Approximately 1.25 million people burned annually in the United States, where 30,000 patients require admissions to burn centers every year and about 3400 die.1,2 Burns requiring hospitalization typically include burns greater than 10% of the total body surface area (TBSA) or significant burns of the face, hands, feet, or perineum.
The highest incidence of burn injury occurs during the first few years of life and between 20 and 59 years of age. The major causes of severe burn injury in younger patients are liquid scalds, and flame burns are more common in adult patients.2 Most burn deaths are caused by flame burns, while liquid scald burns account for the second largest number of deaths.
Between 1971 and 1991, burn deaths decreased by 40% with a concomitant 12% decrease in deaths associated with inhalation injury.3 Since 1991, burn deaths per capita have decreased 25% according to statistics from the Centers for Disease Control and Prevention (www.cdc.gov/ncipc/wisqars). These improvements were in part due to prevention strategies resulting in fewer burns of lesser severity as well as significant advances in treatment techniques particularly in children. In 1949, Bull and Fisher first reported the expected 50% mortality rate for burn sizes in several age groups based upon data from their unit.4 They reported that approximately one-half of children aged 0–14 years with 49% TBSA burns die.4 This dismal statistic has dramatically improved, with the latest reports indicating 50% mortality for 98% TBSA burns in children 14 years and younger.5,6 A healthy child with any size burn might be expected to survive.7 Nevertheless, a 60% TBSA burn has been shown to be an important threshold that, if exceeded, can increase the risk of death.8,9 The same cannot be said, however, for those aged 45 years or older, where improvements have been more modest. This is especially true in patients over 65 years of age, where a 35% burn still kills half of the patients.10
These dramatic improvements in mortality after massive burns are due to better resuscitation, improvements in wound coverage by early excision and grafting, better support of the hypermetabolic response to injury, early nutritional support, more appropriate control of infection, and improved treatment of inhalation injuries. Future breakthroughs in the field are likely to be in the area of faster and better return of function and improved cosmetic outcomes.
Some burn patients benefit from treatment in specialized burn centers. These centers have dedicated resources and the required multidisciplinary approach to maximize outcomes from such devastating injuries.11 The American Burn Association and the American College of Surgeons Committee on Trauma have established guidelines to identify patients who should be transferred to a specialized burn center as follows12:
Partial-thickness burns greater than 10% TBSA.
Burns that involve the face, hands, feet, genitalia, perineum, or major joints.
Third-degree burns in any age group.
Electrical injury, including lightning injury.
Chemical burns.
Inhalation injury.
Burn injury in patients with preexisting medical disorders that could complicate management, prolong recovery, or affect mortality.
Any patient with burns and concomitant trauma in which the burn injury poses the greatest risk of morbidity or mortality.
Burned children in hospitals without qualified personnel or equipment for the care of children.
Burn injury in patients who will require special social, emotional, or rehabilitative intervention.
Burns are classified into six causal categories, three zones of injury, and five depths of injury (Table 48-1). The causes include fire, scald, contact, chemical, electrical, and radiation. Fire burns can be divided into flash and flame burns, while scald burns can be divided into those caused by liquids, grease, or steam. Liquid scald burns can be further divided into spill and immersion scalds.
Burn | Categories | Zones of injury | Burn depth |
---|---|---|---|
Fire | Flash | Zone of coagulation | First degree (superficial) |
Flame | |||
Scald | Liquid | Zone of stasis | Superficial second degree (superficial partial thickness) |
– Spill | |||
– Immersion | |||
Grease | Zone of hyperemia | Deep second degree (deep partial thickness) | |
Steam | Third degree (full thickness) | ||
Contact | Fourth degree (deep organ involvement) | ||
Chemical | |||
Electrical | |||
Radiation |
Flame, scald, and contact burns cause cellular damage primarily by the transfer of energy that induces coagulative necrosis. On the other hand, direct damage to cellular membranes is the cause of injury in chemical and electrical burns.
The skin generally provides a barrier to limit transfer of heat energy to underlying tissues; however, after the source of burn is removed, the response of local tissues can lead to further injury. The necrotic area of a burn is termed the “zone of coagulation.” The area immediately surrounding the necrotic zone has a moderate degree of injury that initially causes a decrease in tissue perfusion. This area is termed the “zone of stasis” and, depending on the environment of the wound, can progress to coagulative necrosis if local blood flow is not maintained. Thromboxane A2, a potent vasoconstrictor, is present in high concentrations in burn wounds, and local application of thromboxane inhibitors has been shown to improve blood flow and may decrease this zone of stasis.13,14 Antioxidants15,16 and inhibition of neutrophil-mediated processes17 may also improve blood flow, preserve this tissue, and affect the depth of injury. Endogenous vasodilators such as calcitonin gene-related peptide and substance P, whose levels are increased in the plasma of burn patients,18 may play a role, also. The last area, the zone of hyperemia, is formed as a result of vasodilation from inflammation surrounding the burn wound. This zone contains clearly viable tissue from which the healing process begins (Fig. 48-1).
The hypermetabolic response and massive release of inflammatory mediators in the wound and in other tissues is seen with a significant burn. This hypermetabolism is associated with alterations in blood serum glucose as well as lipids, and it typically occurs in the ebb phase (first 14 hours) and flow phase (until 5 days post burn).19,20,21 Many mediators have been proposed to explain the changes in permeability after burns, including prostaglandins, catecholamines, histamine, bradykinin, vasoactive amines, leukotrienes, and activated complement.22 Mast cells in the burned skin release histamine in large quantities immediately after injury,23 causing a characteristic response in venules by increasing the space in intercellular junctions.24 The use of antihistamines in the treatment of burn edema, however, has had limited success with the possible exception of H2-receptor antagonists.25 In addition, aggregated platelets release serotonin, which plays a major role in the formation of edema. This agent acts directly to increase pulmonary vascular resistance and indirectly aggravates the vasoconstrictive effects of various vasoactive amines. Serotonin blockade has been shown to improve cardiac index, decrease pulmonary artery pressure, and decrease oxygen consumption after burns.26
As previously noted, another mediator likely to play a major role in changes in vascular permeability and tone is thromboxane A2. Levels of thromboxane increase dramatically in the plasma and wounds of burn patients.27,28 This potent vasoconstrictor leads to platelet aggregation in the wound, contributing to expansion of the zone of stasis. It also causes prominent mesenteric vasoconstriction and decreased blood flow to the gut in animal models with compromised gut mucosal integrity and immune function.29
Patients with major burn injury have the highest metabolic rate of all critically ill or injured patients.30 The metabolic response to a severe burn injury is characterized by a hyperdynamic cardiovascular response, increased energy expenditure, loss of lean body mass and body weight, accelerated breakdown of glycogen and protein, lipolysis, and immune depression.31,32 This response is mediated by increases in circulating levels of catabolic hormones including catecholamines, cortisol, and glucagon.32,33
Pharmacological agents have been used to attenuate catabolism and to stimulate growth after burn injury. To further minimize erosion of lean body mass, administration of anabolic hormones such as growth hormone, insulin, insulin-like growth factor (IGF)/IGF-binding protein-3, oxandrolone, fenofibrate, and catecholamine antagonists such as propranolol has been studied. These agents contribute to maintenance of lean body mass and promote wound healing.34,35,36,37,38,39,40
Major burn injury results in multiple organ dysfunction. Cardiac effects include marked loss of plasma volume, increased peripheral vascular resistance, and decreased cardiac output.41,42,43,44 Pulmonary effects include a decrease in pulmonary static compliance.45 These changes are associated with mild direct cardiac damage.46 Renal blood flow decreases with a fall in glomerular filtration rate, which may result in renal dysfunction. Metabolic changes are characterized by an early depression followed by a marked, sustained increase in resting energy expenditure as well as increased lipolysis, proteolysis, and oxygen consumption. This is driven, in part, by an increase in production of catecholamines, cortisol, and glucagon.47 Increased peripheral lipolysis results in hepatic steatosis, and a fatty liver is caused by breakdown of triglycerol into free fatty acids. Like steatosis, peripheral lipolysis contributes to morbidity and mortality through fatty infiltration of various organs. All burn-related metabolic responses last more than 2 years post burn.21 There is a generalized impairment in host defenses, with depressed production of immunoglobulin, decreased opsonic activity, and depressed bactericidal activity.48 This leads to an increased susceptibility to infections.
The patient should be removed from the source of the burn to stop the burning process, and clothing and jewelry should be immediately removed. The patient should be kept warm by being wrapped in a clean sheet or blanket. The immediate treatment of a burn patient should proceed as with any trauma patient, and any potential life-threatening injuries should be identified and treated.
Assessment of the patient starts with the airway. One hundred percent oxygen is administered, and oxygen saturation is monitored. Stridor, wheezing, tachypnea, and hoarseness indicate impending airway obstruction due to an inhalation injury or edema, and immediate treatment is required. If the patient has labored breathing or signs of obstruction, immediate intubation should be performed with in-line stabilization of the neck if an injury to the cervical spine is suspected.
Arterial blood gas and carboxyhemoglobin (COHb) levels should be obtained when inhalation injury is suspected. Carbon monoxide (CO) has an affinity for hemoglobin (Hb) that is 210–280 times that of oxygen, and COHb can falsely elevate oxygen saturation levels determined colorimetrically. Therefore, use of a pulse oximeter may not be effective, as patients with CO poisoning may show falsely normal oxygen saturation levels. Use of a pulse CO-oximeter enables measurement of absorption at several wavelengths to distinguish oxyhemoglobin from COHb saturation and determines blood oxygen saturation more reliably including the total amount of Hb, COHb, met-Hb, and reduced Hb.49
The treatment for CO inhalation is 100% O2 by endotracheal tube or face mask. This will decrease the half-life of CO from 4–6 hours at room air to 40–80 minutes with 100% O2. In three atmosphere absolute 100% oxygen in a hyperbaric chamber, the half-life decreases further to 15–30 minutes. In addition, full-thickness circumferential burns of the chest can interfere with ventilation. Bilateral expansion of the chest should be observed to document equal air movement. If the patient is on a ventilator, airway pressure and Pco2 should be monitored. Rising airway pressure and Pco2 indicates compromised ventilation, and an escharotomy should be performed to allow better movement of the chest and improve ventilation.
Noninvasive measurement of blood pressure may be difficult in patients with burned extremities, and these individuals may need an arterial line so that their blood pressure can be monitored during transfer or resuscitation. A radial arterial line may not be reliable in patients with upper extremity burns and is difficult to secure. Therefore, the insertion of a femoral arterial line may be more appropriate.
Abdominal injuries and fractures may be present in patients who have been burned, as well. Each patient should be fully assessed for associated injuries that may be more immediately life-threatening. The burn wounds can be addressed after standard evaluation.
As previously noted, burn patients should initially be placed on sterile or clean sheets. Cold water and ice may, in large burns, harm patients by inducing hypothermia and should be avoided. The patient should be kept warm and the wounds clean until assessment by the physicians responsible for definitive care of the burns. A nasogastric tube and bladder catheter are placed to decompress the stomach and to monitor urine output.
After a large burn, there is a systemic capillary leakage that increases with burn size. Capillaries usually regain competence after 18–24 hours if resuscitation has been successful. Waiting for longer times until initiating resuscitation of burn patients results in poorer outcomes, and delays should be minimized.50 The best intravenous (IV) access can be obtained with short large-bore peripheral catheters through unburned skin; however, veins beneath burned skin can be accessed to avoid a delay in obtaining IV access. Central venous lines are required when peripheral IV access is difficult. Intraosseous access is another option until IV access is accomplished. Lactated Ringer’s solution without dextrose is the fluid of choice except in children less than 2 years. Infants should receive some 5% dextrose in intravenous solutions to prevent hypoglycemia because they have limited glycogen stores. This can be accomplished by giving 5% dextrose as maintenance fluid and lactated Ringer’s solution as resuscitation fluid.
The initial rate can be rapidly estimated by multiplying the estimated TBSA burned by the weight in kilograms, which is divided by 4 to get an hourly rate for the first eight hours (ie, a rearrangement of the Parkland formula). Thus, the rate of infusion for an 80-kg man with a 40% TBSA burn would be 40% TBSA × 80 kg /4 = 800 mL/h for the first 8 hours.
Different formulas have been devised to assist the clinician in determining the proper amount of resuscitation fluid. Early work by Baxter and Shires formed the basis for modern fluid resuscitation protocols.51 They showed that edema fluid in burn wounds is isotonic and contains the same amount of protein as plasma and that the greatest loss of fluid is into the interstitial fluid compartment. They used various volumes of intravascular fluid to determine the optimal delivered amount in terms of cardiac output and extracellular volume in a canine burn model. These findings led to a successful clinical trial of the “Parkland formula” in resuscitating burn patients (Table 48-2). From their findings, they also concluded that colloid solutions should not be used in the first 24 hours until capillary permeability returns closer to normal. Others have argued that normal capillary permeability is restored somewhat earlier after a burn (8 hours) and, thus, colloids could be used earlier.52
Moncrief and Pruitt studied the hemodynamic effects of fluid resuscitation in burns, which resulted in the Brooke formula (Table 48-2). They showed that fluid loss in moderate burns results in an obligatory 20% decrease in both extracellular fluid and plasma volume during the first 24 hours after injury. In the second 24 hours, plasma volume returns to normal with the administration of colloid. Cardiac output is low on the first post burn day in spite of resuscitation, but subsequently increases to supernormal levels as the flow phase of hypermetabolism is established.22 Since these studies, it has been found that much of the fluid needs are due to capillary leakage that permits passage of large molecules and water into the interstitial space. Intravascular volume follows the gradient into the burn wound and non-burned tissues. Approximately 50% of fluid resuscitation needs are sequestered in nonburned tissues in 50% TBSA burns.53
Hypertonic saline solutions have theoretic advantages in the resuscitation of burn patients. As these solutions have been shown to decrease net fluid intake,54 decrease edema,55 and increase lymph flow by transferring volume from the intracellular space to the interstitium. During the use of these solutions, care must be taken to avoid serum sodium concentrations greater than 160 mEq/dL.22 Of note, it has been shown that patients with over 20% TBSA burns and randomized to resuscitation with either hypertonic saline or lactated Ringer’s solution do not have significant differences in total volume requirements or in changes in percent weight gain in the days following the burn.56 Other investigators have found an increase in renal failure with hypertonic solutions that has tempered further enthusiasm for their use in resuscitation.57 Some burn centers have successfully used a modified hypertonic solution by adding one ampule of sodium bicarbonate to each liter of lactated Ringer’s solution.58 Further research will need to be conducted to determine the optimal formula to reduce over-resuscitation and maintain adequate cellular function.59
Of interest, it has been shown that resuscitation volumes required in the severely burned patient decrease when high-dose intravenous ascorbic acid is administered during resuscitation. This is associated with decreased weight gain and improved oxygenation.60
Most burn centers use guidelines similar to the Parkland or Brooke formulas, with varying amounts of crystalloid and colloid solutions being administered for the first 24 hours post burn (see Table 48-2). These formulas are guidelines to the amount of fluid necessary to maintain adequate perfusion.
In pediatric burns, the commonly used formulas are modified to account for changes in surface area-to-mass ratios. Compared to adults, children have a larger body surface area relative to their weight and generally have greater fluid needs during resuscitation. The Galveston formula for children based on body surface area uses 5000 mL/m2 TBSA burned for resuscitation + 1500 mL/m2 TBSA for maintenance in the first 24 hours (Table 48-2). This formula accounts for both the resuscitation fluid requirements and maintenance needs of children with burns.
All these formulas calculate the amount of volume given in the first 24 hours, with half being given in the first 8 hours and the other half being given over the next 16 hours.
Both under- and over-resuscitation should be prevented. Under-resuscitation may lead to multiple organ failure caused by decreased perfusion; however, under-resuscitation is uncommon.61 On the other hand, over-resuscitation (so called “fluid creep”59) may result in pulmonary edema, extremity and abdominal compartment syndromes, as well as pericardial effusions which may increase risk of acute respiratory distress syndrome (ARDS).62,63,64,65 Over-resuscitation can be prevented by monitoring urine output, which should be maintained at 0.5 mL/kg/h in adults and 1.0 mL/kg/h in children. Other parameters such as heart rate, blood pressure, mental status, and peripheral perfusion should be monitored, also. The rate of intravenous fluid infusion should be adjusted hourly based on the patient’s response to the particular fluid volume administered.
The depth of burn determines the outcome in terms of survival and scarring. Though technologies such as laser Doppler imaging66,67 with multiple sensors hold promise for quantitatively determining burn depth, it is most accurately assessed by the judgment of experienced physicians. These measurements may give objective evidence of tissue loss and assist the physician in the proper choice of treatment. Determination of depth is critical in the treatment plan, as there are wounds that will heal with only local treatment and those that will require operative intervention for timely healing. Being able to identify patients who will need operative intervention will facilitate care.
Superficial burns (first-degree) are confined to the epidermis. These burns are painful, erythematous, and blanch to the touch with an intact epidermis without blister. Examples include sunburn and a minor scald or flash burn. These burns will heal in 3–5 days, will not result in scarring, and treatment is aimed at comfort with the use of topical soothing salves with or without aloe and nonsteroidal anti-inflammatory drugs or acetaminophen.
Partial-thickness (second-degree) burns are divided into two types (Figs. 48-2 and 48-3), superficial and deep. All partial-thickness burns have some degree of dermal injury, and the division is based on the depth of injury into this level. Superficial partial-thickness burns are erythematous, painful, and wet. They blanch to touch and often form blisters. Blistering may not occur for some hours following injury. Burns thought to be first degree may subsequently be diagnosed as partial-thickness burns by the second day. These wounds will spontaneously reepithelialize in 7–14 days from epidermal structures retained in the rete ridges, hair follicles, and sweat glands. Deep partial-thickness burns extending into the reticular dermis will appear dry, paler than pink, or mottled. They may not blanch to touch, but will remain painful to pinprick. In deeper partial-thickness burns, sensation becomes blunted (less sensitive to pinprick than surrounding normal skin). Capillaries may refill slowly after compression or not at all. These burns will heal in 21–28 days or longer, depending on the depth of burn, by reepithelialization from hair follicles and keratinocytes in sweat glands. Nevertheless, deep partial-thickness burns may require skin grafting to facilitate healing. The longer the wound takes to heal, the worse the scarring will be.
Full-thickness (third-degree) burns are burns that extend through the dermis down to the subcutaneous tissue. They are characterized by a firm leathery eschar that is painless and yellow, grey, white, or cherry red in color (Figs. 48-3 and 48-4). The eschar is insensitive to pinprick but may be sensitive to pressure on palpation. No epidermal or dermal appendages remain, and these wounds must heal by reepithelialization from the wound edges by contraction, which takes a protracted time. Full-thickness burns require excision and skin grafting with autograft skin so that they can heal in a timely fashion.
Fourth-degree burns involve other tissues beneath the skin, such as muscle, tendon, and bone. They have a charred appearance that usually results from high-voltage electrical injury or from prolonged duration of contact with fire or an object such as a hot muffler.
The most commonly used method of determining the burn size in adults is the “rule of nines” (Fig. 48-5). Each upper extremity and the head and neck are 9% of the TBSA. Each lower extremity, the anterior trunk, and posterior trunk are 18% each. Finally, the perineum and genitalia are 1% of the TBSA. Another method of estimating burn size is using the patient’s open hand including the digits, which is approximately 1% TBSA.
The Berkow formula or Lund Browder chart can be helpful in determining burn size in children (Table 48-3). Children have a relatively larger portion of body surface area in the head and neck and a smaller surface area in the lower extremities. Infants have 21% of the TBSA in the head and neck and 14% in each leg, which incrementally approaches adult proportions with increasing age.
Area | 1 y | 1–4 y | 5–9 y | 10–14 y | 15 y |
---|---|---|---|---|---|
Head | 19 | 17 | 13 | 11 | 9 |
Neck | 2 | 2 | 2 | 2 | 2 |
Ant. trunk | 13 | 13 | 13 | 13 | 13 |
Post. trunk | 13 | 13 | 13 | 13 | 13 |
Buttock | 2.5 | 2.5 | 2.5 | 2.5 | 2.5 |
Genitalia | 1 | 1 | 1 | 1 | 1 |
Upper arm | 4 | 4 | 4 | 4 | 4 |
Forearm | 3 | 3 | 3 | 3 | 3 |
Hand | 2.5 | 2.5 | 2.5 | 2.5 | 2.5 |
Thigh | 5.5 | 6.5 | 8 | 8.5 | 9 |
Leg | 5 | 5 | 5.5 | 6 | 6.5 |
Foot | 3.5 | 3.5 | 3.5 | 3.5 | 3.5 |
Overestimation of burn size is common.68,69 Precise estimation of burn size is important, as an inaccurate estimation may lead to an inadequate fluid resuscitation or over-resuscitation.70 In the future, computer-aided systems or smartphones may aid estimation of burn size and improve accuracy.71
With circumferential constricting burns (deep partial- and full-thickness burns) to an extremity, peripheral circulation to the limb can be compromised with edema. Development of generalized edema beneath a non-yielding eschar impedes venous outflow and will have a tourniquet effect on the arterial inflow to the distal beds. This can be recognized by numbness and tingling in the limb and increased pain in the digits. Arterial flow can be assessed by pulse oximetry and determination of Doppler signals in the digital arteries and the palmar and plantar arches in affected extremities. Capillary refill is assessed, also. The release of a burn eschar is performed by making lateral and medial incisions on the affected extremity using the electrocautery. The entire constricting eschar must be incised to relieve the obstruction to blood flow. If the hand is involved, two incisions are made on the dorsal surface and along the medial or lateral sides of the digits, taking care not to damage the neurovascular bundles. These bundles are located slightly to the palmar side of the digit.
If vascular compromise has been prolonged, reperfusion after an escharotomy may cause reactive hyperemia and further edema in the muscle, making continued surveillance of the distal extremities necessary. Increased pressures in the underlying musculofascial compartments may necessitate fasciotomies.
Extremity compartment syndrome can also develop in patients receiving large amounts of resuscitation fluids or in severe burns such as electrical injuries. In such patient, a standard fasciotomy should be performed instead of an escharotomy.
A circumferential burn of the chest with a constricting eschar can cause a similar phenomenon, except the effect is to decrease ventilation by limiting excursion of the chest wall. Any decline in ventilator parameters such as an increase in peak airway pressure and Pco2 should prompt inspection of the chest with appropriate escharotomies. Escharotomies should be made in the lateral chest bilaterally with a connecting incision being introduced across the chest to relieve constriction and allow adequate ventilation.
Inhalation injuries occur in 8% of cutaneous burn injuries and are one of the factors that contributes to mortality in burns by adding another inflammatory focus and impeding normal gas exchange. The presence of such an injury can be used as a significant predictor of outcome in massive burns. Inhalation injuries used to be associated with high mortality, but the last few decades have seen improved survival with early diagnosis, better airway management, gentle ventilation, and pulmonary hygiene.72 Early diagnosis and prevention of complications are necessary to decrease morbidity and mortality related to inhalation injury.
In most inhalation injuries, damage is caused primarily by inhaled toxins. Heat is generally dispersed in the upper airways, whereas cooled smoke particles and released toxins are carried distally into the bronchi and alveoli. The injury is principally chemical in mechanism, and the response is an immediate increase in blood flow in the bronchial arteries with formation of edema and increases in lung lymph flow. The lung lymph in this situation is similar to serum, indicating that permeability at the capillary level is markedly increased. The resulting edema is associated with an increase in lung neutrophils, and it is postulated that these cells may be the primary mediators of pulmonary damage with this injury.73 Neutrophils release proteases and reactive oxygen species that can produce conjugated dienes by lipid peroxidation. High concentrations of these conjugated dienes are present in the lung lymph and pulmonary tissues after inhalation injury, suggesting that increased neutrophils are active in producing cytotoxic materials.37
Another hallmark of inhalation injury is separation of the ciliated epithelial cells from the basement membrane followed by formation of exudate within the airways. The exudate consists of proteins found in the lung lymph and eventually coalesces to form fibrin casts.74 Clinically, these fibrin casts can be difficult to clear with standard airway suction, and bronchoscopy is often required. These casts can also add barotrauma to localized areas of the lung by forming a “ball-valve.” During inspiration, the airway diameter increases, and air flows past the cast into the distal airways. During expiration, the airway diameter decreases, and the cast effectively occludes the airway, preventing the inhaled air from escaping. Increasing volume leads to localized increases in pressure that are associated with numerous complications, including pneumothorax and decreased lung compliance. Therapy aimed at clearing the airway and minimizing complications would likely improve outcomes.
Patients with smoke inhalation often present with a history of exposure to smoke in a closed space, stridor, hoarseness, wheezing, carbonaceous sputum, facial burns, and singed nasal vibrissae. Each of these findings has poor sensitivity and specificity75; therefore, the diagnosis is often established with bronchoscopy. Bronchoscopy can reveal early inflammatory changes such as erythema, edema, ulceration, sloughing of mucosa, and prominent vasculature in addition to infraglottic soot.76 Mechanical ventilation may be needed to maintain gas exchange, and repeated bronchoscopies may reveal continued ulceration of the airways with the formation of granulation tissue and exudate, inspissation of secretions, and edema.
Management of an inhalation injury is directed at maintaining open airways, clearing secretions, and maximizing gas exchange while the lungs heal. A coughing patient with a patent airway can clear secretions very effectively, and efforts should be made to treat patients without mechanical ventilation, if possible. If respiratory failure is imminent, intubation should be instituted early, with frequent chest physiotherapy and suctioning being performed to maintain pulmonary hygiene. Frequent bronchoscopies may be needed to clear inspissated secretions. Mechanical ventilation should be used to provide gas exchange with as little barotrauma as possible. Inhalation treatments have been effective in improving the clearance of tracheobronchial secretions and decreasing bronchospasm.
For the pharmacological treatment of inhalation injury, beta-agonists, nebulized acetylcysteine and heparin, and nebulized racemic epinephrine have been beneficial as has adequate humidification of ventilated air.9,77,78
In addition to conventional ventilator methods, novel ventilator therapies such as high-frequency percussive ventilation have been devised to minimize barotrauma. This method combines standard tidal volumes and respirations (ventilator rates 6–20 breaths/minute) with smaller high-frequency respirations (200–500 breaths/minute) and has been shown to permit adequate ventilation and oxygenation in patients who have failed conventional ventilation. An explanation for the greater utility of this method is the ability to recruit alveoli at lower airway pressures.79 This ventilator method may also have a percussive effect that loosens inspissated secretions and improves pulmonary hygiene.
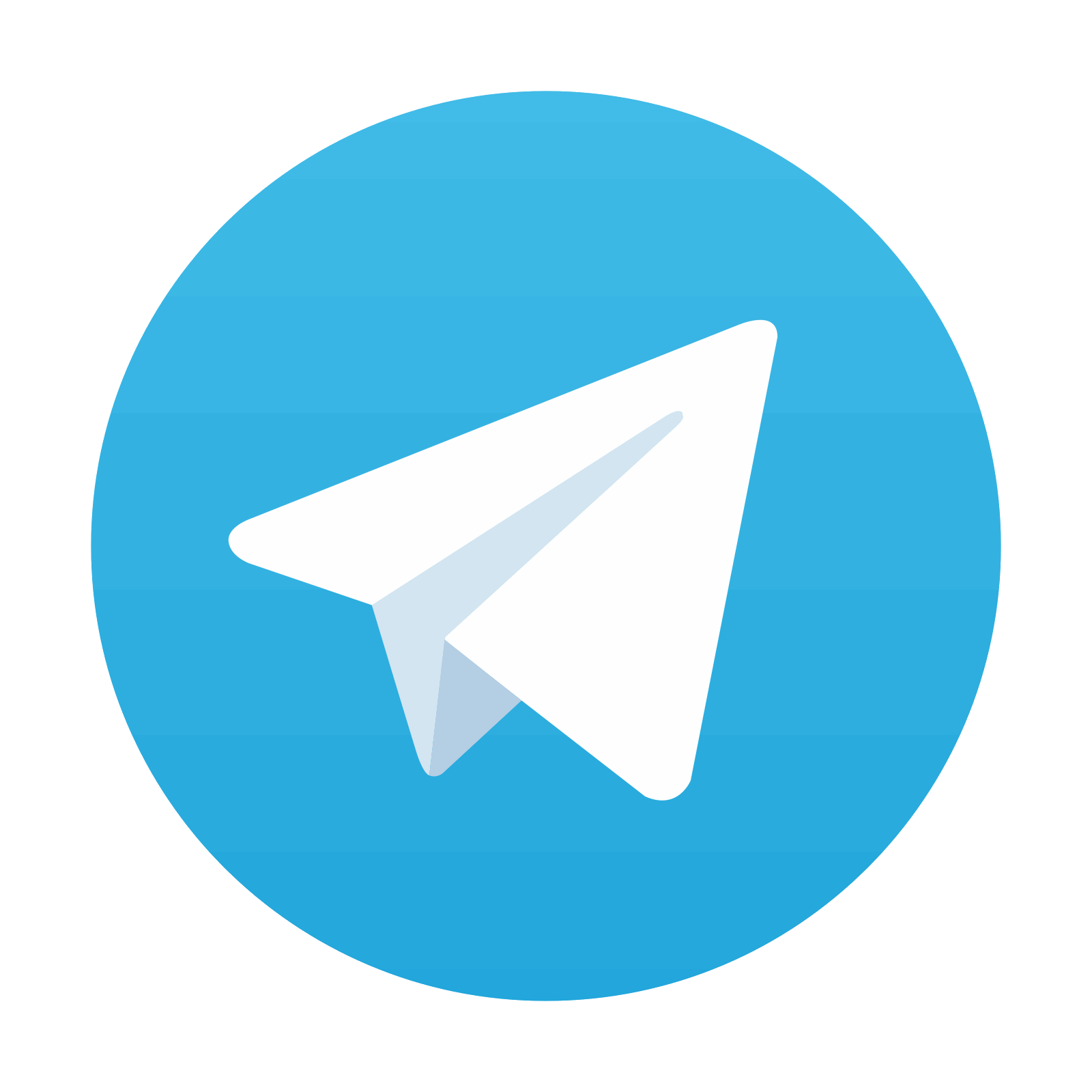
Stay updated, free articles. Join our Telegram channel

Full access? Get Clinical Tree
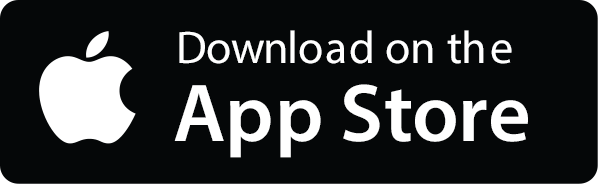
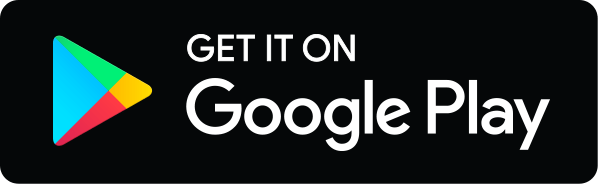