Model
Description
Advantages
Limitations
Methionine-choline-deficient diet (MCD)
•High-sucrose (40 %) and high-fat (10–20 %) diet lacking methionine and choline
•Impaired VLDL assembly and β-oxidation of fatty acids
•Day 10: NASH
•6–10 weeks: perisinusoidal fibrosis
•Highly reproducible
•Lower diet duration, compared to other diets
•Higher fibrosis, inflammation, oxidative stress, and apoptosis than other models
•>30 % body weight loss
•↓ leptin levels and no ↑ adiponectin
•Low glycemia, peripheral insulin sensitivity (though with hepatic insulin resistance)
•No clear hepatocyte ballooning
High-fat diet
•Usually >60 % calories from fat
•Develops obesity, insulin resistance, and dyslipidemia
•Hepatic steatosis
•Effects strain and gender dependent
•Variable liver injury even in the same cohort
•Very mild inflammation
•Fibrosis very mild and only after at least 1 year of treatment
Western/fast-food diet
•↑ % saturated and trans fats
•High cholesterol intake
HFCS equivalents
•Develop obesity, insulin resistance, and dyslipidemia
•NASH with hepatocellular ballooning
•Variable degrees of fibrosis
•Longer duration of diet, usually 16 weeks (12–20 weeks)
•Not all mice develop fibrosis
Atherogenic diet or Paigen diet
•Diet with 1.25 % cholesterol and 0.5 % cholate
•Fibrosing NASH with hepatocellular ballooning
•It needs 6 months of diet (3 months if high fat also)
•Weight loss and ↓ visceral adiposity
•No hypertriglyceridemia or insulin resistance
CLA diet
Supplementation with the trans-fat-conjugated linoleic acid
•Insulin resistance
•Steatosis, apoptosis, and inflammation
•Only with mild perisinusoidal fibrosis
CDAA diet
•Diet choline deficient, l-amino acid defined
•Fibrosing NASH and even cirrhosis in rats, after 3 months
•Hepatocellular carcinoma in 1 year
•Enhanced liver injury if also high-fat diet
•Weight loss
•↑ adiponectin
•Insulin sensitivity
Genetic Models (Table 6.2)
Rodents with Disturbed Appetite Regulation
ob/ob Mice
The ob/ob phenotype resulted from a spontaneous mutation in an outbred colony at Roscoe B. Jackson Memorial Laboratory in 1949 and subsequent transfer of the mutation onto a C57BL/6 background [87]. Forty-five years later, Jeff Friedman discovered that the mutated gene responsible for the phenotype was leptin, an adipokine produced mainly in white adipose tissue [88]. The main signaling pathways of leptin are Janus kinase and signal transducer and activator of transcription (JAK-STAT), mitogen-activated protein kinase (MAPK), phosphoinositide 3-kinase (PI3K), and AMP-activated protein kinase (AMPK) pathways [89]. Leptin receptor has different isoforms generated by alternative splicing of leptin mRNA [90]. The long form of the receptor (Ob-Rb) encoded by the full-length leptin transcript) is necessary for activation of JAK-STAT pathway. Ob-Rb acts both centrally in the nervous system and in peripheral tissues, such as the adipose tissue, muscle, liver, and pancreatic islets. The short form of the leptin receptor (Ob-Ra), which lacks the cytosolic domain of Ob-Rb, is localized predominantly in the skeletal muscle and activates the PI3K pathway [89]. In the hypothalamus, leptin acts in the ventral median nucleus as a potent anorexic agent [6]. Besides regulating satiety, leptin increases energy expenditure, promotes physical activity, enhances thermogenesis, increases sympathetic tone, and regulates immune cells, such as T cells and macrophages, among many other actions. Consequently, ob/ob mice are hyperphagic, inactive, and markedly obese (up to four times the weight of wild-type animals), have all the features of the metabolic syndrome except hypertension, and exhibit impaired immune function [91]. ob/ob mice are indistinguishable from wild-type mice at birth but start to gain weight and become hyperinsulinemic by 2 weeks of age. Hyperglycemia is evident by 4 weeks of age and steadily worsens until 3–5 months of age. After that, glycemia decreases and eventually normalizes with aging [89].
ob/ob mice have deregulated lipid and bile acid metabolism and develop severe hepatic steatosis on regular chow diets [92]. NASH occurs with addition of secondary insults, such as HFD [93–95], or MCD diet [96, 97], or low dose of LPS [98]. However, ob/ob mice are very resistant to fibrosis, even when other triggers for liver injury, such as chronic carbon tetrachloride treatment, are applied [99, 100], revealing the important role of leptin in liver fibrogenesis [91]. Also, the associations between leptin and the sympathetic nervous system seem important, since treating ob/ob mice with norepinephrine shifts the liver immune microenvironment to a more fibrogenic Th2 response and increases recruitment of NKT cells, promoting LPS toxicity and fibrogenesis [101, 102].
In conclusion, although mutations in ob gene are very rare in obese humans with NAFLD [6], functional leptin deficiency caused by hyperleptinemia-induced leptin resistance is common in human obesity. This may explain why the leptin-deficient, ob/ob mouse is a good model for human NAFLD. Like humans with NAFLD, ob/ob mice have metabolic syndrome-associated steatosis and develop NASH when challenged with secondary metabolic/inflammatory stressors. However, ob/ob mice are more resistant to liver fibrosis than most NAFLD/NASH patients and thus, they are not a useful model for studying the pathogenesis of NASH cirrhosis.
db/db Mice
Mice with db/db phenotype were first described in 1996 as a model of type 2 diabetes [103]. They carry a recessive mutation in the leptin receptor gene (Ob-Rb) on mouse chromosome 4 [104]. The db mutation leads to premature termination of Ob-Rb long intracellular signaling domain, resulting in leptin resistance despite normal or increased leptin levels [104]. db/db mice start to gain weight at 3–4 weeks of age and shortly thereafter develop hyperglycemia, polyuria, and glycosuria. As with ob/ob mice, db/db mice develop all the features of the metabolic syndrome except hypertension. Db/db mice were originally described in the C57BLKS/J strain but were subsequently backcrossed onto C57BL/6J and FVB/NJ strains [105]. The phenotype is more severe in the former.
db/db exhibit liver steatosis, without appreciable hepatic inflammation or fibrosis, when fed normal chow. When placed on MCD diets, db/db mice develop worse and earlier liver inflammation and fibrosis than lean nondiabetic mice [11]. Within 4 weeks, collagen-1α gene expression is 2.5-fold greater in MCD diet-fed db/db mice than MCD diet-fed wild-type controls, and both groups have significantly more fibrosis than MCD diet-fed ob/ob mice [96], demonstrating a role of Ob-Ra (short isoform of leptin receptor) in liver fibrogenesis [106]. HFD also significantly worsens liver injury and fibrosis in db/db mice with one third of HFD-fed db/db mice achieving at least 5 points in NAFLD Activity Score after 3 months of HFD exposure [94]. The aggregate data indicate that the db/db mouse is a good model for human NAFLD. Like most NAFLD patients, db/db mice are hyperleptinemic, are leptin resistant, and have liver steatosis and the metabolic syndrome. In addition, like many NAFLD patients, db/db mice develop NASH when further challenged by additional oxidant/metabolic stress. Finally, progressive liver fibrosis results from NASH in db/db mice, as occurs in some patients with NASH.
fa/fa Zucker Rats
The fa/fa Zucker rat obese phenotype was observed by Zucker et al., in 1961, and demonstrated to result from a spontaneous mutation, named fatty or fa [107]. It is an autosomal recessive mutation that affects the extracellular part of the leptin receptor, leading to weaker affinity for leptin and altered signal transduction [90]. The mutation localizes in +269 codon, replacing a glutamine with a proline, leading to a truncated leptin receptor protein [90].
Zucker fa/fa rats exhibit hyperphagia, obesity, and hyperlipidemia, with plasma triglycerides and cholesterol increasing with age. They exhibit mild insulin resistance and hyperinsulinemia but do not have fasting hyperglycemia. Also, moderate hypertension may develop in older mice. fa/fa rats also develop spontaneous liver steatosis, with liver lipogenesis increasing, but adipose tissue lipogenesis decreasing, with age [108]. However, the hepatic steatosis is mild and does not typically lead to NASH or fibrosis. After consuming high-saturated fat diet (60 % calories from fat as lard) for 8 weeks, fa/fa rats developed overt hyperglycemia and liver injury, with increased levels of ALT, oxidative stress, more steatosis, and mild periportal fibrosis (assessed by Sirius red and hydroxyproline assay) [109]. When given long term with or without low doses of LPS, another diet enriched with disaccharides (12.1 % calories as sucrose or lactose) exacerbated steatosis and induced fibrosis, without worsening liver inflammation. Fibrosis severity increased with diet duration, and bridging was apparent after 24 weeks of diet exposure [110, 111]. Thus, fa/fa rats provide another model of potentially progressive NAFLD that develops in the context of the metabolic syndrome.
Agouti Yellow Mice
Agouti was the first cloned gene for obesity [112]. In wild-type mice, Agouti protein is expressed only in the skin during the neonatal period and in the testis during adulthood. Agouti is normally involved in the regulation of coat color, inducing a subapical yellow band on otherwise-black hair [113]. Agouti mice harbor a spontaneous mutation, Ay, that deletes a fragment of DNA 170 kB upstream of the coding region of the Agouti gene. This DNA deletion removes the Raly (ribonucleoprotein associated with lethal yellow) gene coding sequence, leaving the promoter of the Raly gene (which encodes a ubiquitous protein) to control Agouti gene expression. This causes ectopic expression of Agouti in multiple tissues [90]. Homozygous animals for the Ay mutation do not survive. Heterozygous mice are hyperphagic (though less so than ob/ob mice), obese, insulin resistant, hyperglycemic, dyslipidemic, and hyperleptinemic. They also exhibit increased linear growth and yellow coat color [113].
Agouti functions as a competitive inhibitor for melanocortin receptors (MC-R). MC-R are G-protein-coupled receptors expressed in different tissues, for instance, MC1-R is expressed in melanocytes and regulates pigmentation; MC4-R is present in the hypothalamus and regulates appetite. Agouti prevents the binding of α-melanocyte-stimulating hormone (α-MSH) to melanocortin receptors. This suppresses the production of cAMP. In melanocytes, reduced cAMP shifts synthesis of eumelanin (black pigment) to phaeomelanin (yellow pigment). In the hypothalamus, loss of cAMP inhibits appetite suppression [113]. Leptin promotes the expression of α-MSH and, hence, seems to regulate appetite by acting upstream MC4-R [114]. In the hypothalamus, ectopic Agouti prevents a-MSH from activating MC4-R, thereby blocking the normal actions of leptin on MC4-R signaling. Ectopic expression of Agouti in adipose tissue leads to increased leptin expression and secretion. The resultant hyperleptinemia generally reenforces leptin resistance [115]. However, Agouti mice are not resistant to leptin effects on the sympathetic nervous system and they are hypertensive [90]. On chow diets, Agouti mice develop hepatic steatosis. MCD diets provoke worse NASH and result in more severe fibrosis in Agouti mice than in wild-type mice [116].
MC4-R KO Mice
Mice deficient in MC4-R have a similar phenotype as Agouti mouse, without the yellow discoloration of the coat. In the liver, they develop massive hepatic steatosis associated with increased hepatic lipogenesis [117]. When fed a HFD (60 % calories from fat), MC4-R-deficient mice develop progressive disease with NASH (inflammation, hepatocellular ballooning) and pericellular fibrosis at 20 weeks and hepatocellular carcinoma after one year [118].
Fat Aussie (Alms1 foz/foz) Mice
The Alström syndrome is an autosomal recessive disease characterized by childhood-onset obesity, metabolic syndrome, type 2 diabetes mellitus, and infertility. It is caused by mutations in human ALMS1 gene. Fat aussie (Alms1 foz/foz) is a mouse model of Alström syndrome that results from the spontaneous recessive variant in Alms1 gene in a nonobese diabetic (NOD) mouse colony. The variant consists of a deletion causing a frameshift and premature termination codon [119]. The protein encoded by the wild-type Alms1 gene is expressed in the basal bodies of cilia. Although its biological role is not yet understood, Alms1 seems to be involved in appetite regulation. Alms1 foz/foz mice are normal in weight when young but become hyperphagic at 2 months of age and exhibit obesity, hyperinsulinemia, and diabetes by 4 months of age. Male foz/foz mice are always infertile, but females are fertile until the onset of metabolic disturbances [119]. On chow diet, foz/foz mice develop simple steatosis. When fed HFD, they develop NASH after 3 months and pericellular fibrosis after 6 months. HFD liver injury is significantly worse in HFD-fed foz/foz mice than in wild-type mice that were fed the same diet [120, 121]. Increasing the cholesterol content of the diet progressively worsens liver injury in foz/foz mice [122]. The severity of HFD-induced liver injury is also influenced by genetic background, being more severe in foz/foz C57BL/6 mice than foz/foz BALB/c mice [52].
Otsuka Long-Evans Tokushima Fatty Rats
Otsuka Long-Evans Tokushima fatty (OLETF) rats emerged in an outbred colony of Long-Evans rats at the Tokushima Research Institute in 1984 due to a spontaneous loss-of-function mutation in the cholecystokinin 1 (CCK-1) gene [123]. CCK controls food intake. Hence, loss of CCK-1 function results in hyperphagia and obesity. OLETF rats are frankly diabetic by adulthood, manifesting severe hyperglycemia, polyuria, and polydipsia [90].
OLEFT rats fed standard rodent diet develop NAFLD with steatosis and hepatocellular ballooning at 22–38 weeks. Those features, however, disappear at 42 weeks of age, and there is no fibrosis [124]. When OLEFT rats are fed MCD diet, with or without high-fat content, for 8 weeks, they develop NASH with intense lobular inflammation and perivenular/pericellular fibrosis [125, 126].
Genetic Models with Lipodystrophy-Like Phenotype
SREBP-1c Transgenic Mice
There are two nonobese transgenic mouse models that overexpress sterol regulatory element-binding protein (SREBP), an important transcription factor that regulates lipid and glucose metabolism. In PEPCK-nSREBP-1a transgenic mice, the nSREBP-1a transgene (which encodes a nuclear-targeted SREBP-1a) is under the control of the phosphoenolpyruvate carboxykinase promoter, resulting in deregulated hepatic expression of activated SREBP-1a [127]. These mice develop progressive atrophy of white adipose tissue, increased hepatic lipogenesis and steatosis, and increased ALT [128]. However, spontaneous NASH and dyslipidemia do not occur. In the other transgenic strain, the aP2 promoter controls expression of an nSREBP-1c transgene (which encodes a nuclear-targeted form of SREBP-1c). Because aP2 is an adipose tissue-specific promoter, overexpression of SREBP-1c is localized to adipose depots in these mice. Ap2-nSREBP-1c mice exhibit a severe lipoatrophic phenotype, with very low plasma leptin levels [129]. The animals are hyperphagic, insulin resistant, and hypertriglyceridemic. They develop massive ectopic fat accumulation, including hepatic steatosis, which is evident as early as 8 days of age and worsens with age. By 20 weeks, the mice develop NASH with hepatocellular ballooning and pericellular/perivenular fibrosis [130]. Hence, aP2-nSREBP-1c mice are an excellent model for lipodystrophy-associated NASH.
aP2-Diphteria Toxin Mice
aP2-diphteria toxin mice (aP2/DTA mice) are another model of lipodystrophy-induced NAFLD [131]. In these mice, expression of an attenuated diphtheria toxin A chain has been targeted to adipose tissue, resulting in severe atrophy and necrosis of subcutaneous and intra-abdominal adipose tissue by 8–9 months of age [132].
A-ZIP/F1 Mice
This is also a model of severe lipodystrophy. The mice are hyperphagic, hypoleptinemic (20-fold lower levels than wild-type mice), diabetic, and hypertensive. They develop severe hepatic steatosis [133]. Transgenic mice express a dominant negative protein, A-ZIP/F1, under the control of the aP2 promoter. Hence, transgene expression is targeted to fat. The protein encoded by A-ZIP prevents the DNA binding of B-ZIP transcription factors, such as C/EBP and Jun family members, thereby interfering with adipocyte differentiation. Although this lipodystrophy model results in severe hepatic steatosis, it does not appear to cause spontaneous NASH or fibrosis.
CD36-Deficient Mice
CD36 (fatty acid translocase) is a multispecific transmembrane glycoprotein that acts as facilitator of fatty acid cellular uptake [131]. It is expressed in the liver and peripheral tissue, including muscle and adipose tissue. CD36-deficient mice exhibit impairment in fatty acid storage and hence increased circulating levels of long-chain fatty acids and triglycerides, hepatic insulin resistance (though with muscle insulin sensitivity), and hepatic steatosis [134].
Genetic Models with Primary Altered Lipid Metabolism
Acyl-Coenzyme A Oxidase (AOX)-Deficient Mice
AOX KO mice are deficient in Acyl-coenzyme A oxidase, which is the rate-limiting enzyme of peroxisomal β-oxidation of long-chain fatty acids. These mice exhibit growth retardation and high levels of very long-chain fatty acids. They develop hepatic steatosis as soon as 8 days of age. Over time, hepatic lipid accumulation increases in severity and becomes associated with focal inflammatory cell infiltrate. There is also increased hepatocyte apoptosis and hepatocyte proliferation/regeneration. At the age of 2 months, clusters of regenerating hepatocytes with peroxisome-rich eosinophilic granular cytoplasm (indicative of peroxisomal proliferation) accumulate in periportal areas. Thereafter, steatosis progressively decreases. Starting at 8 months, portal-portal and portal-central bridging fibrosis is evident. At 15 months of age, hepatocellular carcinoma develops. Liver disease pathogenesis is attributed to the accumulation of long-chain fatty acids, leading to oxidative stress and sustained activation of peroxisome proliferator-activated receptor (PPAR)-α. The latter induces endoplasmic reticulum stress, which promotes hepatocyte apoptosis and a regenerative response that increases hepatocarcinogenesis [135, 136].
PPAR-α-Deficient Mice
PPAR-α is a transcription factor that regulates peroxisomal, mitochondrial, and microsomal fatty acid oxidation. PPAR-α is expressed in the liver, adipose tissue, muscle, kidney, and pancreas. Its expression decreases in the liver of rodents submitted to high-fat diet [137]. Mice deficient in PPAR-α develop massive hepatic steatosis during HFD feeding or prolonged fasting (24–72 h). In fact, PPAR-α is crucial to the metabolic adaptation to fasting, and PPAR-a-deficient mice develop severe hypoglycemia, hypoketonemia, and hypothermia in that condition [138].
Microsomal Trifunctional Protein-Deficient Mice
Microsomal trifunctional protein (MTP) is a crucial enzyme for mitochondrial β-oxidation of fatty acids. Homozygous KO mice die 6–36 h post birth due to severe hypoglycemia [139]. Heterozygous mice survive the perinatal period but develop progressive, age-related increases in plasma ALT levels, associated with hepatic steatosis, oxidative stress, and mitochondrial degeneration. MPT heterozygous mice do not develop liver necroinflammation or hepatic fibrosis, however [140].
Other Rodent Genetic Models
PTEN Null Mice
Phosphatase and tensin homologue deleted on chromosome 10 (PTEN) encodes a lipid phosphatase, with its main substrate being phosphatidylinositol-3,4,5-triphosphate (PI3P). It acts as a tumor suppressor by inhibiting the PIP3 kinase and serine-threonine protein kinase B (PkB or Akt) pathways [6]. Mice that are global knockouts (KO) for PTEN are not viable, dying early during embryonic development [141]. Liver-specific KO, PTENloxP/loxP, and Alb-Cre+ are viable but develop NAFLD with increased synthesis of fatty acids and glycogen. Contrary to human NAFLD, these mice exhibit liver hypersensitivity to insulin [141]. Liver-specific PTEN KO mice develop progressive liver disease, with macrovesicular steatosis by week 10 of age; NASH with hepatocellular ballooning, Mallory-Denk bodies, and sinusoidal fibrosis by week 40; and hepatocellular carcinoma with a penetrance of 47 % at week 44 and 100 % at weeks 74–78 [35, 142].
Methionine Adenosyltransferase 1A-Deficient Mice
Methionine adenosyltransferase (MAT) is the enzyme that catalyzes the conversion of methionine and ATP in SAMe. The isoform 1A is liver specific [11]. KO mice have impaired antioxidant defense and perturbations of lipid metabolism [143]. Those mice develop spontaneous NASH with fibrosis at 8 months of age. Although they are hyperglycemic, their insulin levels are normal and they do not develop other features of the metabolic syndrome. MAT1A KO mice exhibit a higher susceptibility to development of liver tumors [144].
Cystathionine β-Synthase-Deficient Mice
Cystathionine β-synthase (CBS) deficiency causes severe hyperhomocysteinemia. Homocysteine may be recycled in methionine. Alternatively, it can be converted in cystathionine, which can then be metabolized to cysteine, an important substrate for the synthesis of the antioxidant glutathione [145]. As such, hyperhomocysteinemia induces liver injury by oxidative stress. In addition, the condition induces ER stress and results in enhanced lipid biosynthesis and, hence, liver steatosis [146]. Homozygous KO mice for CBS develop hepatic steatosis and fibrosis at 15 days old on standard rodent diet and usually die before 1 month of age [145]. However, if fed a diet supplemented with choline (1.592 g/kg), the mice survive through adulthood. They develop progressive liver disease, with steatosis, mild inflammation, and fibrosis around vessels by the age of 8 weeks, pericellular fibrosis at 12 weeks, and mild portal fibrosis with worsening fibrosis at 32 weeks [145].
Interleukin (IL)-6 Deficiency
Human NAFLD and HFD-induced NAFLD in rodents associate with increased levels of IL-6, an important inflammatory cytokine [147]. IL-6 also has beneficial actions, however, including induction of compensatory protective mechanisms that guard against energy surplus. IL-6 KO mice develop spontaneous obesity, NASH, and insulin resistance at 4 months of age [148]. When IL-6 KO mice are fed HFD for 12 weeks, they develop worse liver injury, necroinflammation, and insulin resistance than HFD-fed wild-type mice, despite similar weight gain and ectopic fat accumulation [148, 149]. Mice deficient in IL-10, an anti-inflammatory cytokine, develop a huge liver inflammatory response with increase in IL-6 and downstream activation of STAT-3 when fed HFD for 12 weeks. Despite this increased inflammatory response, these mice develop less steatosis and less hepatocellular damage than wild-type controls, suggesting a “disconnect” between IL-6-mediated inflammation and fatty liver damage. This concept is supported by evidence that deletion of IL-6 or STAT-3 restored HFD-induced steatosis and hepatocellular damage in IL-10-deficient mice. Surprisingly, the liver inflammatory response also worsened in the absence of IL-6/STAT-3. In the liver, IL-6-mediated induction of STAT-3 was shown to downregulate lipogenic genes and upregulate genes associated with fatty acid oxidation [149]. A recent study showed that increased production of IL-6 might be a compensatory response to obesity that skews macrophages toward a less-inflammatory (i.e., M2) phenotype, thereby limiting the development of insulin resistance. The authors took advantage of specific KO mice to IL-6 receptor α chain in myeloid cells and submitted them to HFD. Compared to wild-type controls, those mice showed higher insulin resistance in adipose tissue and liver; higher systemic, hepatic, and adipose tissue inflammatory response; and a shift toward M1 phenotype [150].
NEMO-Deficient Mice
The IkB kinase (IKK) is essential for activation of the survival factor nuclear factor kappa B (NFkB), which regulates cellular responses to inflammation. Mice with global KO of the IKK subunit NEMO develop massive hepatocyte apoptosis and die during embryonic development. However, liver-specific conditional KO mice develop spontaneous NASH, with steatosis, inflammation, hepatocellular ballooning, apoptosis, and fibrosis, as young as 8 weeks of age. At 12 months they develop hepatocellular carcinoma with 100 % penetrance [151]. Interestingly, hepatocyte apoptosis seems related to activation of DR5 signaling through bile acid deregulation in Alb-Cre, IKK-deleted mice because treating these mice with nor-ursodeoxycholic acid decreased liver apoptosis, inflammation, and fibrosis [152].
Summary
There are a large number of genetic models of NAFLD/NASH. In general, the ones that associate with obesity and the metabolic syndrome do not develop severe NASH or fibrosis. In order to achieve those phenotypes, a second stimulus is necessary, such as exposure to either MCD diet or HFD. Leptin-deficient ob/ob mice, one of the most used models, remain resistant to fibrosis even when subjected to added dietary challenges, demonstrating the importance of leptin signaling in liver fibrogenesis.
There are some models of spontaneous NASH that progress to significant fibrosis and hepatocellular carcinoma over time. Unfortunately, those models generally do not exhibit the metabolic background characteristic of human NAFLD.
Another disadvantage of genetic animal models is that proving causality mandates difficult breeding strategies, considerable time, and expense (Table 6.2).
Table 6.2
Genetic rodent models of NAFLD/NASH
Model | Description | Advantages | Limitations |
---|---|---|---|
ob/ob | •Spontaneous mutation in leptin •Leptin deficiency leads to disturbed appetite regulation | •Mice are hyperphagic, inactive, obese, with insulin resistance and dyslipidemia •Severe hepatic steatosis •MCD diet leads to features of NASH | •No hypertension •NASH requires a second hit (MCD or HFD) •Resistant to fibrosis, even after a second hit |
db/db | •Mutation in leptin receptor (Ob-Rb) | •Obesity and insulin resistance/diabetes mellitus •Macrovesicular steatosis •Fibrosing NASH after MCD diet | •No hypertension •NASH and fibrosis do not occur spontaneously, needs a second hit |
aP2-nSREBP-1c transgenic | •Overexpression of SREBP-1c in adipose tissue, under regulation of aP2 promoter •Impaired adipose tissue differentiation | •Hyperglycemia and ↓ adiponectin •Day 8: steatosis •20 weeks: NASH; mild fibrosis (in 54 %) •30 weeks: fibrosis in 93 % | •Model of lipodystrophy-associated NASH •Hypoleptinemia |
AOX KO | •Deficiency in acyl-coenzyme A oxidase, rate limiting of peroxisomal β-oxidation | •Day 8: steatosis •2 months: inflammation, apoptosis, regenerating hepatocytes •8 months: fibrosis with bridging •15 months: hepatocellular carcinoma | •Fibrosis only after 8 months of age |
PTEN null mice | •PTENloxP/loxP; Alb-Cre+ •Liver-specific KO for the tumor suppressor PTEN | •10 weeks: steatosis •40 weeks: fibrosing NASH •74 weeks: hepatocellular carcinoma in 100 % | •No obesity or metabolic syndrome •Insulin hypersensitivity •Phenotype acquired at advanced age |
MAT1A KO | •Deficiency in methionine adenosyltransferase leading to impaired antioxidant defense and lipid metabolism | •Spontaneous NASH at 8 months •Higher susceptibility to tumors | •Hyperglycemia but normal insulin •Do not develop metabolic syndrome •Fibrosis not described |
Non-rodent Models of NAFLD/NASH
Opossum Model
Gray short-tailed opossum (Monodelphis domestica), when fed a high-fat (19 % calories from fat), high cholesterol (0.7 %) diet for 24 weeks, may develop severe hypercholesterolemia (40-fold increase in VLDL and LDL) or be resistant to it, being classified as high or low responders, respectively. This diet response associates with a variant in the ABCB4 gene [153]. High responders also develop typical features of fibrosing NASH, with elevation of plasma aminotransferases, steatosis, lobular inflammation, hepatocellular ballooning, and perisinusoidal/periportal fibrosis [154].
Ossabaw Pigs
Mini-pigs residing in the Ossabaw Island exhibit a thrifty genotype, developing obesity, insulin resistance, hypertension, and dyslipidemia when fed a high-caloric/atherogenic diet. Thus, these animals are a good model to study the metabolic syndrome and type 2 diabetes mellitus [155]. When the pigs are fed a modified atherogenic diet, with lower choline content (700 ppm versus 1500 ppm in regular diet), they develop liver injury similar to human NASH, with macro- and microvesicular steatosis, inflammation with foamy Kupffer cells, extensive hepatocellular ballooning, and pericellular/perisinusoidal fibrosis. They also exhibit the usual adipokine pattern observed in human NAFLD, with low levels of serum adiponectin and high levels of leptin and TNF-α [156].
Fish Models
Fish models of NAFLD have several advantages over more widely used small mammal models. Namely, fish are less expensive, have shorter generation times, and are highly fertile. Thus, fish are efficient tools for screening studies. Fish larvae also are optically clear, allowing visualization of internal organs without surgery. Also, fat and specific proteins can be visualized in live fish using fluorescent tags [157]. However, fish liver structure is different from mammals, and its small size makes molecular analysis of tissue more difficult.
Two fish models have been used to study metabolic diseases and NAFLD, zebra fish (Danio rerio) and the ricefish medaka (Oryzias latipes). When fed HFD for 12 weeks, medaka develop hyperlipidemia, hyperglycemia, and liver disease characterized by steatosis, hepatocellular ballooning, apoptosis, and fibrosis [158–160]. A diet-induced obesity (DIO) model in zebra fish overfed with Artemia (a living prey with high amount of fat) has also been used to study hepatic steatosis. This model exhibits overexpression of inflammatory cytokines (IL-6 and IL-1β) and lipolytic transcription factors (SREBP-1 and PPAR-γ) in the liver [161]. Treating zebra fish larvae from day 3 to 5 postfertilization elicits an acute phase response, ER stress, hepatomegaly with steatosis, and hepatic stellate cell proliferation and activation [161].
Mutant fish with disrupted ER function, or deregulated metabolism of methionine, phospholipids, and lipids also manifest hepatic steatosis and variable degrees of ER stress but lack evidence of inflammation/NASH [162–166]. Several other transgenic fish models demonstrated increased hepatic lipogenesis with hepatic steatosis [167–169], and spontaneous degeneration to hepatocellular carcinoma has been reported to occur in one of these [167].
Conclusion
The quest for the ideal animal model for human NAFLD/NASH continues. Nevertheless, a variety of animal models are currently available. Some of these are more ideal for modeling the physiology of liver steatosis in the context of the metabolic syndrome, while others better model hepatic inflammation and fibrosis. Ultimately, model selection should be guided by the purpose of the study.
Rodents are by far the most used animals to model NAFLD, since they provide a good balance between cost, time necessary for breeding and intervention, and amount of tissue samples that can be obtained. Mice are particularly amenable for genetic manipulation, allowing for causality studies. Two strategies have been used to evoke NAFLD/NASH, dietary and genetic. Regarding the former, a diet deficient in methionine and choline is the one that produces more severe liver injury, namely, inflammation and fibrosis, in a very reproducible way and after the shortest duration of diet exposure (i.e., 1–2 months). However, it does not induce the metabolic syndrome, and animals lose considerable weight. High-fat diets better model the metabolic syndrome and can also induce fibrosing NASH if they contain high contents of saturated fat and cholesterol and are supplemented with high-fructose corn syrup equivalents. However, a much longer duration of feeding is required, ideally starting as soon as possible after weaning and continuing for an additional 4–6 months. Another disadvantage of this time-consuming and costly approach is that it generally induces relatively mild liver injury and fibrosis, and there appears to be considerable inter-animal variability with regard to the severity of liver injury, even within the same feeding cohort. Several genetic mouse models exhibit obesity, diabetes mellitus, and NAFLD, the most used ones being ob/ob and db/db mice. However, to induce severe NASH and particularly hepatic fibrosis in these strains, a second dietary “hit” is necessary, such as feeding a methionine- and choline-deficient diet. Even with this added dietary challenge, however, only mild liver fibrosis develops in ob/ob mice. Other genetically abnormal mice manifest spontaneous NASH with fibrosis and even hepatocellular carcinoma. However, similar to MCD diet-fed wild-type mice, these genetic models of “spontaneously” progressive NASH are not naturally obese or insulin resistant. More recently, non-rodent models have been used to study NASH, such as opossum and mini-pigs. However, data on these models is still scarce, and they are more expensive than rodent NAFLD/NASH models. Increasingly, fish are being used to model NAFLD/NASH. Fish provide a very useful model to screen for potential genetic defects associated with disease and for therapeutic approaches, but results still need to be confirmed in mammals.
References
1.
Clark JM, Brancati FL, Diehl AM. The prevalence and etiology of elevated aminotransferase levels in the United States. Am J Gastroenterol. 2003;98:960–7.PubMed
2.
Loomba R, Sanyal AJ. The global NAFLD epidemic. Nat Rev Gastroenterol Hepatol. 2013;10:686–90.PubMed
3.
Vernon G, Baranova A, Younossi ZM. Systematic review: the epidemiology and natural history of non-alcoholic fatty liver disease and non-alcoholic steatohepatitis in adults. Aliment Pharmacol Ther. 2011;34:274–85.PubMed
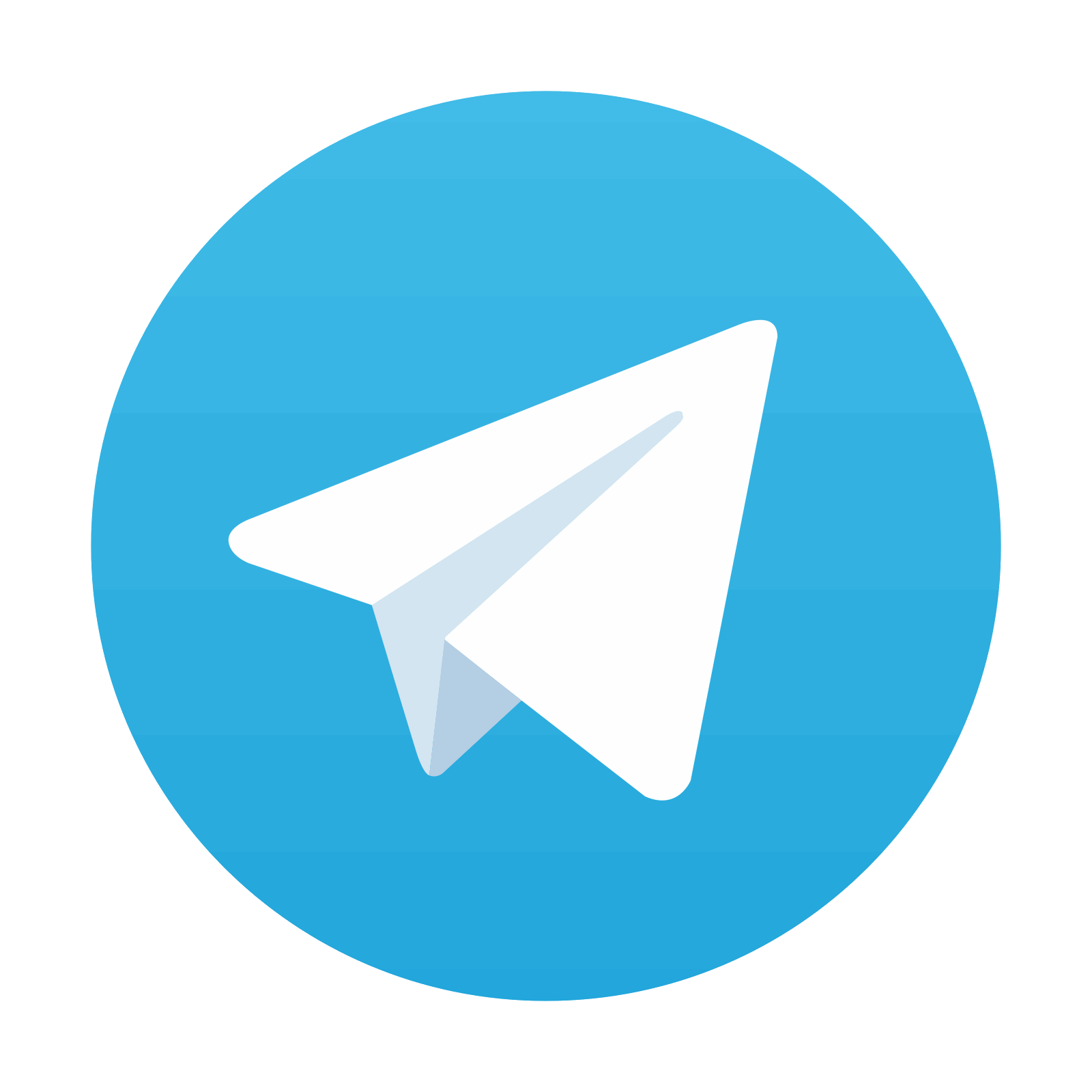
Stay updated, free articles. Join our Telegram channel

Full access? Get Clinical Tree
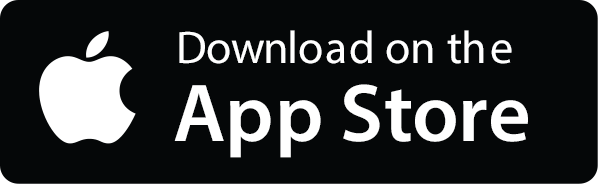
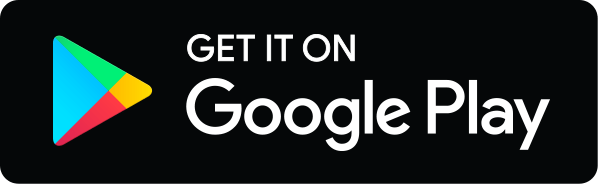