INTRODUCTION
AKI is broadly defined as a rapid deterioration in kidney function as manifested by a reduction in GFR. The term acute kidney injury is intended to highlight the potential reversible nature of the injury, whereas the prior term acute renal failure (ARF) implicates renal impairment that is sustained. AKI is comprised of a variety of syndromes that are characterized by kidney dysfunction that occurs over hours to days. AKI can occur in the patient with completely normal kidney function or superimposed on chronic kidney disease (CKD). The loss of kidney function results in the accumulation of nitrogenous wastes within body fluids that would otherwise be excreted by the kidneys. The most commonly employed markers of AKI are serum creatinine and BUN, both of which rise in this setting. AKI may also cause disturbances in salt and water balance, potassium and phosphorus retention, acid–base homeostasis, and endocrine abnormalities. Descriptive terms in the setting of AKI include the following:
1. Azotemia—A buildup of nitrogenous wastes in blood.
2. Uremia—A constellation of symptoms and signs of multiple-organ dysfunction caused by retention of “uremic toxins” in the setting of renal failure.
Urine output is highly variable in the setting of AKI. It is often oliguric (<400 mL/day), but may be nonoliguric, with urine volumes actually exceeding 3 L/day (polyuric). In certain clinical states, urine output will be less than 100 mL/day, defined as oligoanuric or anuric (no urine output). Therefore, it is important to recognize that the presence of urine output does not exclude the possibility of AKI. In general, the level of kidney impairment in AKI includes a spectrum ranging from mild and rapidly reversible to very severe with a prolonged course and often a poor outcome. As is discussed later, the etiology of AKI, as well as the population of patients in which it occurs, determines the ultimate clinical course of AKI.
Prior to 2002 there was not a precise universal definition of AKI. In recent years, 2 evidence-based validated classification systems have been used in clinical settings to define AKI: RIFLE criteria and AKIN staging. The Acute Dialysis Quality Initiative workgroup in 2002 proposed the RIFLE criteria, an acronym that defines AKI with 3 grades of increasing severity (Risk, Injury, Failure) and outlines 2 outcomes variable (Loss and End-stage) (Figure 15.1).
FIGURE 15-1. The RIFLE and AKIN classifications of acute kidney injury are noted. RIFLE is based on serum creatinine, estimated GFR, and urine output. AKIN is based on serum creatinine (Cr) and urine output (UO). (With permission from BioMed Central Publishing. Cruz DN, Ricci Z, Ronco C. Clinical review: RIFLE and AKIN—time for reappraisal. Crit Care. 2009;13:211.)
In 2007, the Acute Kidney Injury Network (AKIN) modified the RIFLE criteria, which increases the sensitivity of RIFLE–Risk by including a small absolute change in serum creatinine of greater than 0.3 mg/dL in addition to the prior criteria, which would be AKIN Stage I. AKIN Stage II is identical to the RIFLE–Injury criteria. Finally, any patient receiving renal replacement therapy (RRT) would also be classified as AKIN Stage 3 (RIFLE–Failure). Furthermore, AKIN staging requires that the abrupt change in kidney function be within 48 hours, versus 1 week with RIFLE. Both classification systems incorporate urine output criteria as seen in Figure 15.1.
The addition of an absolute change in serum creatinine of equal to or greater than 0.3 mg/dL is based on epidemiologic data that demonstrated an 80% increase in mortality risk associated with changes in serum creatinine concentrations as little as 0.3 to 0.5 mg/dL. The inclusion of a time constraint of 48 hours is based on data that showed worse outcomes associated with small changes in the serum creatinine when the rise in creatinine was observed within 24 to 48 hours.
The Kidney Disease Improving Global Outcomes (KDIGO) proposed acute kidney diseases and disorders (AKD) as another category of kidney disease that includes AKI alone, AKI superimposed on CKD, AKD without AKI, and AKD superimposed on CKD (Table 15.1). No kidney disease (NKD) is an additional category added by the group. With the additional category of AKD, kidney diseases and disorders that do not meet either AKI or CKD definition yet, but have alterations in kidney function or structure, can be identified. This approach will allow patients with these kidney disorders to have evaluation and treatment that restores kidney function or reverses kidney damage.
TABLE 15-1. Definitions of AKI, CKD, and AKD
KEY POINTS
MEASURES OF KIDNEY FUNCTION
Although serum creatinine concentration is the most commonly employed clinical laboratory measure of kidney function, it actually is a poor reflection of true GFR in many patients. This problem exists because changes in serum creatinine concentration do not precisely correlate with changes in GFR. The concentration of serum creatinine is influenced by a number of factors.
1. In the setting of kidney disease, creatinine is cleared from the body by the kidney through both glomerular filtration and tubular secretion.
2. Certain drugs compete with tubular secretion of creatinine (trimethoprim, cimetidine) and may increase serum creatinine concentration in the absence of any change in GFR.
3. The reported serum creatinine concentration can be falsely elevated by interference with the laboratory technique used to measure creatinine (certain cephalosporins, endogenous chromophores).
4. The gender and muscle mass of the patient influence the serum creatinine concentration and can mask changes in GFR. This results because muscle is the primary source of creatine, which is converted to creatinine in the liver. Female gender and severe muscle wasting will reduce the production of creatine and limit the rise in serum creatinine that would normally accompany a reduction in GFR.
The relationship between BUN and GFR is even more confounded. First, renal handling of urea includes glomerular filtration as well as tubular secretion and reabsorption. Thus, any disease state associated with reduced tubular flow rates will increase urea reabsorption in the kidney and increase BUN. Second, multiple factors increase BUN in the absence of changes in GFR. They include protein loading (total parenteral nutrition, high-protein supplements), hypercatabolic states (infection, steroids, etc.), gastrointestinal (GI) bleeding (reabsorbed blood converted to urea), and tetracycline antibiotics (increase urea generation). Alternatively, BUN may remain very low despite significant kidney dysfunction in states such as cirrhosis (reduced urea generation), poor protein intake, and protein malnutrition, all of which are associated with decreased urea generation.
In spite of the problems associated with serum creatinine and BUN as accurate estimates of GFR, they are the most commonly employed laboratory tests to identify AKI. Clinicians use these less than optimal markers of kidney function because they are readily available, are familiar to all physicians, and though alternative tests may identify AKI earlier, there has not yet been a commercially standard use of these. Better measures of GFR, such as technetium-labeled iothalamate, are not practical in the acute clinical situation and not widely available. Inulin clearance, the gold standard measure of GFR, is strictly a research tool. Estimates of GFR or creatinine clearance, such as those based on the Modification of Diet in Renal Disease (MDRD) formulas and Cockcroft-Gault formula, were only tested in patients with stable CKD and would probably be inaccurate in the setting of AKI with a rapidly changing GFR.
These above limitations have led to new techniques based on proteomics to identify novel biomarkers of AKI. The goals of biomarker research are earlier diagnosis of AKI, appropriate differential diagnosis of AKI, and prediction of AKI that will worsen, require RRT and associate with higher mortality. Early diagnosis would permit the appropriate preventive and preemptive strategies to be implemented, and treatment regimens offered such that permanent loss of function can be avoided. In patients who develop AKI, biomarker concentrations demonstrate changes earlier than do serum creatinine concentrations (Figure 15.2). Biomarkers may also distinguish between prerenal azotemia (prerenal AKI), acute tubular necrosis (ATN), and other glomerular disorders, allowing appropriate interventions and avoiding potentially harmful therapies such as continued aggressive intravenous fluid therapy in a patient with ATN (risk of volume overload and other end-organ consequences). Finally, the ability of biomarkers to predict outcomes such as worsening kidney function (ie, progression to higher AKIN stages), RRT requirement, and mortality is of highest interest for nephrologists caring for patients with AKI.
FIGURE 15-2. Evolution of acute kidney injury. Measurement of different biomarkers can detect injury before a decrease in GFR and can also be used for diagnostic and prognostic assessment. Abbreviations: CRO, C reactive protein; CysC, cystatin C; GFR, glomerular filtration rate; GST, glutathione-S-transferase; IL-6, interleukin-6; IL-18, interleukin-18; KIM-1, kidney injury molecule-1; L-FABP, liver fatty-acid-binding protein; NGAL, neutrophil gelatinase-associated lipocalin. (With permission from Elsevier. Bellomo R, Kellum JA, Ronco C. Acute kidney injury. Lancet. 2012;380:756-766.)
The expression of the NGAL (neutrophil gelatinaseassociated lipocalin; also called lipocalin-2 or siderocalin) messenger ribonucleic acid and protein in the kidney has been shown to be significantly increased in the kidney tubules of patients with ischemic, septic, or posttransplantation AKI, as well as within 2 to 6 hours post–cardiopulmonary bypass surgery, and even following contrast administration. The cytokine interleukin (IL)-18, which is formed in the proximal tubules and can be detected in the urine, is a candidate biomarker for renal parenchymal injury. Patients with ATN had significantly higher levels of IL-18 in their urine than did control subjects or persons with other forms of kidney disease. Another molecule that is upregulated in postischemic injury in the proximal tubule is kidney injury molecule 1 (KIM-1). Urinary KIM-1 has been suggested as another biomarker for the diagnosis of ischemic ATN. Cystatin C is a protease inhibitor that may be an alternative to serum creatinine in the measurement of renal function of the GFR. In contrast to other biomarkers, serum cystatin C levels are usually noted when tissue injury has led to significant changes in the filtration function.
These biomarkers can also be utilized to better identify subsets of patients who would otherwise not be identified as having AKI with our current serum creatinine-based and urine output-based criteria. Biomarkers measured on the day of AKI diagnosis improve risk stratification and identify patients who are at higher risk for progression of AKI and worse patient outcomes. Moreover, by identifying possible mechanisms of injury, novel biomarkers increase our understanding of the pathogenesis of AKI. Further research will be needed for the development and clinical validation of new biomarkers for the eventual definition of kidney injury. As these new biomarkers evolve, so will our understanding of AKI. Presently, these biomarkers are not readily available for clinical use and are under clinical investigation. Whether a panel of biomarkers would provide complementary information and be practical in use compared with a single biomarker approach remains to be determined. Consequently, the clinician should assess the patient with suspected AKI using all of the clinical tools currently available yet recognize their limitations.
KEY POINTS
EPIDEMIOLOGY OF ACUTE KIDNEY INJURY
AKI is a frequent problem in hospitalized patients, whereas it is less common in the community setting. Clearly, the actual incidence and outcomes of AKI are dependent on the definition used, as well as the patient population evaluated. A few studies have been published that evaluate the incidence and etiology of community-acquired renal failure, as well as the AKI that develops in hospitalized patients.
In a study designed to examine community-acquired AKI, renal failure was defined as an increase in serum creatinine concentration of 0.5 mg/dL in patients with a baseline less than 2.0 mg/dL, a rise of 1.0 mg/dL in patients with a baseline between 2.0 and 4.9 mg/dL, or a rise of 1.5 mg/dL in patients with a baseline greater than 5.0 mg/dL. The incidence of AKI on admission to the hospital was 0.9%. Approximately half of the patients had AKI superimposed on CKD. Prerenal AKI accounted for 70% of the cases, whereas obstructive uropathy caused 17%. Intrinsic AKI from various etiologies resulted in only 11% of the AKI cases. Overall mortality was 15% in patients with AKI. Mortality was highest in patients with intrinsic kidney failure (55%) and lowest in patients with prerenal azotemia (7%). As will be seen in the discussion of hospital-acquired AKI, the mortality of community-acquired AKI is much less when compared with that seen in the hospital.
Two studies evaluated the incidence of AKI in the hospital. It is worth noting that the incidence of hospital-acquired AKI is higher than that seen with AKI that develops in the community. In a study performed in 1979, the incidence of AKI was 4.9% of all hospital admissions when a definition of AKI similar to the one employed above was used. Once again, prerenal AKI was the most common cause of AKI (42%), whereas postoperative AKI resulted in 18%, radiocontrast material in 12%, and aminoglycosides in 7% of episodes. Overall mortality associated with AKI was 29% and mortality was highest in patients with a serum creatinine concentration greater than 3 mg/dL (64% vs. 3.8% in patients with serum creatinine concentration <2 mg/dL). As noted, this study represents trends in AKI that occurred in the late 1970s. In 1996, the same group of investigators performed a similar study to determine if the incidence of and mortality associated with AKI changed. They postulated that the population of patients studied in this time period were older, possessed higher comorbidities, and received more nephrotoxic medications, placing them at higher risk for AKI. When compared with the study 20 years earlier, the incidence of hospital-acquired AKI increased slightly to 7.2%. Once again, decreased renal perfusion remained the most common cause of AKI (39%). This was followed by nephrotoxic drugs (aminoglycosides and nonsteroidal antiinflammatory drugs [NSAIDs]) causing 16%, radiocontrast material causing 11%, and postoperative renal impairment causing 9% of the episodes of AKI. CKD was a common underlying risk factor for AKI as compared with patients with normal kidney function. Remarkably, the overall mortality was 19.4%, lower than the mortality noted 20 years prior. This may reflect improved supportive care and advances in several life-saving technologies. Mortality, however, remained high in patients with serious illnesses, such as sepsis (mortality 76%), when AKI developed. As seen previously, the correlation between severity of AKI and mortality was again observed.
The mortality associated with a hospitalized AKI patient depends on the severity of illness and burden of organ system dysfunction that the patient suffers from. For example, whether quantifying disease severity by number of failed organ systems or Acute Physiology and Chronic Health Evaluation (APACHE) II or III score, the mortality increases as the severity of patient illness increases. As the number of organs failed increased from zero to 4, the mortality associated with AKI increased from less than 40% to above 90%. Similarly, the mortality associated with AKI progressively increased from less than 10% with an APACHE III score less than 50, to 52% with a score of 51 to 70, to 58% with a score of 71 to 90, to 86% with a score of 91 to 110, and to 100% with a score greater than 110. As one might suspect when examining these data, the mortality associated with AKI that develops in the medical or surgical intensive care unit is extremely high.
A metaanalysis that examined long-term outcomes of AKI, defined as at least 6 months of follow-up, published in 2009 summarized that AKI (variable definitions) had an increased long-term risk for death compared to hospitalized patients without AKI (relative risk [RR] 2.62; 95% confidence interval [CI] 1.99 to 3.45). A number of other studies have reported on the long-term impact of AKI on CKD. For example, CKD patients with an eGFR <45 mL/min per 1.73 m2 pre-hospitalization who experienced severe AKI, defined as RRT-requiring AKI, had a 26.3% rate of death during the hospitalization compared to 4.7% among CKD patients without AKI. Among this population of RRT-requiring AKI patients with underlying CKD, within 30 days of discharge 66% or survivors received a diagnosis of ESRD compared to 1.5% of non-AKI survivors. Another study examined patients with preserved kidney function, an enhanced GFR (eGFR) greater than 45 mL/min per 1.73 m2 who did not develop AKI and those who developed RRT-requiring AKI. The RRT-requiring AKI group had a 28-fold increased risk of CKD progression. Several studies examining the outcomes of hospital-acquired reversible AKI (within 48 to 72 hours) demonstrated higher mortality rates compared with patients with nonprogressive community-acquired AKI and with control patients without AKI. Combined, these studies suggest that all forms of AKI, including the RRT-requiring forms, appear to be associated with an increased risk for development of new CKD, progression of CKD, end-stage renal disease (ESRD) and death. It is important to recall, however, that many of these studies suffer from various forms of confounding, making it hard to definitively state that AKI in and of itself leads to CKD/ESRD and mortality.
KEY POINTS
CLINICAL ASSESSMENT OF ACUTE KIDNEY INJURY
Table 15.2 provides a list of the etiologies of AKI classified as prerenal, intrinsic renal, or postrenal. Figure 15.3 is a schematic of the various causes of AKI. A logical approach to AKI is achieved by broadly classifying the clinical causes into the following:
TABLE 15-2. Etiologies of Acute Kidney Injury
FIGURE 15-3. Etiologies of acute kidney injury. Common causes of acute kidney injury are noted in this schematic.
1. Prerenal AKI—A decrease in GFR that occurs as a consequence of reduced renal blood (plasma) flow and/or reduced renal perfusion pressure.
2. Intrinsic AKI—A decrease in GFR as a result of direct parenchymal injury in the kidney, often subdivided by the various compartments involved (vascular, glomerular, interstitial, and tubular).
3. Postrenal AKI—A decrease in GFR primarily as a result of an obstruction to urine flow anywhere from the pelvis and calyces to the urethra.
Prerenal AKI
AKI is classified as prerenal AKI when a patient exhibits a rising BUN and serum creatinine concentration caused by inadequate blood flow to the kidneys. To provide a framework to understand the concept of prerenal AKI, the following description of renal blood flow (RBF) is provided. The kidneys receive up to 25% of the cardiac output, which results in more than 1 L of RBF per minute. This high rate is necessary to not only maintain GFR, but also to preserve renal oxygen delivery (to sustain ion transport and other energy-requiring processes). Thus, normal kidney function is dependent on adequate perfusion. It is intuitive that a significant reduction in renal perfusion may be sufficient to diminish filtration pressure and lower GFR. Broad examples of prerenal AKI include the following causes of renal circulatory insufficiency:
1. Renal circulatory insufficiency from “true” intravascular volume depletion.
a. Hypovolemia from hemorrhage, renal losses (diuretics), GI losses (vomiting, diarrhea), third spacing, and severe sweating.
2. Renal circulatory insufficiency from “effective” intravascular volume depletion.
a. Impaired cardiac function from cardiomyopathy, hypertensive heart disease, valvular heart disease, pericardial disease, and severe pulmonary hypertension. Venous congestion from hypervolemia also contributes to reduced renal perfusion and reduced GFR.
b. Impaired liver function from acute hepatic failure and severe cirrhosis with hepatorenal physiology.
c. Impaired systemic vascular tone (inappropriate vasodilation) because of sepsis, medications, and autonomic failure.
3. Renal circulatory insufficiency caused by renal artery disease.
a. Main renal artery disease (renal artery stenosis).
b. Small renal vessel narrowing (hypertensive arterionephrosclerosis).
4. Renal circulatory insufficiency caused by altered intrarenal hemodynamics.
a. Afferent arteriolar vasoconstriction (NSAIDs, calcineurin inhibitors).
b. Efferent arteriolar vasodilation (angiotensin-converting enzyme [ACE] inhibitors, angiotensin receptor blockers [ARBs]).
Both “true” and “effective” hypovolemia activate several neurohormonal vasoconstrictor systems as mechanisms to protect circulatory stability. These include catecholamines from the sympathetic nervous system, endothelin from the vasculature, angiotensin II (AII) from the renin-angiotensin system (RAS), and vasopressin from the neurohypophysis. All of these substances raise blood pressure through arterial and venous constriction. They also possess, however, the ability to constrict the afferent arteriole and reduce GFR, especially when systemic blood pressure is inadequate to maintain renal perfusion pressure. Structural lesions in the renal arterial and arteriolar tree can also reduce perfusion and promote prerenal azotemia. In response to these hemodynamic challenges, renal adaptive responses are stimulated to counterbalance diminished renal perfusion, whether as a result of functional or structural causes. Myogenic influences and the production of vasodilator substances constitute these adaptive processes. The myogenic reflex is activated by low distending pressures sensed in the renal baroreceptors, thereby causing afferent arteriolar vasodilation. Prostaglandins (PGE2, PGI2), nitric oxide, and products from the kallikrein-kinin system modify the effects of above-noted vasoconstrictors on the afferent arteriole. Disturbance of the balance between afferent vasodilation and efferent vasoconstriction can disrupt intrarenal hemodynamics and precipitate AKI. Medications such as the NSAIDs and selective cycloxygenase-2 (COX-2) inhibitors act to cause prerenal azotemia through inhibition of vasodilatory prostaglandins in patients who require prostaglandin effects to maintain renal perfusion. Despite its vasoconstrictor properties, AII acutely preserves glomerular filtration pressure and GFR in states of reduced renal perfusion by constricting the efferent arteriole. This effect in part explains the reduction in GFR that occurs when an ACE inhibitor or an ARB is administered to a patient who is dependent on AII to constrict the efferent arteriole.
The cardiorenal syndrome (CRS) is an umbrella term that encompasses various cardiac or kidney derangements that coexist and their interplay. There are 5 subtypes of the CRS (Table 15.3). Hospital-acquired AKI caused by CRS is most often that of the type I variety. For example reduced stroke volume or cardiac output, arterial underfilling, elevated atrial pressures, and venous congestion, independently or in combination, cause impairments in the renal circulation, causing a reduction in GFR and AKI. The neurohumoral adaptations that occur to preserve perfusion to vital organs, such as activation of the sympathetic nervous system and renin-angiotensin-aldosterone system (RAAS) as well as increases in the release of vasopressin and endothelin-1, promoting salt and water retention and systemic vasoconstriction, can promote or exacerbate AKI by 2 mechanisms. First, these neurohormonal adaptations increase cardiac afterload and further reduce cardiac output and renal perfusion, and second, they increase central venous pressure, renal venous pressure and/or intraabdominal pressure, ultimately lowering GFR. Identifying AKI in the setting of heart failure is clinically relevant because reduced GFR is generally associated with a worse prognosis in these patients.
TABLE 15-3. Definition and Classification of the Cardiorenal Syndrome
Just as there is a strong physiologic interplay between cardiac dysfunction and kidney impairment, there is a strong association with liver disease and kidney impairment. In patients with advanced, decompensated cirrhosis or fulminant hepatic failure, a unique form of AKI, termed hepatorenal syndrome (HRS) may occur. It is important to note, that HRS is only a fraction of all AKI cases in cirrhotic patients and is a diagnosis of exclusion (that is difficult to firmly make). Table 15.4 summarizes the diagnostic criteria for HRS. There are 2 subtypes of HRS: Type 1 HRS is characterized by rapidly progressive renal failure defined by doubling of the initial serum creatinine concentrations to a level greater than 2.5 mg/dL in less than 2 weeks. Type 2 HRS is characterized by moderate renal failure (serum creatinine from 1.5 to 2.5 mg/dL). The hallmark of HRS is profound renal vasoconstriction in the setting of systemic and splanchnic arterial vasodilation. Figure 15.4 summarizes the hemodynamic changes that occur in HRS. Currently, there is no test that is specific for the diagnosis of HRS. The main differential diagnoses of type 1 HRS are ATN and prerenal AKI, as all of these conditions have an acute onset with progressive deterioration of kidney function. Although prerenal AKI can generally be recognized by response to intravenous fluids (albumin and saline), HRS type 1 and ATN are more problematic. Traditional markers such as urine microscopy (renal tubular cells and granular casts) and urine chemistries (fractional excretion of sodium or urea) to differentiate ATN from HRS are sometimes helpful, but can be insensitive. Therefore urinary biomarkers, including IL-18, NGAL, KIM-1, and others, are currently under investigation to differentiate between type 1 HRS and ATN. This is crucial as therapy for these 2 causes of AKI are very different—midodrine and octreotide, norepinephrine (or vasopressin) for HRS and supportive therapy for ATN. Liver transplantation (or combined liver-kidney transplantation) is the definitive therapy for HRS. Determining the etiology of AKI in cirrhotic patients is also critical for prognosis as HRS carries the worst survival among all causes of AKI in cirrhotic patients. It also heavily influences decisions such as the candidacy of a patient for liver transplantation and the utility of RRT in these patients.
TABLE 15-4. Hepatorenal Syndrome Criteria
FIGURE 15-4. Pathophysiology of hepatorenal syndrome. In cirrhosis, portal hypertension gives rise to compensatory splanchnic vasodilation, which creates a state of decreased effective circulating volume. In response, the RAAS and the SNS are activated, increasing renal sodium avidity, worsening ascites, and causing severe intrarenal vasoconstriction, the hallmark of HRS. Abbreviations: SBP, spontaneous bacterial peritonitis; SNS, sympathetic nervous system.
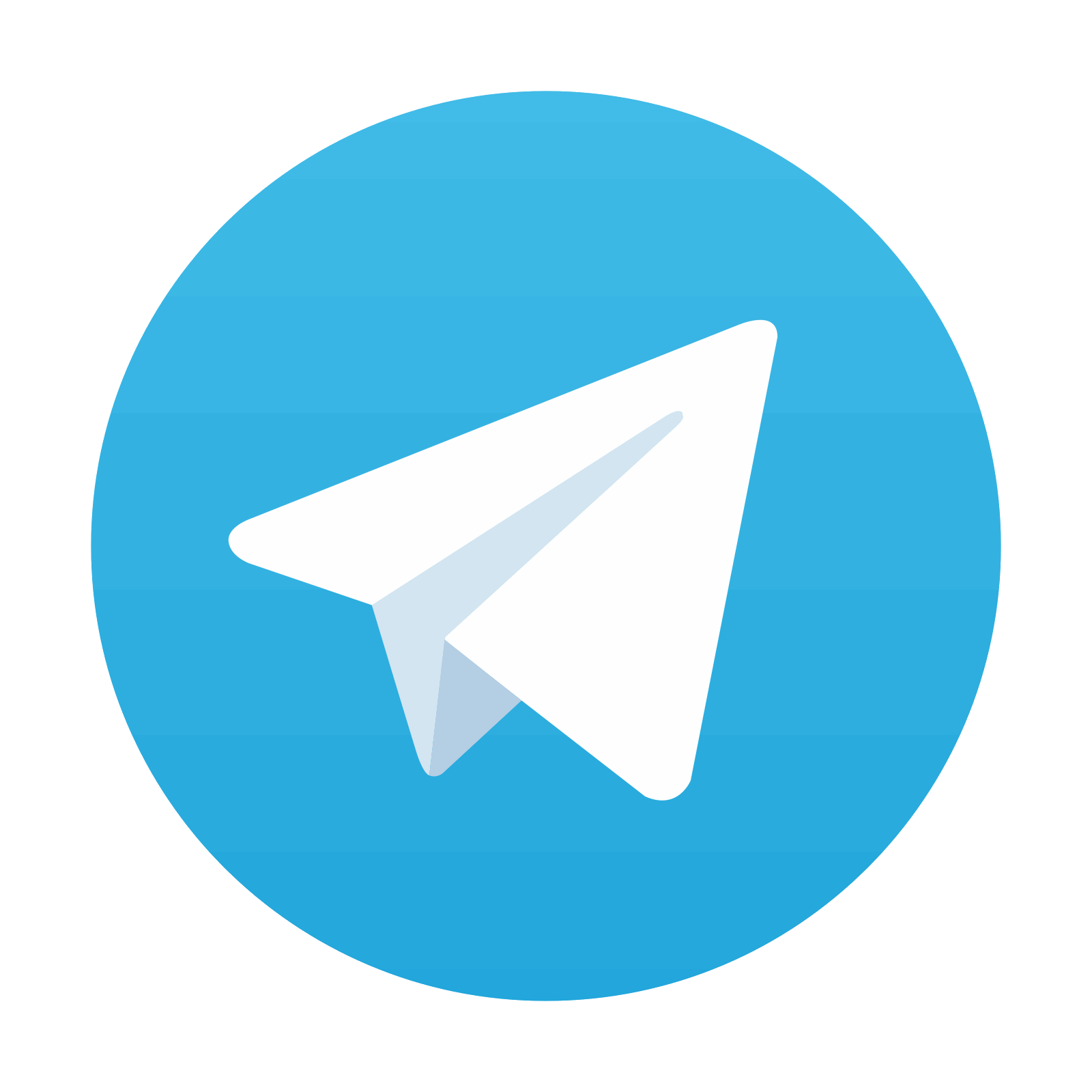
Stay updated, free articles. Join our Telegram channel

Full access? Get Clinical Tree
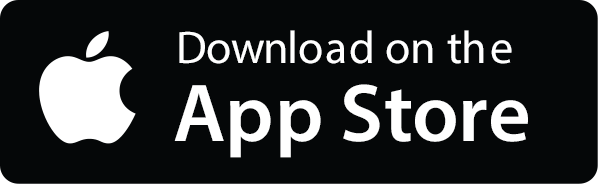
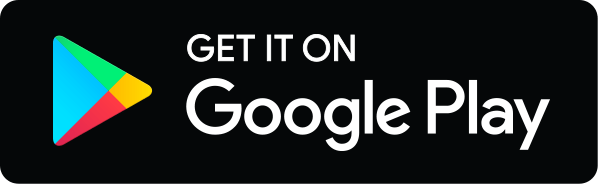