Introduction
The scope of oncology has changed greatly over the past decade as therapies for cancer have moved toward creating or intensifying an immune response against it. The ever-growing number of therapies uses a multitude of different mechanisms, including blockade of specific immune checkpoints, manipulation of T-cells, or direct stimulation of the immune system to enhance killing of tumor cells. Many of the newly available cancer immunotherapies leverage knowledge that the anticancer effects of the immune system (recognizing tumor antigen) are typically balanced by checkpoints that guard against autoimmunity (recognizing self-antigen). These immune checkpoints participate in cancer surveillance and tumor eradication, allowing tumor killing; however, different cancers are able to co-opt these checkpoints to evade surveillance and allow unchecked tumor cell proliferation and metastasis. This immune evasion, which is one of the hallmarks of cancer, leaves the immune system unable to mount an effective antitumor response. The modern immunotherapies work to create robust, antitumor responses by manipulation of the immune system through a variety of mechanisms.
Understanding the basics of the immune system’s role in cancer cell surveillance is critical to recognizing the utility of various immunotherapies. In general, the acquired immune response involves antigen presentation to naïve T-cells, which leads to their subsequent activation and proliferation. T-cell activation requires three signals, which include the T-cell receptor interacting with antigen-bound major histocompatibility complex (MHC) on an antigen-presenting cell (APC). This interaction initiates a second intracellular signaling cascade that is modulated by a second signal from a costimulatory or coinhibitory molecule on the T-cell surface. Lastly, signals produced by cytokines and other molecules further refine the immune response. ,
Enhancing or suppressing T-cell activation via costimulatory or coinhibitory molecules modifies the effector T-cell response and provides a “checkpoint” for immune regulation. Several different receptors on the T-cell surface participate in this process. The constitutively expressed T-cell membrane receptor, CD28, is stimulated and interacts with the CD80 or CD86 receptor on the APC surface when the T-cell receptor engages with MHC/antigen complex. The costimulatory signal promotes recruitment and activation of phosphatidylinositol-3-kinase, which causes intracellular accumulation of 3-phosphorylated lipids that are able to bind and activate pleckstrin-homology-containing proteins. Activation of these intracellular signaling proteins increases cellular metabolism, expression of survival factors, and cell progression through the cell cycle. This T-cell activation cascade can be inhibited at various steps, known as checkpoints ( Fig. 18.1 ). Two receptors that play an important role in regulating T-cell activation and continued function via these intracellular signaling pathways are programmed cell death 1 (PD-1) and cytotoxic lymphocyte-associated antigen 4 (CTLA-4).

PD-1 receptor is a transmembrane protein that is expressed on activated effector and regulatory T-cells, B-cells, and APCs that binds the ligands (PD-L1 and PD-L2), which are expressed on tumor cells, APCs, and other cells. Upon ligand binding, the PD-1 receptor cytoplasmic domain is phosphorylated at two different sites following ligand binding, which recruits two tyrosine phosphatases, , which dephosphorylate signaling intermediates and reduce downstream effects, thereby blunting T-cell activation. CTLA-4 receptor is expressed on the T-cell surface when there is significant T-cell receptor stimulation and competes with CD28 to bind CD80/CD86, thereby reducing the magnitude of the CD28 costimulatory response. , CTLA-4 receptor binding can also inhibit T-cell activation in the absence of CD28 by directly antagonizing T-cell receptor-driven signaling pathways. Ultimately, CTLA-4 receptor activation disrupts effective T-cell signal transmission and T-cell proliferation and inhibits cell cycle progression. , This pathway also suppresses effector T-cells and promotes the development of regulatory T-cells.
Ligand binding to PD-1 and CTLA-4 receptors modifies the immune system response to antigens by inhibiting T-cell activation and allows immunologic self-tolerance. Experimental blockade of PD-1 and CTLA-4 receptors provides insight into the importance of these checkpoints in preventing autoimmunity. PD-1 receptor blockade in both wild type and CD28 deficient mice resulted in an accelerated and severe form of autoimmune encephalomyelitis, marked by increased antigen-specific T-cell expansion and cytokine production. In addition, PD-1 receptor deficient mice had increased B-cell proliferation and reduced expression of CD5 (negative regulator of B-cell activation) compared with control animals. CTLA-4 receptor blockade mice enhanced antitumor immunity, but also elicited autoimmune responses. , Enhanced autoimmunity in a variety of end organs was also noted in six of 14 patients with metastatic melanoma patients, whereas three patients had significant antitumor effects.
Novel immunotherapies include immune checkpoint inhibitors (ICIs), which are monoclonal antibodies aimed at targeting specific immune checkpoints via their ligand-receptor interactions. CTLA-4 is a receptor that, when activated, limits T-cell activation. PD-1 receptor is expressed primarily on the surface of activated T-cells, and is a negative costimulatory receptor. , When PD-1 receptor binds to one of its ligands, such as programmed death ligand 1 (PD-L1) or programmed death ligand 2 (PD-L2), an otherwise cytotoxic T-cell response is inhibited (see Fig. 18.1 ). , As noted, various cancers use this pathway to evade T-cell induced antitumor activity. At present, ICIs available for clinical use include ipilimumab, a CTLA-4 receptor antagonist approved for advanced melanoma, and nivolumab and pembrolizumab, PD-1 receptor antagonists approved for advanced melanoma and non-small cell lung cancer. Other drug trials are underway examining the utility of these monoclonal antibodies for various malignancies.
Another novel modality of harnessing the immune system for its antitumor effects includes chimeric antigen receptor (CAR) T-cell therapy. CAR T-cells are modified using a single-chain variable fragment from an antibody along with a costimulatory domain to target an extracellular antigen. This T-cell manipulation evokes an immune response targeted at specific antigens alone. Clinical trials have focused on targeting CD19, which is expressed ubiquitously on B-cells, thus CD19 targeted CAR T-cells have been used to treat B-cell malignancies. In October 2017, axicabtagene ciloleucel, a CAR T-cell therapy, was approved to treat adult patients with some types of large B-cell lymphoma that have relapsed, or have not responded, to two types of therapy thus far. Given the novelty of the field, the potential targets for CAR T-cell therapy, and the malignancies it may be used in, have not yet been fully discovered.
Interferon alpha (IFN-α) is an older immunologic therapy that has been used in the treatment of chronic myeloid leukemia since 1981. Its use has since been expanded to include metastatic melanoma and hairy cell leukemia (HCL). IFN-α was initially described to have antiviral activity and later realized to have antitumor activity. IFN-α activates the immune system by promoting effector T-cell mediated responses (such as interleukin [IL]-12 secretion) via several signaling events. This robust immune response leads to successful antitumor activity.
IL-2 is a cytokine with both activating and regulatory immune functions. IL-2 mediates the immune system by binding to several different receptors. These receptors are found on activated T-cells, T regulatory cells, memory T-cells, and natural killer cells. Administration of high doses of IL-2 thus leads to a robust immune response, which has been used in the treatment of patients with metastatic renal cell carcinoma since 1991, and with metastatic melanoma since 1998, with improvement in survival.
With the advent of immunotherapy, starting with IFN-α, followed by IL-2 and more recently with ICIs and CAR T-cell therapy, survival for many different cancers has been extended. Most notably, the landscape for metastatic melanoma, non-small cell lung cancer, metastatic renal cell carcinoma, HCL, and B-cell lymphomas has markedly improved. However, these immunotherapies have the potential to result in many different types of nephrotoxicity. In this chapter, we outline each class of immunotherapy, the types of cancer they are approved to treat, and the resultant potential mechanisms of nephrotoxicity.
Immune checkpoint inhibitors
The scope of malignancies that can be treated with ICIs continues to grow, as more clinical trials show promising results. Ipilimumab, the CTLA-4 receptor antagonist, is currently approved for patients with advanced melanoma. Pembrolizumab, a PD-1 receptor antagonist, is currently approved for use in subsets of patients with advanced non-small cell lung cancer, advanced melanoma, advanced urothelial bladder cancer, advanced head and neck squamous cell cancer, classic Hodgkin lymphoma, and microsatellite instability-high tumors. , Nivolumab, another PD-1 receptor antagonist, is approved for a subset of patients with advanced non-small cell lung cancer, advanced melanoma, advanced urothelial bladder cancer, advanced head and neck squamous cell cancer, classic Hodgkin lymphoma, but can also be used in select patients with hepatocellular carcinoma, advanced renal cell cancer, and mismatch repair deficient or microsatellite instability-high metastatic colorectal cancer.
Although these ICIs bring much promise to the treatment of many advanced malignancies, the robust immune response that they generate lends itself to a myriad of different side effects. Almost all organs can be affected by ICI therapy; the most highlighted side effects in the literature are dermatitis, colitis, hypophysitis, pneumonitis, and endocrinopathies. Organs known to be affected by immune-related toxicities include the skin, the endocrine glands, the lungs, the gastrointestinal tract, the central nervous system, the kidneys, the hematologic cells, the musculoskeletal system, the cardiovascular system, and the eyes ( Fig. 18.2 ). The scope and mechanisms of the nephrotoxicities caused by ICI therapy are outlined subsequently.

The best estimate of the incidence of nephrotoxicity cause by ICI therapy comes from Cortazar et al. They analyzed data from phase II and III clinical trials with the goal of estimating the overall incidence of acute kidney injury (AKI), while on ICI therapy. From a total of 3695 patients, they found that the overall incidence of AKI was 2.2%. The incidence of grade III or IV AKI (increase in serum creatinine greater than threefold above baseline, an increase in serum creatinine greater than 4.0 mg/dL, or the need for renal replacement therapy) was 0.6%. When stratified, AKI occurred more frequently with combination therapy with ipilimumab and nivolumab (4.9%), than in patients on monotherapy with ipilumab (2%), nivolumab (1.9%), or pembrolizumab (1.4%). These data suggest that although the incidence of ICI-induced AKI is generally low, the risk appears to be greatly increased with combination therapy. As seen in Table 18.1 , acute interstitial nephritis (AIN) ( Fig. 18.3 ) is by far the most common drug-induced kidney lesion; however, minimal change disease (MCD), immune-complex glomerulonephritis (GN), membranous nephropathy, focal segmental glomerulosclerosis (FSGS), and thrombotic microangiopathy (TMA) have also been described. ,
Reference | Immune Checkpoint Inhibitor Therapy | Onset of AKI After Initiation of ICI | Kidney Presentation | Urinalysis/Urine Sediment | Kidney Biopsy | Treatment | Outcome |
---|---|---|---|---|---|---|---|
Cortazar et al. | |||||||
Ipilimumab | 54 days | AKI | 5–10 WBCS, 2 RBCS | AIN with granuloma | Drug DC, prednisone | PR | |
Ipilimumab + Nivolumab | 91 days | AKI | 2–3 WBCS, 3–5 RBCS | AIN with granuloma | Drug DC, prednisone | CR | |
Ipilimumab + Nivolumab | 69 days | AKI | 5–10 WBCS, 0 RBCS | AIN with granuloma | Drug DC, prednisone | PR | |
Ipilimumab | 70 days | AKI | 0–2 WBC casts, 16–34 WBCS | AIN | Drug DC only | NR | |
Ipilimumab + Nivolumab | 245 days | AKI | 5 WBCS, 1 RBC | AIN | Drug DC, MP, then prednisone | PR | |
Ipilimumab | 183 days | AKI | 0 WBC, 0 RBC | AIN | Drug DC, MP, then prednisone | NR | |
Nivolumab | 224 days | AKI | 0 WBC, 0 RBC | AIN | Drug DC, prednisone | PR | |
Ipilimumab | 154 days | AKI | 6–9 WBCs, 0–3 RBCs | TMA | Drug DC, prednisone | NR | |
Ipilimumab + Nivolumab | 42 days | AKI | 9 WBCs, 8 RBCs | AIN | Drug DC, MP, prednisone, MMF | PR | |
Ipilimumab | 120 days | AKI | 3 WBCs/RBCs, WBC casts | AIN and IC deposits | Drug continued | NR | |
Ipilimumab | 60 days | AKI | 50–100 WBCs, 0–2 RBCs | AIN | Drug DC, prednisone | PR | |
Pembrolizumab | 21 days | AKI | 20–50 WBCs, 0–2 RBCs | AIN | Drug DC, MP, then prednisone | PR | |
Pembrolizumab | 231 days | AKI | 11–20 WBCs, 0 RBCs | AIN | Drug DC, MP, then prednisone | CR | |
Shirali et al. | |||||||
Nivolumab | 11 months | AKI | Bland | AIN | Drug continued initially then held | CR 6 months after drug DC | |
Nivolumab | 16 months | AKI | 2 WBC casts/HPF | AIN | Drug DC, prednisone | CR | |
Nivolumab + bevacizumab | 10 months | AKI | 2–5 WBCs/LPF | AIN | Drug continued, MP, then prednisone | CR | |
Pembrolizumab | 3 months | AKI | Numerous WBCs/HPF | AIN | Drug DC, prednisone x 2 courses | CR after 2nd steroid course | |
Nivolumab+ Ipilimumab | 8 months | AKI | 1 WBC cast/LPF | AIN | Drug DC, recurrent AKI with retrial treated with MP and prednisone | CR, recurrent AKI with retrial, PR after steroids | |
Pembrolizumab | 365 days | AKI | 15–30 WBCs/HPF | AIN | Drug DC, prednisone | CR | |
Izzedine et al. | |||||||
Ipilimumab | 42 days | AKI | 35 WBCs/HPF, 70 RBCs/HPF | AIN | Drug DC, prednisone | CR | |
Ipilimumab | 42 days | AKI | N/A | AIN with granuloma | Drug DC, prednisone | CR | |
Belliere et al. | |||||||
Nivolumab | 48 days | AKI | Bland | AIN with granuloma | Drug DC, steroids | PR | |
Pembrolizumab | 137 days | AKI | Bland | AIN | Drug DC, steroids | PR | |
Ipilimumab | 130 days | AKI | Bland | AIN | Drug DC, steroids | CR | |
Murakami et al. | |||||||
Nivolumab + Ipilimumab | 21 days | AKI | 9 WBCs/HPF, 8 RBCs/HPF, WBC and granular casts | AIN | Drug DC, MP and prednisone, MMF after failure | NR, fatal septic shock | |
Kitchlu et al. | |||||||
Pembrolizumab | 1 month | NS, AKI | N/A | MCD, mild ATI | Drug DC, prednisone, dexamethasone | PR | |
Ipilimumab | 18 months | NS | N/A | MCD | Drug DC, prednisone | CR, relapse of NS with retrial, CR with DC | |
Fadel et al. | |||||||
Ipilimumab | 21 days | NS | 25 RBCs/cubic mm | Lupus nephritis | Drug DC, prednisone | CR | |
Jung et al. | |||||||
Nivolumab | 10 months | AKI | Numerous RBCs, 3–5 WBCs, 1–3 granular casts | IgA dominant GN | Drug DC, MP followed by prednisone | HD dependent for 6 months, CR off HD | |
Daanen et al. | |||||||
Nivolumab | 1.5 months | NS, AKI | Many hyaline casts, few WBCs/RBCs | FSGS | Drug DC, MP, then prednisone | PR | |
Lin et al. | |||||||
Nivolumab | 2 months | NS | Bland | Membranous nephropathy | Drug DC, prednisone | PR | |
Kidd et al. | |||||||
Ipilimumab | N/A | NS, AKI | N/A | MCD | Drug DC, prednisone | CR (NS), PR (AKI) | |
Ray et al. | |||||||
Nivolumab | 14 days | AKI | N/A | Collapsing FSGS | Drug DC, steroids | NR, HD dependent |

Acute interstitial nephritis
Cortazar et al. described 13 patients, from seven academic medical centers, with AKI occurring in the setting of ICI use, all of whom underwent kidney biopsy. Of these patients, six were on ipilimumab monotherapy, one on nivolumab monotherapy, one on pembrolizumab monotherapy, and four were on combination therapy with ipilimumab and nivolumab. Seven of these patients had a documented immune related adverse event (IRAE) before AKI and one patient had a concurrent IRAE with AKI. The most common extrarenal IRAEs were colitis and hypophysitis. The timing of AKI from initiation of ICI therapy ranged from 21 to 245 days, and the timing of AKI compared with the last dose of ICI ranged from 7 to 63 days. Of these patients, eight had pyuria, three had hematuria, and one had eosinophilia. Mean peak serum creatinine was 4.5 mg/dL and the majority of patients had subnephrotic proteinuria. AIN was found in 12 of the 13 patients; one patient had acute TMA. AIN was predominantly lymphocytic with some plasma cells and eosinophils whereas three out of the 12 AIN cases had granulomatous features. One patient with AIN also had evidence of subepithelial and intramembranous deposits; however, an antinuclear antibody titer was negative, the patient had no evidence of infection, and ultimately no explanation was found for these deposits.
ICI therapy was discontinued in 12 of 13 patients because of AKI and other IRAEs. Of the patients with AIN, 10 out of the 12 received treatment with glucocorticoids as did the patient with TMA. Glucocorticoid therapy in AIN patients on glucocorticoids was associated with partial recovery of kidney function in seven and complete recovery in two. The patient with TMA did not recover kidney function and was dialysis dependent. Two patients with AIN who were not treated with glucocorticoids did not recover kidney function, and one became dialysis dependent. Two patients with AIN required transient hemodialysis before recovering. Two patients who recovered after glucocorticoid treatment were rechallenged with ICI therapy and neither developed AKI.
Shirali et al. reported six cases of AKI caused by biopsy-proven AIN in patients with non-small cell lung cancer following treatment with the PD-1 inhibitors, nivolumab and pembrolizumab. In one of the cases, nivolumab was combined with ipilimumab. AKI developed after 3 to 16 months of drug exposure with a median time of 10.5 months. Four of the patients were on other medications, five on proton-pump inhibitors for greater than 3 months and one on ibuprofen for greater than 12 months. In these patients, serum creatinine ranged from 1.8 to 10.6 mg/dL with a mean serum creatinine of 4.1 mg/dL. None of the patients had clinical evidence of an allergic drug reaction except for two patients with a mild serum eosinophilia (6% and 7 %, respectively). Urine studies were suggestive of an inflammatory kidney lesion in four of six patients who had sterile pyuria ( n = 3) or white blood cell casts ( n = 2). One patient had completely bland urine sediment. Following discontinuation of the ICIs (and other culprit drugs) and corticosteroid therapy in all but one patient, five patients had complete recovery of kidney function. One patient had partial recovery with serum creatinine remaining slightly above baseline. No patient required transient or permanent dialysis.
In 2014 Izzedine and colleagues reported the association of the anti–CTLA-4 antibody ipilimumab with two cases of granulomatous AIN. In addition to these two cases, literature review revealed several cases of AIN (clinical and biopsy diagnosis) and one case of an immune-complex GN from this CTLA-4 antagonist. Murakami et al. also described biopsy-proven AIN following exposure ICI therapy. A 75-year-old male treated with combination therapy of ipilimumab and nivolumab developed AKI after his second cycle of treatment. Kidney biopsy revealed diffuse extensive interstitial inflammation without granuloma. Treatment with glucocorticoids was initially associated with improvement in kidney function; however, a week later AKI redeveloped. The patient was treated again with pulse dose steroids and mycophenolate mofetil. Unfortunately, the patient suffered from septic shock and died. This case contrasts the other reported cases, where glucocorticoid treatment resulted in some kidney recovery.
Review of the aforementioned publications highlights the clear link between the ICIs and AIN presenting clinically as AKI. In addition, there appears to be a lack of consistent concomitant allergic symptoms and signs in patients developing ICI-mediated AIN. In reviewing the 13 cases compiled by Cortazar et al., seven patients had developed an IRAE before occurrence of AKI. Only two of Shirali’s six cases had mild eosinophilia at the time of AKI. Urine studies were suggestive of an inflammatory kidney lesion (sterile pyuria, white blood cell [WBC] casts) in some but not all patients with biopsy-proven AIN, making laboratory studies unreliable. In our opinion, a kidney biopsy should be pursued for most patients who develop AKI in the setting of ICI therapy, as our personal experience supports that a significant number of patients with AKI in this setting have another kidney lesion (acute tubular injury [ATI]/acute tubular necrosis [ATN]). As such, a reasonable diagnostic clinical approach is the following: clinicians must be watchful for AKI when patients are on ICI therapy, particularly once an initial IRAE occurs. There is no expected timeline for when AKI develops, which contrasts IRAEs affecting other organs that have more expected time courses. For example, dermatologic side effects typically occur within the first few weeks of ICI initiation, gastrointestinal IRAEs tend to occur within 5 to 10 weeks of initiation, and endocrinopathies and hepatic side effects occur after approximately 6 weeks. In addition, the timeline for ICI-mediated AIN is more variable than seen with traditional drug-induced AIN. ICI-mediated AIN has the potential to develop long after the first and last dose of drug. Laboratory findings in the setting of ICI-induced AIN are generally not helpful, with classic allergic findings, such as rash, fever, and eosinophilia, not present. Sterile pyuria and WBC casts seen on urine sediment examination may provide a clue to the presence of drug-induced AIN, which is largely insensitive and quite often nonspecific (seen in other kidney lesions).
Collectively, the data suggest that complete or partial recovery of kidney function may be achieved with some combination of drug discontinuation and glucocorticoid treatment. However, a small number may require long-term dialysis. Although initial drug discontinuation is prudent in the setting of AKI (and biopsy-proven AIN), the feasibility of restarting ICI versus permanent discontinuation is unknown. At present, experts recommend permanent cessation of ICI therapy if a patient develops grade III or IV nephrotoxicity, which is defined as tripling of serum creatinine concentration or need for renal replacement therapy. However, patients who improved on glucocorticoid therapy and were retrialed on ICI therapy did not develop recurrent AKI/AIN. However, one patient developed a recurrence of AKI, when rechallenged with ICI therapy. Thus ICI rechallenge may be feasible in some but not all patients—close monitoring is warranted. With regards to the role of glucocorticoid treatment in ICI-mediated AIN, nine out of 10 patients with AIN (Cortazar’s study) who received glucocorticoids had some degree of kidney recovery as compared with the two untreated patient who did not recover. , In the report by Shirali and coworkers, five of the six patients received glucocorticoid therapy and recovered kidney function to or near baseline, supporting use of this treatment in patients with biopsy-proven AIN. Although these represent case reports, the literature thus far leads the clinician to believe that there is a clearer role for glucocorticoid use in ICI-mediated AIN.
The mechanism underlying kidney injury with ICI therapy has not been clearly worked out. It is possible that ICI-induced AIN may be the result of reprogramming of the host’s immune system, which subsequently leads to loss of tolerance against endogenous kidney antigens not normally targeted. This contrasts the delayed-type hypersensitivity reaction typically seen in AIN from other medications. This mechanism may ultimately explain the long latency period from drug exposure to kidney injury that has been observed in the cases previously reported. In addition, it is possible that ICI therapy with activation of T-cells lowers normal host tolerance to concomitantly prescribed medications linked to AIN (nonsteroidal antiinflammatory drugs [NSAIDs] and proton-pump inhibitors), thereby precipitating drug-induced AIN.
Other kidney lesions
Although AIN is by far the most common nephrotoxicity of ICI therapy, immune-complex GN has also been reported. Fadel et al. described a patient with metastatic melanoma who received ipilimumab intravenously every 3 weeks. After two rounds of treatment, the patient was found to have a serum creatinine of 1.01 mg/dL with a urinary protein excretion rate of 7.5 g/day. Antinuclear antibody was mildly positive at 1:100 and antidouble stranded deoxyribonucleic acid (DNA) antibodies were positive. On kidney biopsy, a mildly proliferative glomerular lesion with thick capillary loops was seen on light microscopy with extramembranous and mesangial desposits of immunoglobulin (Ig)G, IgM, C3, and C1q on immunofluorescence. Granular, electron-dense extramembranous deposits were seen on electron microscopy. The findings were indicative of lupus nephritis and ipilimumab was discontinued. With cessation of ICI therapy, the antidouble stranded DNA antibodies became negative; however, 6 months later the patient continued to have nephrotic range proteinuria and developed a venous thrombotic event. He was subsequently started on prednisone therapy and his proteinuria improved to nonnephrotic range at 12 months postdiagnosis. Given the normalization of the antidouble stranded DNA with withdrawal of the ICI therapy, ipilimumab was thought to be the potential cause of this lupus nephritis-like glomerulopathy.
Jung et al. described a patient with metastatic renal cell cancer on nivolumab treatment, every 2 weeks, who developed an AKI 10 months into treatment. Serum creatinine rose to 10.08 mg/dL from a value of 1.67 mg/dL the month prior. Antinuclear antibody, antidouble stranded DNA antibody, and serum C3 and C4 were normal. Kidney biopsy revealed diffuse tubular injury and a lymphocytic interstitial infiltrate along with an immune complex-mediated GN with cellular crescents and necrosis. Immunofluorescence microscopy revealed diffuse granular mesangial staining for IgA, C3, and kappa and lambda light chains. Electron microscopy revealed hump-like subepithelial deposits with no subendothelial deposits. The final diagnosis was ATI with acute postinfectious, IgA-dominant GN. As this patient had no signs of infection, had a normal C3, and had a lymphocytic infiltrate on biopsy, the AKI was felt to be caused by the ICI. Nivolumab was discontinued and glucocorticoids were initiated. The patient transiently needed hemodialysis, but after 6 months of glucocorticoid therapy, kidney function recovered.
MCD has also been reported in the setting of ICI therapy. In a letter to the editor, Kidd et al. described the first case of MCD associated with ICI therapy. They report on a 55-year-old man with metastatic melanoma treated with ipilimumab who presented with 2 weeks of lower extremity edema, found to have AKI with serum creatinine 2.97 mg/dL (baseline 1.2 mg/dL) with nephrotic range proteinuria (9 g per day) and a serum albumin of 2.2 g/dL. Kidney biopsy revealed an eosinophilic interstitial infiltrate and edema and unremarkable glomeruli on light microscopy. However, electron microscopy revealed marked foot process effacement consistent with MCD. Treatment with steroids improved kidney function and proteinuria to baseline.
Kitchlu et al. also reported two patients who developed nephrotic syndrome secondary to MCD in the setting of ICI therapy. A 43-year-old man with Hodgkin lymphoma treated with pembrolizumab presented with AKI and edema, and was found to have nephrotic range proteinuria of 10.3 g/day. Kidney biopsy revealed focal tubular injury with positive linear basement staining for IgG but negative for IgM, IgA, C3, and C1q. On electron microscopy, marked foot process effacement was seen without deposits, consistent with MCD. Pembrolizumab was discontinued and treatment with a prednisone and taper, followed by dexamethasone and more prednisone, resulted in partial kidney improvement. A 45-year-old man with metastatic melanoma treated with ipilimumab developed nephrotic syndrome 18 months into treatment. Kidney function remained normal; however, hypoalbuminemia (albumin 2.6 g/dL) and proteinuria quantified at 9.5 g/day were noted. Kidney biopsy revealed mesangial staining for IgM on immunofluorescence and diffuse foot process effacement, consistent with MCD. Ipilimumab was discontinued, and prednisone was initiated, with improvement in nephrotic syndrome. Two years later he was retrialed on ipilimumab, however, and developed recurrence of nephrotic syndrome. Like the majority of patients with ICI-mediated AIN, MCD caused by ICI therapy appears to be responsive to glucocorticoid therapy.
FSGS has also been described in the setting of ICI use. Ghosh et al. described a 42-year-old man receiving nivolumab for lung cancer who developed an AKI, 2 weeks after the first dose. Nephrotic range proteinuria with 30.8 g/day developed and high-dose intravenous (IV) steroids were administered. Kidney function continued to worsen despite this regimen and biopsy revealed collapsing FSGS involving 20 out of 46 glomeruli. Hemodialysis was initiated for advanced kidney failure and, despite cessation of nivolumab therapy and steroid taper, the patient remained dialysis dependent. Daanen et al. described a 62-year-old man with renal cell carcinoma treated with nivolumab who developed AKI and generalized edema with nephrotic range proteinuria (17 g/day) after four cycles of treatment. Corticosteroid therapy was initiated and kidney biopsy was ultimately obtained for worsened kidney function despite this treatment. Biopsy revealed FSGS. Nivolumab was discontinued and pulse methylprednisolone was given followed by oral prednisone. Mycophenolate mofetil (MMF) was added because of the poor kidney response to glucocorticoids; partial improvement was achieved, although nephrotic syndrome recurred with steroid tapering 5 months later.
Lin et al. reported on a 75-year-old male with metastatic melanoma treated with nivolumab who presented with signs of nephrotic syndrome after his fifth treatment. Laboratory tests revealed the following: albumin of 1.4 mg/dL, normal serum creatinine, and nephrotic proteinuria quantified at 12 g/day. Nivolumab was discontinued and prednisone was started empirically. Kidney biopsy revealed granular IgG capillary wall staining on immunofluorescence and subepithelial dense deposits and podocyte effacement on electron microscopy consistent with membranous nephropathy. After drug discontinuation and steroid treatment, the patient achieved partial recovery of nephrotic syndrome.
Although ICI therapy is a relatively uncommon cause of AKI, its prevalence will likely increase as ICI therapy continues to expand. Because patients who develop AKI are generally asymptomatic, routine monitoring of kidney function with intermittent serum creatinine measurements and urinalysis is essential. It is prudent to make a definitive diagnosis of the cause of AKI and/or proteinuria/nephrotic syndrome in patients receiving ICIs before administering glucocorticoids as not all lesions (ATI/ATN, etc.) respond to steroids. Once other causes of kidney disease have been excluded, current oncology-driven recommendations for the management of ICI-mediated nephrotoxicity are based on the gradation of the AKI ( Table 18.2 ). , For grade 1 kidney injury, which is defined as serum creatinine 1 to 1.5 times baseline or greater than 1 to 1.5 times the upper limit of normal, 1+ proteinuria on urinalysis, or urinary protein less than 1.0 g/day, the recommendation is to monitor the serum creatinine weekly and continue immunotherapy. If the serum creatinine worsens and the patient progresses to a higher grade (2, 3, or 4), the recommendations change.
Grade of Kidney Injury | Definition (Serum Creatinine) | Definition (Proteinuria) | Treatment Recommendations |
---|---|---|---|
Grade 1 |
|
|
|
Grade 2 |
|
|
|
Grade 3 |
|
|
|
Grade 4 |
|
|
Grade 2 kidney injury is defined as serum creatinine 1.5 to 3.0 times baseline or greater than 1.5 to 3.0 times the upper limit of normal, 2+ proteinuria on urinalysis, or urinary protein quantified between 1.0 to 3.4 g/day. Grade 3 kidney injury is defined as serum creatinine greater than 3.0 times baseline or greater than 3.0 to 6.0 times the upper limit of normal, or urinary protein quantified greater than 3.5 g/day. For both grade 2 and 3 kidney injury, the recommendation is to monitor serum creatinine at least every 2 to 3 days and to withhold ICI therapy. Therapy can be resumed if kidney injury and associated symptoms are mild, resolve, or return to baseline. In addition, it is recommended to administer IV methylprednisolone 0.5 to 1.0 mg/kg/day or equivalent until there is improvement of kidney function to mild severity. Glucocorticoid taper over the course of a month is additionally recommended. Lastly, grade 4 kidney injury is defined by a serum creatinine 6.0 times the upper limit of normal. Recommendations are to monitor the serum creatinine daily, and consider nephrology consultation with potential for kidney biopsy. ICI therapy should be permanently discontinued and IV methylprednisolone should be administered at 1 to 2 mg/kg/day or equivalent, until there is improvement to mild severity. Glucocorticoids should be tapered over a month at the minimum. , ,
From a nephrology perspective, we recommend a slightly different approach to patients developing AKI and proteinuria in the setting of ICI therapy. Patient who develop the Kidney Disease: Improving Global Outcomes (KDIGO) stage 1 AKI can be monitored and evaluated for reversible causes, such as volume depletion and NSAID or contrast-related kidney injury. Those who progress to a higher AKI stage, or present with stage 2 or 3 AKI, should have a nephrology consultation to evaluate for the cause of kidney injury and the possible role of kidney biopsy. The same applies for persistent proteinuria that develops following ICI therapy, especially if proteinuria exceeds 1 g/day. General workup should include complete blood count, chemistry, kidney ultrasonography (exclude obstruction), urinalysis, and urine microscopy. Proteinuria noted on urinalysis should be quantified with a spot urine protein:creatinine ratio or 24-hour urinary collection. Urine microscopy revealing sterile pyuria and WBC casts are suggestive of an inflammatory kidney lesion (AIN or GN) and should prompt a kidney biopsy. Because serum and urine tests lack both sensitivity and specificity for AIN, stage 2 or 3 AKI without an obvious cause would benefit from kidney biopsy to define the underlying lesion. This information will help guide therapy, as ICI-induced AIN is generally glucocorticoid responsive, whereas other AKI lesions (ATI/ATN, TMA, FSGS, etc.) are not. This will limit unnecessary and potentially harmful steroid exposure in patients with cancer (and may allow ongoing ICI therapy). In addition to glucocorticoid therapy, patients with biopsy-proven AIN should have the ICI discontinued in most cases (KDIGO stage 2 and 3 AKI or Oncology grade 2–4 toxicity).
Reinitiation of ICI therapy in patients who have developed AKI is an important and currently unresolved issue. In general, it is considered reasonable to reinitiate ICI therapy if AKI is grade 2 or less and the patient has responded well to treatment with glucocorticoids. Grade 3 to 4 AKI following ICI exposure requires a discussion with the oncology team regarding the risks and benefits of restarting therapy. Of note, two patients reported by Cortazar et al. who developed grade 3 to 4 AKI were rechallenged on these drugs after AKI improved following glucocorticoid therapy. Neither one developed recurrent AKI. A third patient who developed AKI from ICI therapy and was treated conservatively with drug cessation, and no glucocorticoid therapy, did not have any worsening in his kidney function upon drug reexposure. These findings exemplify the importance of having individualized discussions with the patient, nephrologist, and oncologist regarding the risks and benefits of ICI reinitiation.
Given the increasing use of ICI therapy for various cancers, and the potential for nephrotoxicity, nephrologists need to be aware of the different mechanisms of injury. In addition, it is important to be familiar with guidelines for drug discontinuation, glucocorticoid treatment, and reinitiation of ICI therapy. By far the most common pathology of ICI-mediated AKI is AIN, whereas proteinuria (+/- AKI) may occur because of drug-induced membranous nephropathy, immune complex GN, TMA, MCD, and FSGS. We believe it is critical to obtain a kidney biopsy in patients suspected of having ICI-induced AKI or proteinuria to facilitate our understanding of the mechanism of injury, frequency of lesion types, and the likelihood of response to glucocorticoid therapy.
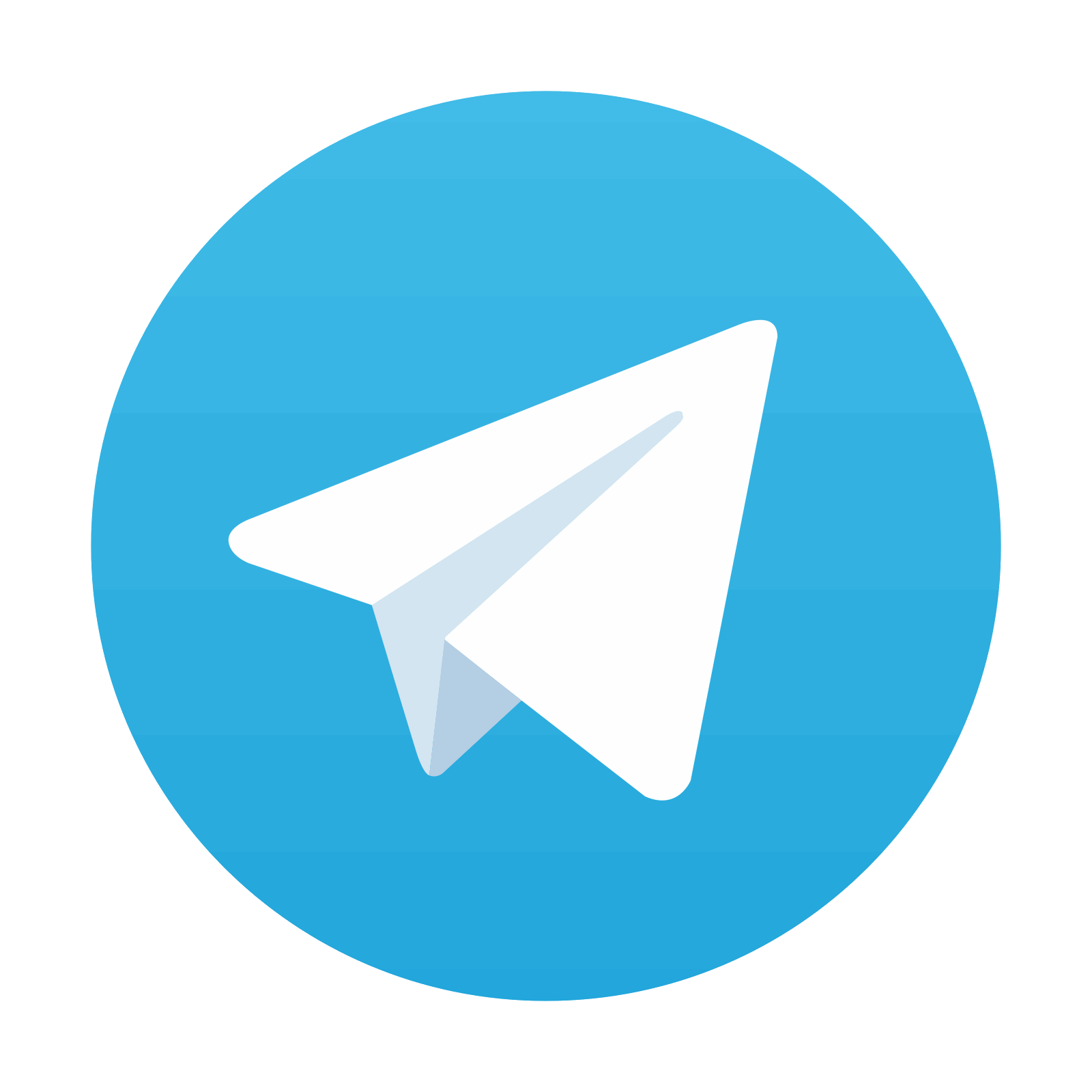
Stay updated, free articles. Join our Telegram channel

Full access? Get Clinical Tree
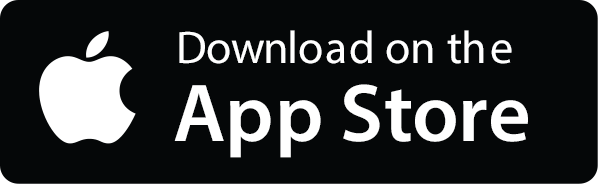
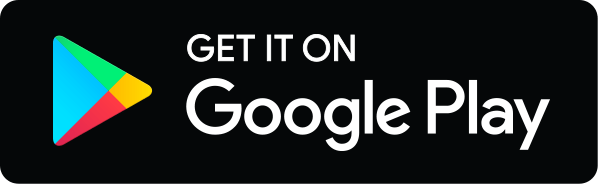