Introduction
Volume disorders are common in patients with cancer, given the vast array of organ dysfunction that occurs in these patients, as well as the frequent toxicities associated with many of the chemotherapeutics and biologics used for treatment. In healthy physiology, a complex system is in place to preserve volume homeostasis and maintain equilibrium across the major fluid compartments of the body. However, in disease states, several maladaptive changes can occur that disrupt this equilibrium and lead to volume disorders. This chapter describes the volume regulatory apparatus in the body and clinical effects that develop in pathologic cancer states. The various pathways of this system are directly connected to the final common endpoint of alterations in sodium and water handling by the kidneys. The frequent etiologies of hypovolemic and hypervolemic disorders in cancer patients are reviewed, and further discussion is focused on assessing and treating patients with extracellular fluid (ECF) volume contraction.
Volume homeostasis
Under normal physiologic conditions, the human body tightly regulates fluid volume. This is critical to maintain adequate perfusion of organs and ultimately to provide nutrients to cells and remove waste products. The system for regulating volume is complex, and it consists of a number of afferent sensors and efferent pathways that adapt to fluid changes to maintain homeostasis ( Fig. 5.1 ). Central to volume homeostasis are the kidneys, which regulate salt and water excretion. Additional critical components include the heart, the central nervous system, the vascular tree, and both volume and pressure baroreceptors. Although direct insults to any of these organ components can result in a fluid disorder, so too can insults to nearly any other organ system in the body, irrespective of its primary role in volume homeostasis. As an example, volume disorders are ubiquitous with damage to organs such as the kidneys and heart; however, disease processes involving other organs, such as the intestines, skin, and liver are also commonly associated with significant volume disturbances. For this reason, cancer patients are at significant risk for fluid disorders from a number of different pathologies.

It is important to appreciate that the body does not function like a water-filled balloon, shrinking when water is removed, and expanding when water is added. Rather, the central teaching in volume homeostasis is that there are several key fluid compartments within the body and adequate distribution of water between these compartments is critical ( Fig. 5.2 ). Total body water volume is roughly equal to 60% of the body weight in men, and 50% of the body weight in women and the elderly. The intracellular fluid (ICF) compartment contains 55% to 65% of total body water, and the ECF compartment contains the remaining 35% to 45%. The ECF compartment is further divided into the interstitial fluid compartment (approximately 75% of the ECF volume) and the intravascular compartment (approximately 25% of the ECF volume). Fluid can readily move across cell membranes, and therefore the volume of both the ICF and the ECF is dependent upon the gradient of effective osmotic agents between these two spaces. Sodium is the predominant osmotic agent in the ECF, and potassium is the predominant osmotic agent in the ICF. The Na + /K + adenosine triphosphatase pump, which is found in the cell membrane of nearly every cell within the body, maintains the distribution of sodium in the ECF compartment and potassium in the ICF compartment. Water will shift across cell membranes to maintain balanced osmolality between the ECF and ICF. Because the body’s regulatory mechanisms for sensing volume are mainly present in the ECF, sodium is the major factor that impacts the volume status of the entire body. Excessive sodium intake can lead to increases in ECF fluid volume that clinically manifest as hypertension, edema, and dyspnea. Alternatively, sodium losses can lead to decreases in ECF volume that clinically manifest as hypotension, increased skin turgor, and dizziness.

Volume regulation
The body’s ability to regulate volume is critical to one’s survival; as such, a complex set of mechanisms is present to preserve homeostasis. When the body begins to accumulate excess fluid, the fluid will distribute into the ECF and ICF based on the concentration of effective osmolytes within each compartment (predominantly sodium in the ECF and potassium in the ICF). When ECF volume increases, the body’s volume regulatory mechanisms will lead to adaptations that ultimately result in an increase in salt and water excretion by the kidneys to reduce the body volume. Alternatively, when ECF volume decreases, regulatory mechanisms will lead to adaptations that result in a decrease in salt and water excretion by the kidneys, in an effort to preserve body volume. The volume sensing components within the body predominantly respond to changes within the intravascular compartment of the ECF. Therefore even though the intravascular volume consists of less than 10% of the total body water, its role in regulating body volume is critical. In healthy physiology, the intravascular volume and the ECF are in equilibrium. From a physiologic stand point, the emphasized importance of the intravascular volume, and in particular the arterial vascular volume, makes a lot of sense, as this is what determines organ perfusion and overall survival. The term effective arterial blood volume specifically refers to the arterial volume that interacts with the volume sensing components and ultimately drives the response of the kidneys and other vascular mediators.
Integrated responses to volume changes
Changes in ECF volume trigger a number of mechanisms that act to adjust sodium and water excretion by the kidneys ( Fig. 5.3 ). These mechanisms include low pressure receptors in the right atrium and pulmonary blood vessels, as well as baroreceptors in the carotid sinus and aortic arch. In volume depleted states, stretch receptors at these sites upregulate sympathetic nerve activity in the kidneys. This results in a number of effects: (1) reduced glomerular filtration rate (GFR) via afferent arteriole constriction; (2) upregulation of sodium reabsorption in the nephron tubule; and (3) activation of the renin-aldosterone-angiotensin-system (RAAS). In addition, volume depleted states result in a decrease in atrial natriuretic peptide release by the cardiac atria, which further decreases GFR and also promotes sodium and water reabsorption along the nephron, in particular in the collecting duct. Volume overloaded states have the opposite effect on these systems, as they promote sodium and water excretion in the kidney. These integrated responses work together to restore body volume in healthy states. However, in maladaptive states, such as heart failure and cirrhosis, these mechanisms become disassociated from the body volume. More specifically, the ECF and intravascular volumes are no longer in equilibrium. Therefore, elevated ECF volumes are actually worsened because of the coexistence of low effective arterial volumes.

Volume disorders in the cancer patient
Volume depletion
The cancer patient is particularly vulnerable to volume depletion because of disorders of the gastrointestinal tract. Reduced fluid intake is common in cancer patients and etiologies for this include anorexia, mucositis, refractory nausea, and dysfunction of the oropharynx and esophagus. These insults can be direct consequences of the underlying malignancy, as well as the chemotherapeutic agents used to treat the underlying cancer. Despite severe reductions in fluid intake in this setting, the body continues to have unregulated insensible water losses from the skin and respiratory tract, and this can account for up to a liter or more per day. Patients in this scenario tend to develop hypernatremic hypovolemia, or what is more commonly referred to as dehydration , given the net loss of more free water than sodium. Another common cause of volume depletion in cancer patients is extensive sodium and water losses from vomiting or diarrhea. Patients may maintain oral hydration in this scenario, but it is often not enough to match the losses, and patients ultimately become volume depleted. The net result in these patients is more sodium loss than water loss, and they typically develop hyponatremic hypovolemia. Patients with substantial vomiting will also be at risk for metabolic alkalosis, given the significant amount of hydrogen and chloride loss in the gastric fluids. This can further promote potassium losses from the kidney because of sodium and bicarbonate delivery to the distal nephron, so called bicarbonaturia . On the other hand, in those subjects with diarrhea, a nonanion gap metabolic acidosis typically occurs because of bicarbonate losses from the intestinal fluids.
Polyuria in the setting of diabetes insipidus is another potential cause for volume depletion in patients with cancer. Central diabetes insipidus can result from metastatic tumors in lung cancer, leukemia, and lymphoma. Alternatively, nephrogenic diabetes insipidus can occur in the setting of tumor related obstructive uropathy, hypercalcemia, or ifosfamide and pemetrexed administration. , These patients present with polyuria and polydipsia, and often times daily urine output will exceed 5 L. Typically, they maintain euvolemia and isonatremia by drinking excess amounts of free water; however, in settings of impaired thirst or when patients are unable to access water because of barriers from changes in mentation or physical barriers, these subjects then develop hypernatremia and hypovolemia.
Volume overload
Increases in ECF volume are also common in oncology patients and these disorders can often be difficult to manage. Typically, this occurs because of insults to the heart, liver, or kidney, and the etiology can either be directly from the malignancy or from chemotherapeutic treatments. Common cardiac insults include chemotherapeutic-induced cardiomyopathies from anthracyclines (doxorubicin, daunorubicin, and epirubicin) and the human epidermal growth factor receptor 2 (HER2) modulator trastuzumab. In addition, primary malignant tumors of the heart, such as sarcomas, lymphomas, and pericardial malignancies, can also result in severe left ventricular dysfunction, valvular disease, or obstruction. In these scenarios, cardiac output declines and venous congestion occurs, resulting in a reduction of effective arterial volume. This results in neurohormonal changes that leave the kidneys in a sodium avid state in the proximal tubule and distal convoluted tubule because of upregulation of angiotensin II, aldosterone, and the sympathetic nervous system, and increased free water reabsorption in the cortical and medullary collecting ducts because of increased vasopressin. These patients often develop volume overload in the venous compartment of the vasculature system with normal to low serum sodium levels. In addition to using loop diuretics, other strategies for treating these patients include neurohormonal blockade with angiotensin converting enzyme inhibitors, angiotensin receptor blockers, mineralocorticoid receptor antagonist, beta-blockers, and sacubitril. , New evidence also supports specific renal and cardiac benefits from sodium glucose transport protein 2 SGLT2 inhibitors, and some patients may also benefit from low-dose dopamine, hypertonic saline combined with high dose diuretics, or vasopressin 2 receptor antagonist (vaptans).
Liver injury is another cause of volume overload in the oncology patient. This can result from parenchymal damage because of primary malignancies of the hepatobiliary tract, as well as the presence of metastatic tumors, which can commonly occur with colon, gastric, pancreas, and breast cancers. In addition, chemotherapy induced hepatotoxicity can also occur. The state of volume overload in liver injury is typically driven by the distortion in hepatic architecture leading to portal hypertension. This results in nitric oxide and carbon monoxide mediated splanchnic vasodilation, with secondary pooling of blood volume in the splanchnic vessels. In this situation, blood is stolen from other arterial beds, leading to a decrease in effective arterial volume. As a consequence of this, baroreceptor-mediated activation of the RAAS, sympathetic nervous system, and nonosmotic release of antidiuretic hormone occurs in an effort to restore arterial volume. Similar to congestive heart failure, these neurohormonal changes result in sodium and water retention in the kidney, which leads to volume overload. In addition to sodium restriction of less than 200 mg per day, patients with cirrhosis should be managed with diuretics to address volume overload. Guidelines recommend starting with 40 mg of furosemide and 100 mg of spironolactone, with titration upwards maintaining roughly the same ratio.
Kidney failure of any form can result in volume overload given the reduction in sodium and water excretion. This is common in clinical scenarios with a significant reduction in GFR. However, injuries that result in nephrotic-range proteinuria tend to present with more severe degrees of volume overload, even when the GFR is intact or modestly reduced. Recent evidence suggests that this is a result of serine protease activation of the epithelial sodium channel in the collecting duct. , These injuries are common in patients with cancer and can result as a paraneoplastic syndrome. Membranous nephropathy is the most frequent form of cancer-related nephrotic syndrome, and this occurs in the setting of breast, lung, and colon cancers, but minimal change disease and focal segmental glomerulosclerosis are also well described paraneoplastic lesions. In addition, targeted anticancer agents with antivascular endothelial growth factor properties can also result in proteinuria with volume overload. Agents most likely to cause this include the antiangiogenesis drugs bevacizumab, sorafenib, sunitinib, vatalanib, and axitinib. Loop diuretics are key to treating volume overload in renal failure, and when high doses of these agents are ineffective, the addition of a thiazide diuretic can be helpful. In states with coexisting proteinuria, angiotensin converting enzyme inhibitors or angiotensin receptor blocking agents should be coadministered given their antiproteinuric effects.
Fluid resuscitation
Assessing volume status and volume responsiveness
Accurately assessing volume status remains an important task for clinicians, and unfortunately one that is particularly challenging. In critically ill, volume depleted patients, studies have shown that both inadequate replacement of intravascular volume, as well as overly aggressive resuscitation, are associated with poorer outcomes. When considering the administration of intravenous volume to a patient suspected of having ECF contraction, clinicians should appreciate that the fundamental aim is to increase stroke volume and improve tissue and organ perfusion. It is therefore important to not only assess volume status, but also to anticipate and assess whether the patient is volume responsive, as these variables can be mutually exclusive.
The history and physical examination should be the initial parameters used to assess a patient’s need for volume replacement, as these are readily available ( Box 5.1 ). This includes a thorough history inquiring about recent reductions in oral fluid intake, as well as asking about increased fluid losses from vomiting, diarrhea, and bleeding. Routine evaluation of the blood pressure, pulse, and the degree to which these measurements vary with postural changes should also be done. Assessing mentation, capillary refill, skin turgor, dryness in the axilla and mucosal membranes, temperature in the extremities, and urine output are also part of the basic physical examination to guide fluid management. However, it should be noted that studies have shown poor correlations between physical examination findings and accurate determination of volume status. In fact, data have shown that postural changes in heart rate and blood pressure may be lacking with nonblood loss volume depletion (such as reduced oral intake, vomiting, and diarrhea) or when blood loss is only moderate (< 1 L). , Therefore clinicians should appreciate the challenge associated with predicting volume status and volume responsiveness based on physical examination findings alone. In many situations, additional testing is performed to further guide fluid management.
Vital signs:
Blood pressure
Pulse
Orthostatic changes of blood pressure and pulse
Physical examination:
Mentation
Dry mucous membranes and axilla
Capillary refill
Skin turgor
Urine output
Quality of peripheral pulses
Temperature in extremities
Laboratory findings:
Serum sodium
BUN/Creatinine
Urine sodium
Blood lactate
Brain-natriuretic peptide
BUN , Blood urea nitrogen.
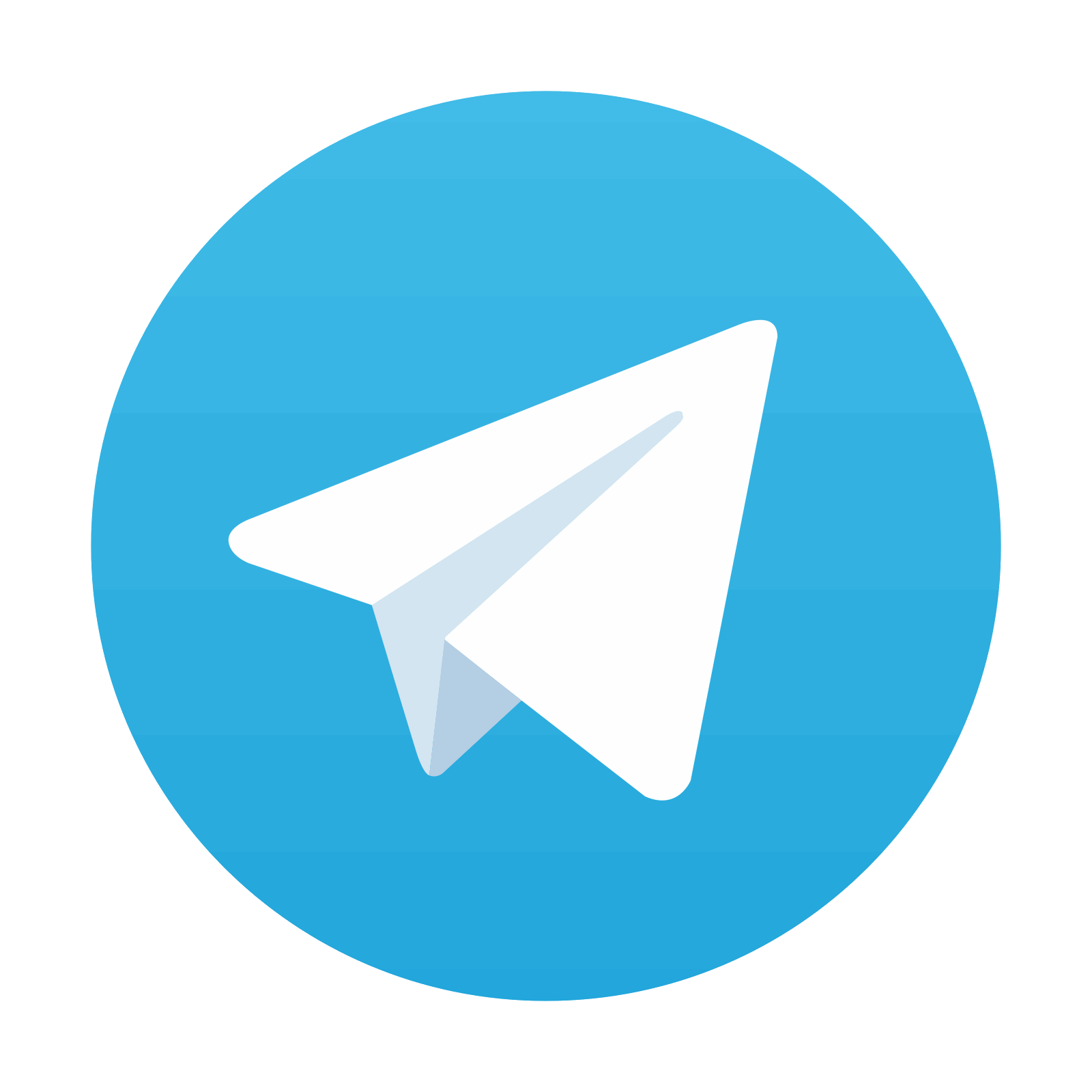
Stay updated, free articles. Join our Telegram channel

Full access? Get Clinical Tree
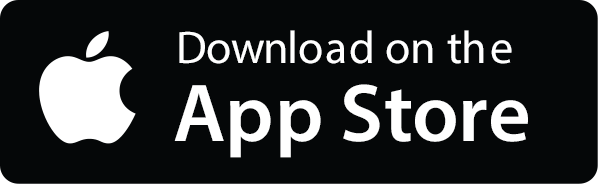
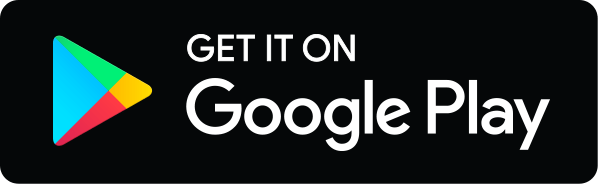
