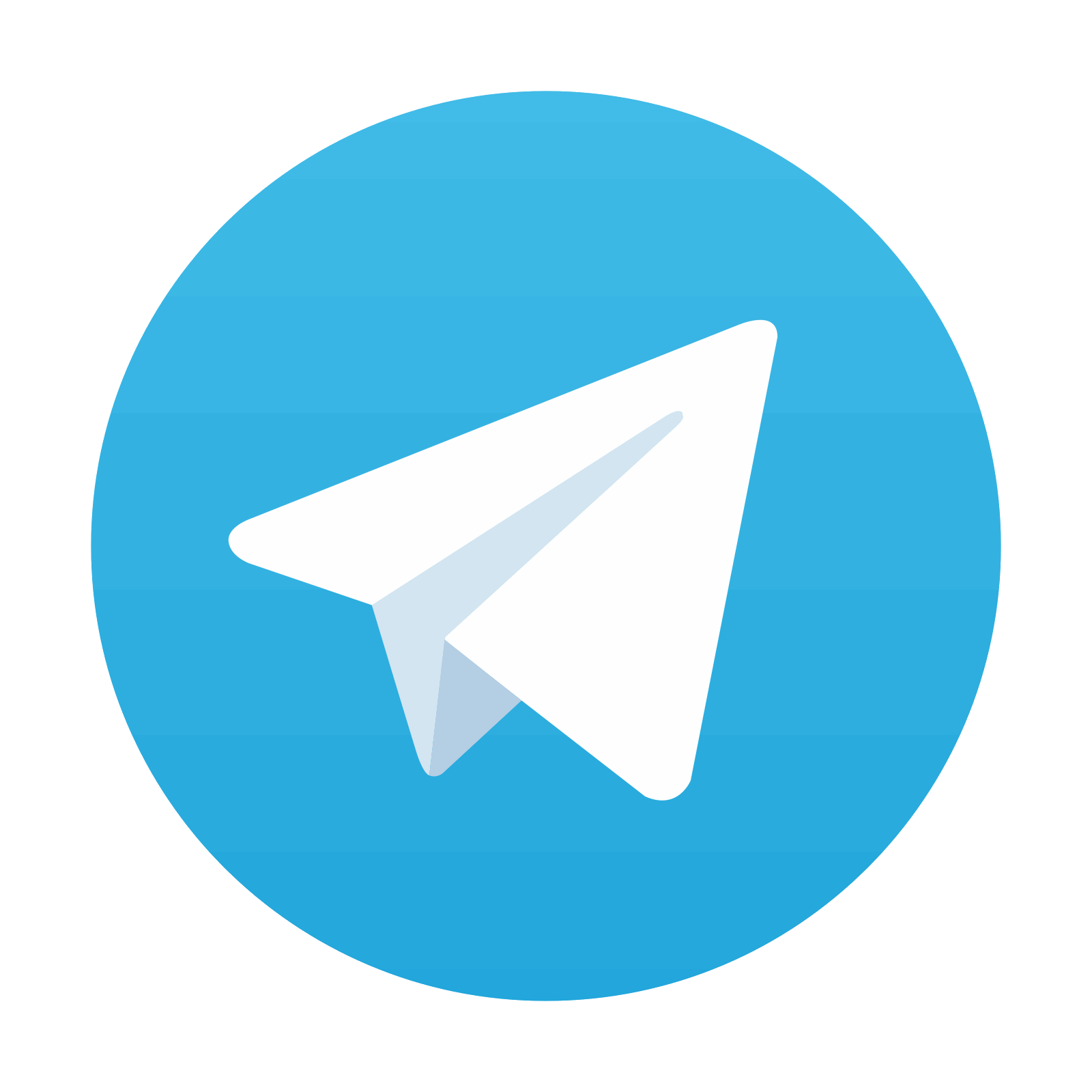
Stay updated, free articles. Join our Telegram channel

Full access? Get Clinical Tree
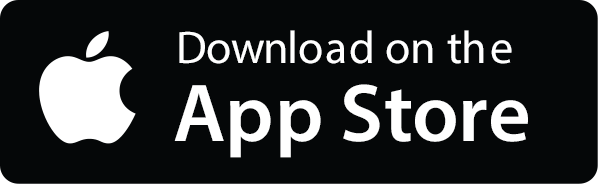
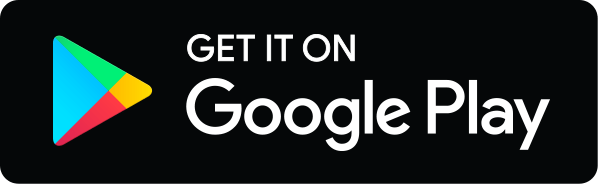