Essential mineral elements must also be absorbed to maintain normal physiological function and health. The typical diet contains several hundred milligrams per day of macrominerals such as calcium, sodium, potassium, magnesium, chloride, phosphorus, and sulfur. Microminerals or trace elements are present in smaller amounts, ranging from a few milligrams to micrograms per day. Essential trace elements including chromium, cobalt, copper, fluoride, iodide, iron, manganese, molybdenum, selenium, and zinc have well-established functions in human physiology. Other trace elements (arsenic, boron, cadmium, nickel, silicone, tin, vanadium) have physiological functions in some species, but an essential role in human metabolism had not been clearly defined. Minerals and trace elements are also present in various gastrointestinal (GI) secretions and are variably reabsorbed by the intestine. Minerals serve diverse physiological roles, including structural functions (bone minerals), components of metalloproteins (enzymes, transporters) and as ions involved in neurotransmission, muscle function, regulation of fluid and acid-base balance, energy gradients, and as second messengers.
Intraluminal factors substantially affect the efficiency of mineral and trace element absorption by altering the following processes: (1) intraluminal pH; (2) redox state of the metal; (3) formation of chelates that enhance solubility of the mineral; (4) formation of insoluble complexes that diminish absorption; and (5) digestion of proteins that are associated with dietary minerals. Kinetic studies of mineral uptake across the small intestine brush-border membrane generally have been consistent with facilitated diffusion or active transport when studied at low physiological concentrations, although many of these putative carriers have not been definitively identified. At higher concentrations, intestinal uptake via passive diffusion , either paracellularly or transcellularly, becomes quantitatively more important. Within the enterocyte, minerals often associate with intracellular ligands that play critical roles in regulating absorption and delivery of these elements into the circulation. Mechanisms for extrusion of minerals across the basolateral membrane into the portal circulation are incompletely characterized, but associations with transport proteins and other ligands are important. Effects of one mineral on the absorption of others are commonly observed, reflecting interactions in the luminal environment and shared mechanisms for absorption (Table 9.2). For example, high doses of zinc interfere with copper absorption. Individuals chronically taking zinc supplements for prevention of colds, etc. may develop copper deficiency with anemia , neutropenia, and bone disease. Zinc is used in the treatment of Wilson’s disease, a genetic disorder characterized by diminished biliary copper excretion and copper overload. Zinc treatment decreases intestinal copper absorption, contributing to a reduction in body copper.
Table 9.2
Common mineral interactions and antagonismsa
Mineral | Condition or state | Effect on net absorption |
---|---|---|
Iron | Fe deficiency | ↑ |
Fe excess | ↓ | |
Mn excess | ↓ | |
Co excess | ↓ | |
Conditions favoring Fe2+ | ↑ | |
Conditions favoring Fe3+ | ↓ | |
Copper | Cu deficiency | ↑ |
Cu excess | ↓ | |
Zn excess | ↓ | |
Cd excess | ↓ | |
Ag excess | ↓ | |
Conditions favoring Cu+ | ↓ | |
Conditions favoring Cu2+ | ↑ | |
Zinc | Zn deficiency | ↑ |
Zn excess | ↓ | |
Cu excess | ↓ | |
Cd excess | ↓ | |
Manganese | Mn deficiency | ? |
Mn excess | ? | |
Fe excess | ↓ | |
Co excess | ↓ |
A detailed consideration of the absorption of each of the water-soluble vitamins, minerals, and trace elements is beyond the scope of this book. In this chapter, two water–soluble vitamins, folate and vitamin B 12 , and two minerals, iron and calcium, will be considered in depth as illustrations of the important principles of intestinal absorption of these classes of nutrients.
2 Folate
2.1 Structure and Biochemical Function
The term folate denotes a family of compounds with nutritional properties and biochemical structures similar to the reference compound, folic acid or pteroylglutamic acid (Fig. 9.1). Folic acid comprises three moieties: a pteridine linked by a methylene bridge to para–aminobenzoic acid (PABA) and joined by a peptide bond to glutamic acid . The coenzyme forms of folate have reduced pteridine rings at positions 5, 6, 7, and 8 and one-carbon additions at N-5 or N-10. In addition, naturally occurring folates are mainly in the polyglutamate form, in which up to nine glutamate residues are conjugated via a unique γ-glutamyl bond forming a peptide chain (Fig. 9.1).
Folate is widely distributed in foods, with liver , yeast, leafy vegetables, legumes and some fruits being especially rich sources. Only a few foods, such as milk and egg yolk, contain principally monoglutamyl forms, whereas organ meats contain mainly penta- and heptaglutamates. Folic acid is the most common form of the vitamin in pharmaceutical preparations and has been used in much of the research on intestinal folate absorption. A substantial amount of folate is also secreted in bile, mainly as monoglutamyl 5–methyltetrahydrofolate, and must be reabsorbed by the intestine to maintain the normal folate economy.
Folates function metabolically as coenzymes in biochemical reactions that transfer one-carbon units from one compound to another. These reactions are important in amino acid metabolism and in nucleic acid synthesis. Folate deficiency therefore impairs cell division and alters protein synthesis, with the most prominent effects noted in rapidly growing tissues. The most common clinical manifestation of human folate deficiency is a macrocytic anemia , characterized by abnormally large red blood cells (macro–ovalocytes). Hypersegmentation of the chromatin of circulating neutrophils is also observed, and with severe folate deficiency neutropenia and thrombocytopenia may also be present. Bone marrow examination demonstrates abnormal precursors of red blood cells (megaloblasts), neutrophils, and platelets resulting from defective DNA synthesis.
2.2 Hydrolysis of Polyglutamyl Folates
In the lumen of the small intestine , polyglutamyl folates must be hydrolyzed to the monoglutamyl form before transport through the enterocyte (Fig. 9.2). Because of the unique γ–glutamyl linkage, polyglutamyl folates are not hydrolyzed by typical pancreatic or intestinal proteases, and specific enzymes known as folate conjugases are required.
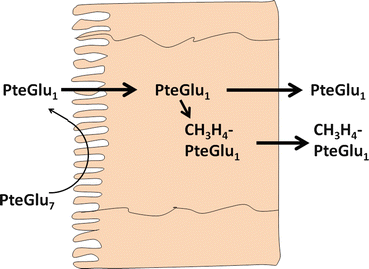
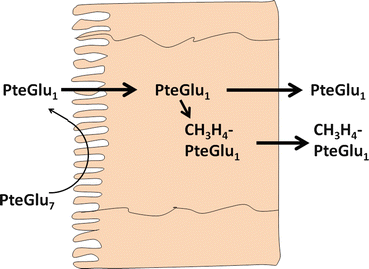
Fig. 9.2
Enterocyte metabolism and absorption of conjugated folates. PteGlu 7 heptaglutamyl folate , PteGlu 1 monoglutamyl folate, CH 3 H 4 PteGlu 1 5-methyltetrahydrofolate (Adapted from Mason and Rosenberg [7])
In humans, folate conjugase activities are present in both the small intestinal brush–border membrane and in an intracellular fraction composed mainly of lysosomes. The brush-border membrane enzyme appears to be responsible for the hydrolysis of dietary polyglutamyl folates. This enzyme has a pH optimum of pH 6.5–7.0, the typical pH of the upper small intestine , and is activated by Zn2+. The brush-border folate conjugase is an exopeptidase that sequentially cleaves glutamate residues from the end of the peptide chain, eventually producing the monoglutamate form. The function of the intracellular conjugase enzyme is currently unknown. This enzyme is an endopeptidase, cleaving the polyglutamate chain between the first and second glutamic acid residues. Intracellular conjugase has a pH optimum of pH 4.5, and no metal requirement for this enzyme has been defined. During folate digestion and absorption, monoglutamyl folates accumulate in the intestinal fluid, indicating that the rate–limiting step is the absorption of monoglutamyl folates rather than deconjugation of polyglutamate forms. Studies have shown that alcohol decreases brush-border conjugase activity, a factor that may contribute to the high prevalence of folate deficiency in alcoholics. Several drugs have also been shown to inhibit folate deconjugation, including the anti-inflammatory medication sulfasalazine used in the treatment of inflammatory bowel disease .
2.3 Absorption of Monoglutamyl Folates
Studies of the intestinal uptake of monoglutamyl folates using different experimental preparations have consistently demonstrated the presence of both saturable and nonsaturable uptake mechanisms. Saturable uptake appears to reflect carriermediated transport, whereas the nonsaturable component is due to diffusion through the cell membrane and/or through the paracellular pathway . At physiological concentrations, carrier-mediated transport accounts for most of the folate absorption, whereas diffusion predominates at high folate concentrations.
Two carriers involved in intestinal folate absorption have been identified, RFC (reduced folate carrier, the product of the SLC19A1 gene) and PCFT (the product of the SLC46A1 gene). RFC is expressed in the intestinal cell brush-border membrane and functions at neutral pH. PCFT is expressed mainly in the apical membrane of jejunal enterocytes with low expression in the ileum and colon. Transport of folate through the PCFT system is proton coupled and occurs via a folate–proton symport using energy generated from the downhill movement of protons into the enterocyte. It is possible that PCFT is responsible for folate absorption in the proximal small bowel where the luminal pH is somewhat acidic, whereas RFC plays a role in absorption of folate in the distal small intestine and colon. Mutation of the PCFT transporter results in hereditary folate malabsorption . Folic acid, reduced folates such as 5-methyltetrahydrofolate, and the antimetabolite methotrexate (a competitive inhibitor of the enzyme dihydrofolate reductase) all appear to share the same brush-border membrane carrier, with similar affinities for the transporter. Folate uptake, however, is structure-specific, as degradation products such as PABA or glutamic acid and inactive diastereoisomers of 5-methyltetrahydrofolate do not interact with the brush-border membrane transporter. In addition to its effect on folate deconjugation, sulfasalazine is also a competitive inhibitor of intestinal monoglutamyl folate transport. The mechanism of transport of folate across the basolateral enterocyte membrane is not well understood, but some data suggest involvement of MDR (multidrug resistance) proteins.
Bacteria in the intestine synthesize folate , with a substantial portion in the monoglutamate form. Although the contribution of colonic absorption of bacterially-derived folate via the RFC transporter expressed in the colonocyte brush-border membrane to folate economy is uncertain, it is possible that it plays a significant role in human nutrition, particularly supplying folate for colonocytes.
Folate digestion and absorption are both regulated by the level of folate in the diet. Folate deficiency causes an increase in folate conjugase and in carrier-mediated folate transport with increased expression of PCFT and RFC. Carrier-mediated folate transport and expression of RFC and PCFT increase as enterocytes mature to villus cells .
Some data have suggested an alternative mechanism for intestinal folate absorption in the neonate. Folate in milk is largely bound to a high–affinity folate–binding protein. In contrast to free folate, this protein-bound folate is more avidly absorbed in the ileum than jejunum and is not inhibited by sulfasalazine.
2.4 Folate Metabolism in the Enterocyte
At physiological concentrations, folic acid is largely reduced and methylated or formylated within the enterocyte. Appearance of folate in the blood is faster after intraluminal administration of 5-methyltetrahydrofolate folate than after folic acid, suggesting that folic acid reduction in the enterocyte via dihydrofolate reductase may be rate–limiting. At pharmacological concentrations, unmodified folic acid appears in the portal blood.
3 Vitamin B12
3.1 Structure and Biochemical Function
The basic structure of vitamin B12 is illustrated in Fig. 9.3. A central cobalt atom is surrounded by a planar corrin nucleus comprising four reduced pyrrole rings linked together. Below the corrin nucleus is a nucleotide moiety (1-α-D-ribofuranosyl-5,6-dimethylbenzimidazole-3-phosphate) that lies at a right angle to the corrin nucleus and is joined to the rest of the molecule at two points: (1) via a phosphodiester bond to a 1-amino-2 propanol group and (2) through coordination to the central cobalt via one of its nitrogens. The various forms of vitamin B12 have different anionic groups in coordinate linkage with the cobalt. The coenzyme forms of the vitamin are methylcobalamin and adenosylcobalamin (5′-deoxyadenosylcobalmin), formed via a unique carbon-cobalt bond. Other important forms of vitamin B12 are hydroxocobalamin and cyanocobalamin .
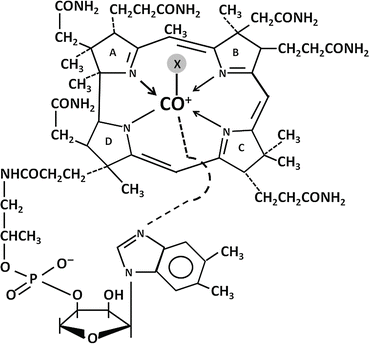
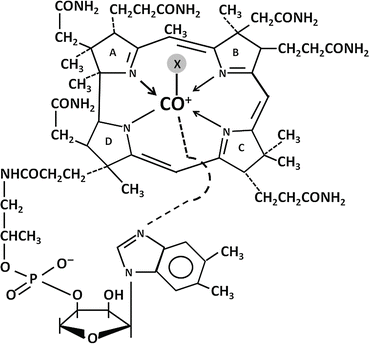
Fig. 9.3
Structure of vitamin B12. X, axial ligand in coordinate linkage with cobalt
Vitamin B12 is synthesized only by microorganisms, and in the human diet it is almost entirely by animal products. Strict vegetarians are therefore at increased risk for vitamin B12 deficiency. The daily losses of vitamin B12 are, however, very small compared with the body pool size, and 10–20 years of a deficient diet is required to produce clinically significant depletion. Meat contains mainly adenosyl- and hydroxocobalamin, whereas dairy products have predominantly methyl and hydroxocobalamin. Cyanocobalamin is a stable form of the vitamin used in pharmaceutical preparations. Cyano- and hydroxocobalamin are readily converted to the coenzyme forms by enzyme systems found in the cytoplasmic and mitochondrial fractions. In human plasma and tissue, the predominant forms of vitamin B12 are methylcobalamin, adenosylcobalamin, and hydroxocobalamin. Bile contains a significant amount of vitamin B12 that is reabsorbed by the small intestine . The importance of this enterohepatic circulation in the maintenance of the vitamin B12 pool is indicated by the observation that patients with vitamin B12 malabsorption become deficient in only 2–3 years compared with the 10–20 years needed for deficiency to develop in individuals who lack dietary vitamin B12, but normally conserve biliary cobalamins.
Vitamin B12 serves as a coenzyme for two important enzymatic reactions. Methylcobalamin is required for the conversion of homocysteine to methionine (Fig. 9.4). This reaction is catalyzed by the cytoplasmic enzyme 5–methyltetrahydrofolate–homocysteine methyltransferase, which utilizes 5-methyltetrahydrofolate as a methyl donor. This pathway is therefore important to maintain the supply of both methionine and tetrahydrofolate. Tetrahydrofolate is subsequently converted to 5,10-methylenetetrahydrofolate, which donates its one-carbon unit to deoxyuridylate, forming thymidylate and contributing to DNA synthesis. Adenosylcobalamin is required for the isomerase reaction in mitochondria that converts methylmalonyl coenzyme A (CoA) to succinyl CoA (Fig. 9.5). Methylmalonyl CoA is derived from propionate and amino acids such as valine , isoleucine , and threonine . In vitamin B 12 deficiency, propionate and methylmalonate accumulate and result in impaired fatty acid synthesis.
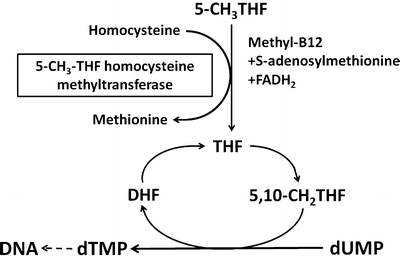
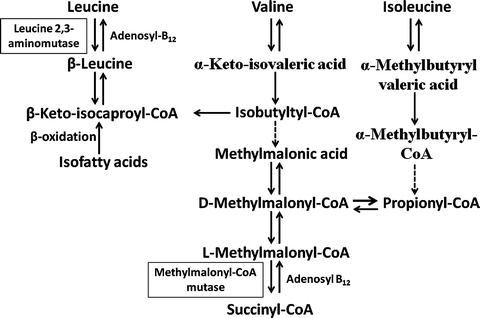
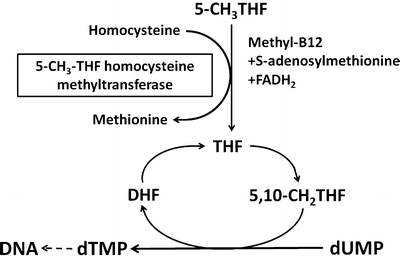
Fig. 9.4
Role of methylcobalamin in the conversion of homocysteine to methione. 5-CH 3 THF 5-methyltetrahydrofolate, THF tetrahydrofolate, CH 2 THF methylenetetrahydrofolate, DHF dihydrofolate, dUMP uridylate, dTMP thymidylate (Adapted from Kano et al. [5])
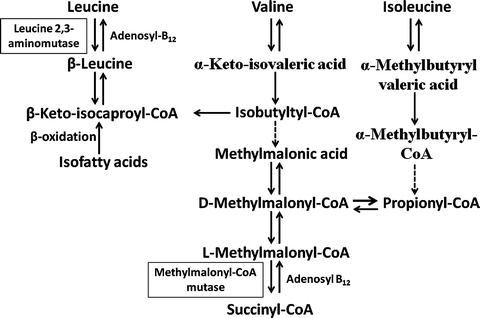
Fig. 9.5
Function of adenosylcobalamin in the mitochondrial isomerase reaction that converts methylmalonyl CoA to succinyl CoA (Adapted from Kano et al. [5])
The major clinical manifestations of vitamin B12 deficiency are hematologic and neuropsychiatric abnormalities. The hematologic changes are identical to those seen in folate deficiency (see above) and are thought to be due impaired generation of tetrahydrofolate and altered DNA synthesis. The neuropsychiatric abnormalities may be a consequence of alterations in brain and peripheral nerve fatty acid synthesis due to deficient methylmalonyl-CoA mutase activity, but disordered folate and methionine metabolism may also play important roles.
3.2 Intraluminal Events in Vitamin B12 Absorption
In the diet, vitamin B12 is predominately protein-bound, either to transport proteins or to the enzyme systems described above. Acid and pepsin play important roles in the digestion of these proteins and in the release of vitamin B12 into the gastric fluid. Individuals with reduced gastric acid and pepsin secretion are often able to absorb pure crystalline vitamin B12 normally, but have impaired absorption of vitamin B12 contained in food.
Gastric juice contains two important vitamin B 12 binding proteins, haptocorrin (R protein–type binder) and intrinsic factor (IF). Haptocorrin is a 60–66-kd glycoprotein present in many digestive secretions, although the haptocorrin in gastric juice is mainly derived from the salivary gland. Vitamin B12 has a much higher affinity for haptocorrin than for IF, particularly at low pH. The vitamin B12 liberated from food therefore binds preferentially to haptocorrin in gastric juice. In addition, bile contains a substantial amount of vitamin B12 bound to haptocorrin. Both haptocorrin and IF are unaffected by acid-peptic digestion. In contrast, pancreatic proteases do not alter IF, but modify haptocorrin to a smaller molecular weight form that has a markedly decreased affinity for vitamin B12. In the small intestinal fluid, therefore, vitamin B12 is rapidly and essentially completely transferred from haptocorrin to IF (Fig. 9.6).
< div class='tao-gold-member'>
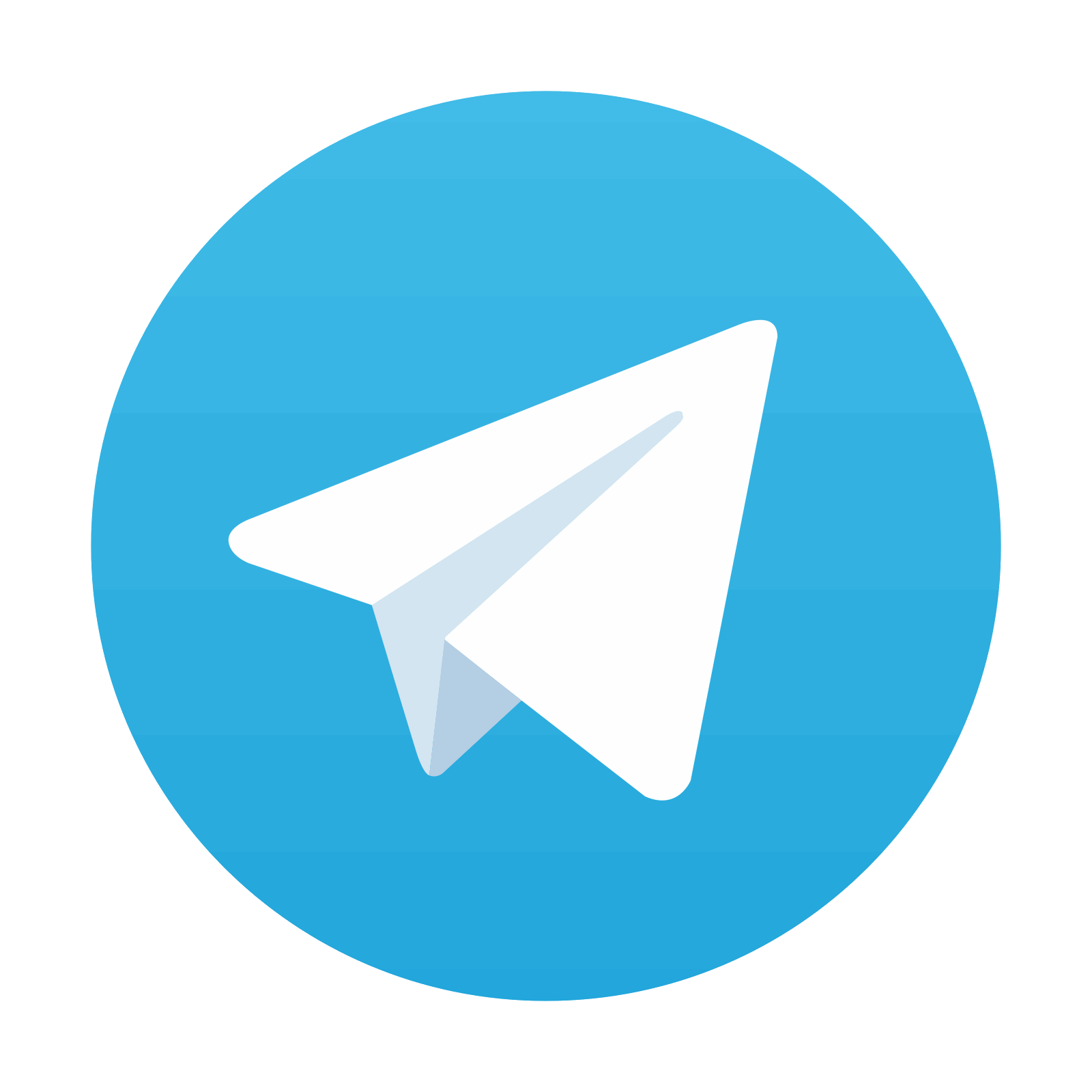
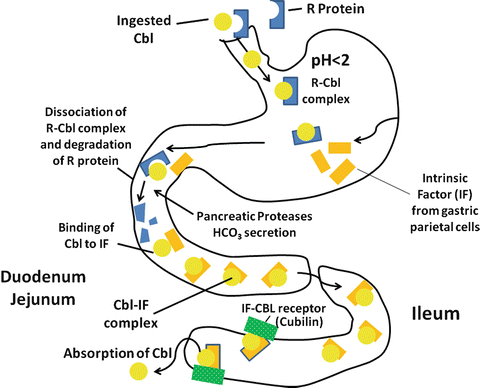
Only gold members can continue reading. Log In or Register a > to continue
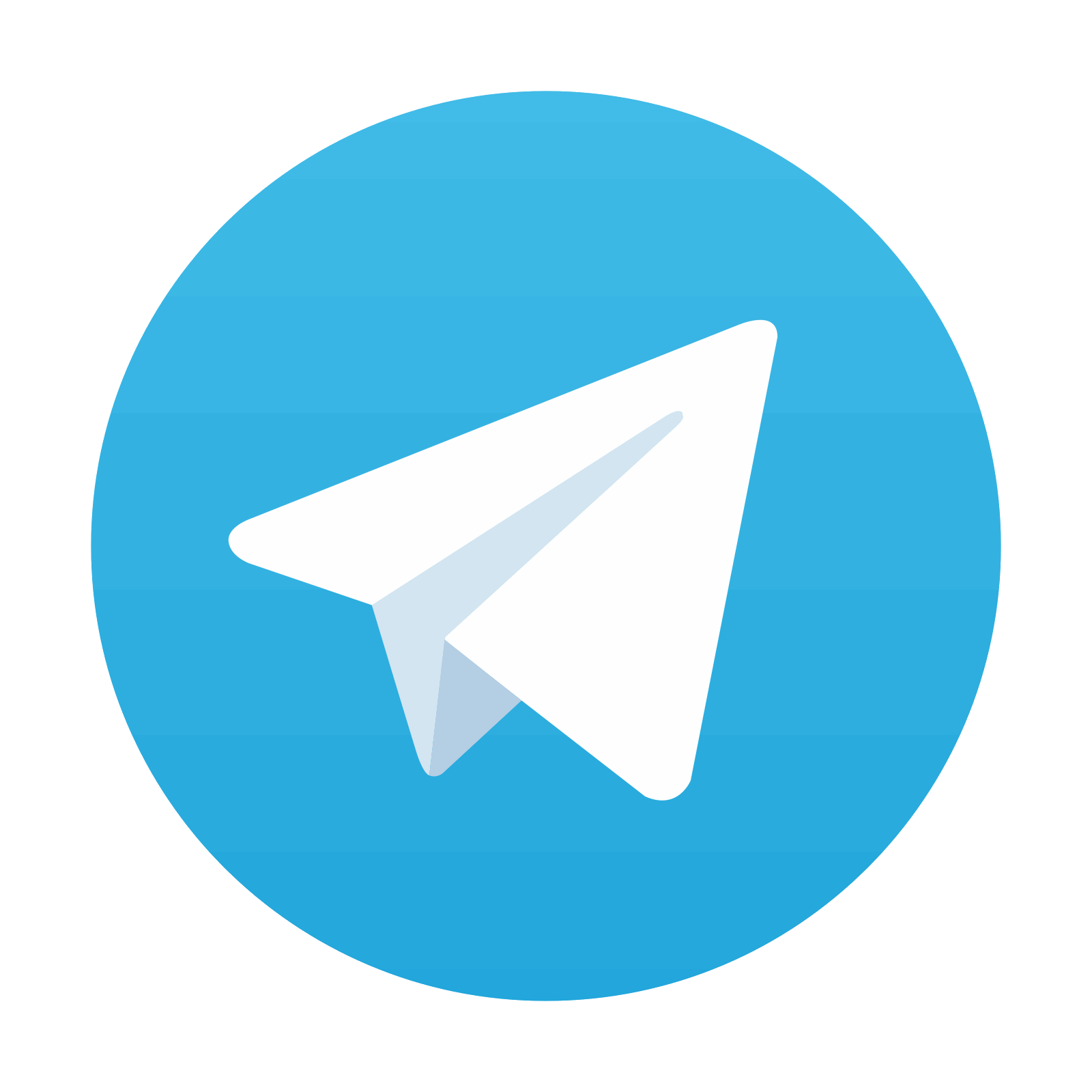
Stay updated, free articles. Join our Telegram channel

Full access? Get Clinical Tree
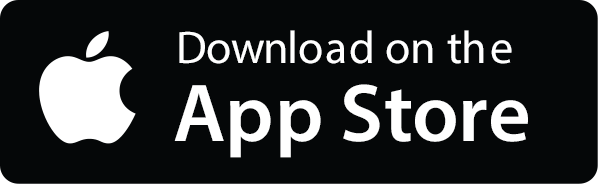
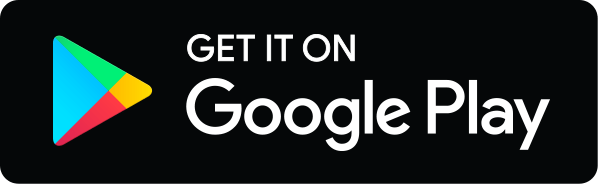