Diffusion across a semipermeable membrane. The driving force for solute diffusion is the transmembrane concentration gradient. Small solutes with higher concentrations in the blood compartment, such as potassium, urea, and small uremic toxins, diffuse through the membrane into the dialysate compartment. Dialysis dissipates this concentration gradient (i.e., the molecular concentration gradient decreases with dialysis). Larger solutes and low-molecular-weight proteins such as albumin diffuse poorly across the semipermeable membrane
Convection: The spontaneous transport of solutes across the dialysis membrane is called convection. Convection is mainly responsible for scavenging macromolecules. Factors affecting convection include the screening coefficient of dialysis membrane and membrane pore size, the size and configuration of solute molecules, and the charge of membrane and solute.
Adsorption: Adsorption is a method for removing molecules from the blood or plasma by attachment to a surface incorporated in a module within an extracorporeal circuit. Adsorption occurs fundamentally because of the hydrophobic properties of sorbents. In this group, the sorbents used in different dialysis techniques are charcoal and nonionic macroporous resins. Adsorption occurs by chemical affinity, such as ion-exchange resins and chemisorbents.

Ultrafiltration across a semipermeable membrane. The driving force for ultrafiltration is the transmembrane hydrostatic pressure. When applied to the blood compartment, solvent flows across the membrane into the dialysate compartment, bringing along solutes
17.3 Dialysis System
The purpose of HD is to effectively remove uremic toxins and extra fluid from the blood. The dialysis systems are mainly composed by the dialyzer, dialysis machine, extracorporeal blood circuit, and water purification system [3]. The dialysis machine has a blood and dialysate pumps, dialysate mixing and degassing unit, heating system, UF balancing system, as well as monitoring and safety system. Contaminants must be purified from the water for dialysis prior to its use. The final dialysate is produce by the proportioning water purification system.
17.3.1 Dialyzer

Membrane fluxes in dialysis. Dialysis is the process of separating elements in a solution by diffusion across a semipermeable membrane (diffusive solute transport) down a concentration gradient. This is the principal process for removing the end-products of nitrogen metabolism (urea, creatinine, and uric acid) and for repletion of the bicarbonate deficit of the metabolic acidosis associated with renal failure in humans. The preponderance of diffusion as the result of gradient is shown by the displacement of the arrow
17.3.2 Dialysis Membrane
Examples of dialyzer
Material (abbreviation) | Membrane type |
---|---|
Cellulose triacetate (CTA) | Hollow fiber |
Polysulfone (PS) | Hollow fiber |
Polyethersulfone (PES) | Hollow fiber |
Polymethylmethacrylate (PMMA) | Hollow fiber |
Polyester polymer alloy (PEPA) | Hollow fiber |
Ethylene vinyl alcohol copolymer (EVAL) | Hollow fiber |
Polyacrylonitrile (PAN) | Hollow fiber, laminated |
- 1.
Biocompatibility of membrane: The biocompatibility of synthetic membrane is superior to that of fiber membrane. After contact with the blood, the dialysis membrane may produce various reactions, including the activation of complement, slow excitation peptide, white blood cells, platelets, and blood coagulation factor. Membranes with better biocompatibility produce less reaction. Improvement in biocompatibility can reduce the deposition of amyloidosis, which can be characterized by joint disease, bone lesions and pathological fracture, soft tissue swelling, and carpal tunnel syndrome. In addition, it can reduce allergic reactions, reduce the occurrence of dialysis hypotension, improve nutrition, preserve residual renal function, reduce the incidence of cardiovascular disease, and reduce mortality.
- 2.
Permeability of dialysis membrane: Permeability of water is expressed as UF coefficient Kuf, which refers to the UF of unit pressure in unit time. According to Kuf, dialyzers can be divided into three groups: low-flux dialyzer (Kuf <6 mL/mmHg·h) is used in ordinary dialysis; Kuf 7–20 mL/mmHg·h is in flux, the parser that can be used for efficient dialysis; and high-flux dialyzer (Kuf >20 mL/mmHg·h) is used for the removal of water and macromolecules. It is suitable for high-flux HD and hemofiltration (HF).
- 3.
Dialysis membrane solute sieving coefficient: The ability of the dialysis membrane to filter the solute in the convection is related to the molecular weight of the solute.
17.3.3 Water Treatment and Dialysate
Association for the Advancement of Medical Instrumentation water quality standard for dialysis
Substance | Maximum allowable concentration (mg/L) |
---|---|
Aluminum | 0.01 |
Chloramines | 0.1 |
Copper | 0.1 |
Fluoride | 0.2 |
Nitrate | 2.0 |
Sulfate | 100.0 |
Zinc | 0.1 |
Arsenic | 0.005 |
Barium | 0.1 |
Cadmium | 0.001 |
Chromium | 0.014 |
Lead | 0.005 |
Mercury | 0.0002 |
Selenium | 0.09 |
Silver | 0.005 |
Calcium | 2.0 (0.1 mEq/L) |
Magnesium | 4.0a (0.3 mEq/L) |
Potassium | 8.0a (0.2 mEq/L) |
Sodium | 70.0a (3.0 mEq/L) |
Antimony | 0.003 |
Free chlorine | 0.50 |
Thallium | 0.002 |
The dialysate carries away the waste materials and fluid removed from the blood by the dialysis procedure, prevents the removal of essential electrolytes, normalizes electrolyte levels, and averts excess water removal during the procedure. Furthermore, the dialysate corrects the acid–base balance in the patient. These functions are achieved by modulating the chemical composition in the dialysate close to that in normal blood.
Solute concentrations present in the dialysate
Solutes | Concentration (mEq/L) |
---|---|
Sodium | 135–145 |
Chloride | 102–106 |
Bicarbonate | 30–39 |
Dextrose | 11 |
Acetate | 2–4 |
Magnesium | 0.5–1 |
Potassium | 0–4 |
Calcium | 0–3.5 |
pH | 7.1–7.3 |
17.4 HD Techniques
Here, HD techniques refer to common blood purification techniques, which include HD, HF, and hemoperfusion (HP) [4–6].
17.4.1 Hemodialysis
HD removes solutes by diffusion based on the concentration gradients of solutes between the blood and dialysate across the semipermeable membrane. For example, urea diffuses from the blood to the dialysate compartment, thereby decreasing the total urea mass in the body and the urea concentration in the plasma. Conversely, the concentration gradient of bicarbonate usually favors diffusion of this ion from the dialysate to the blood compartment. Movement of water-carrying solutes across the dialysis membrane is not necessary for solute transport in this modality, although removal of fluid from the patient’s plasma is often desirable because dialysis patients are usually fluid-overloaded. High efficiency in HD refers to a high rate of removal by diffusion of small-sized solutes; high flux in HD refers to a high rate of removal by diffusion of “middle molecules” that are substantially larger than urea. A membrane can be a high-efficiency/high-flux, high-efficiency/low-flux, low-efficiency/high-flux, or low-efficiency/low-flux membrane. The term “conventional dialysis membrane” usually refers to a low-efficiency/low-flux membrane.
17.4.2 Hemofiltration
HF, another form of extracorporeal therapy, removes fluid by convection (i.e., water movement across the large-pore HF membrane into the ultrafiltrate compartment drags along the solutes dissolved in the water). A crucial distinction between HD and HF is that fluid removal, but not the concentration gradient of the solute, is required for solute removal in HF. Removal of fluid with its accompanying solutes results in a loss of the total body mass of the solute, but not necessarily a decrease in the plasma concentration. In order to achieve a substantial decrease in concentration, “clean” replacement fluid devoid of that solute is intravenously infused to replace nearly the large volume of plasma fluid removed in the hemofilter. This modality is analogous to glomerular filtration, in which plasma solutes are removed by convection. In the case of the glomerulus, however, the replacement fluid is water and electrolytes that are selectively reabsorbed from the renal tubules. The term hemodiafiltration (HDF) refers to the combination of HD and HF operating simultaneously using a large-pore membrane (i.e., solutes are removed by both diffusion and convection).
When HD, HF, and HDF are continuously applied for days to weeks in the presence of acute kidney injury (AKI), they are referred to as continuous renal replacement therapy (CRRT). The terms are further qualified by the forms of vascular access used. For example, continuous HF using an artery for blood supply and a vein for blood return in the extracorporeal circuit is called continuous arteriovenous hemofiltration. Continuous HDF exclusively using veins for vascular access is called continuous venovenous HDF. A rather common form of CRRT is slow (or sustained) low-efficiency HD.
17.4.3 Hemoperfusion
HP is the removal of solutes (usually toxins) from the blood by adsorption onto materials, such as charcoal or resins, in the extracorporeal circuit. HP is primarily used as treatment for acute poisoning. Adsorbents designed to remove specific molecules, such as β2-microglobulin, are not used in routine clinical renal replacement therapies (RRTs).
17.5 Acute Complications of HD
17.5.1 Neurological Complications
17.5.1.1 Disequilibrium Syndrome
Disequilibrium syndrome is a situation that produces neurological and other symptoms soon after a patient begins dialysis treatment. Urea is able to move freely between the cells and serum. Theories suggest that when a severely uremic patient is dialyzed for the first time, as the urea is removed, the plasma becomes more hypotonic, causing water to shift from the plasma into the brain tissue, which is less hypotonic and contains higher amounts of urea. Patients who (1) are treated with HD, (2) have very high blood urea nitrogen levels (usually >60 mmol/L), (3) have severe acidosis, (4) are elderly individuals or children, and (5) have a history of neurological diseases are at risk of disequilibrium syndrome. As the water flows to higher urea concentration, the brain cells begin to swell, causing neurological symptoms that range from headache, nausea, vomiting, restlessness, and twitching to the more severe tremors, disorientation, and convulsions. Treatment includes the administration of a hypertonic solution, such as hypertonic saline, 50% dextrose, or mannitol. The patients’ symptoms should be treated. Delivering a less effective treatment using lower blood and dialysate flow rates, decreasing treatment time, or running the patient with a concurrent flow will help minimize these symptoms until the blood urea nitrogen levels stabilize [7, 8].
17.5.1.2 Muscle Cramps
Persistent involuntary skeletal muscle contractions with pain is a common complication during dialysis. It usually occurs near the end of dialysis, may be associated with low blood pressure, and is responsive to treatment with plasma volume expanders. However, cramps do not always rely on significant volume reduction and hypotension and have a positive response to hypertonic saline, mannitol, and hypertonic glucose solutions, which may suggest a role for low osmotic pressure in the pathogenesis of muscle contraction. Muscle cramps is often associated with peripheral vascular disease and the management for symptom includes the following aspects which are aimed at increasing plasma osmotic pressure. It has been shown that parenteral infusion of 25% mannitol (50–100 mL), 23.5% hypertonic saline (15–20 mL), or 50% hypertonic glucose solutions (25–50 mL) is equally effective. Applied before hemodialysis, midodrine hydrochloride tablet may be effective in reducing muscle contraction in patients with symptomatic hypotension during dialysis. Precautions include reducing excessive weight gain between dialysis to avoid rapid UF. Increasing the dry weight may be suggested if interdialytic weight gain is appropriate. It has been found that the use of quinine sulfate (oral, 325 mg) at the onset of HD may significantly reduce the incidence of muscle contraction. Using different sodium modeling strategies (exponential or stepwise), for example, starting from a dialysate sodium concentration of 145–155 mEq/L and linearly decreasing to 135–140 mEq/L, has led to similar clinical results. The use of internal blood volume UF feedback control system is associated with a lower incidence of muscle contraction. Finally, stretching exercises for affected muscle groups during dialysis may be beneficial [9–11].
17.5.1.3 Convulsion
Convulsion occurs in <10% of patients and more frequently in patients starting HD. Cerebral hemorrhage should be considered when focal neurological signs are positive. Other reasons include hypertensive encephalopathy, disequilibrium syndrome, uremic encephalopathy, acute aluminum poisoning, hypoglycemia, and alcohol withdrawal. Termination of HD should be immediately performed when convulsion occurs, the respiratory tract should be kept unblocked, and the circulation should be maintained stable. Electrolyte and blood glucose levels should be immediately measured, and sedatives should be administrated to stop convulsion. Subsequent treatment needs to be decided according to the process of attack, signs, and results of blood tests.
17.5.2 Allergic Reactions
17.5.2.1 Dialyzer Reactions
Dialyzer reactions are sometimes referred to as “first-use syndrome” because some patients, upon exposure to the dialyzer membrane for the first time, develop allergic-type symptoms. Dialyzer reactions are at present more commonly referred to as type A and B reactions, with type A reactions being more severe and often presenting with anaphylactic-type symptoms. These reactions usually occur within the first 5 min of treatment, with patients experiencing the following symptoms: dyspnea, chest and back pain, feeling of warmth, sense of impending doom, and cardiac arrest. Less threatening symptoms include itching, urticaria, coughing, sneezing, watery eyes, and abdominal cramping. The causes are probably multifactorial and may involve the activation of plasma proteins by dialysis membranes, allergy to disinfectants, or release of noxious substances that have contaminated the dialyzers during the manufacturing or sterilization process. Another cause is the accumulation of vasoactive kinins as a result of enhanced activation of kininogen by dialysis membranes made of copolymers of acrylonitrile and methallyl sulfonate and decreased kinin degradation due to the simultaneous administration of angiotensin-converting enzyme inhibitors, which are also kininase inhibitors. Type A reactions are usually due to the factory sterilant ETO. This type of reaction is currently less common because some dialyzer manufacturers are using alternative sterilization methods such as gamma irradiation as a sterilant, e-beam sterilization, or steam sterilization. For those using dialyzers sterilized with ETO, proper priming of the dialyzer may help prevent pockets of ETO from remaining in the fibers to be released during the patient’s dialysis treatment. Type B reactions are less threatening but more commonly seen. The symptoms usually occur as soon as the patient’s blood is exposed to the dialyzer and returned to the patient. Symptoms include chest pain, hypotension, and occasionally back pain. The treatment for both types of reactions is based on symptoms. Dialysis treatment should be discontinued until the cause of symptoms is determined and the physician is notified. Oxygen is generally administered for difficulty in breathing. Intravenous antihistamines or epinephrine may be ordered for anaphylaxis. Blood pressure support may also be necessary for hypotension [12].
17.5.2.2 Reuse Syndromes
The reuse reaction is more likely due to other agents such as germicides for re-treatment of dialyzers because most of the remaining ETO is washed out of the dialyzer at “first use.” Commonly used germicides include glutaraldehyde, peracetic acid/hydrogen peroxide, and formaldehyde. Formaldehyde is a known allergen and a life-threatening response is observed in HD patients who are positive for the radioactive sorbent formaldehyde test. Disinfection of the water supply system may also result in exposure to residual formaldehyde.
17.5.2.3 Drug-Induced Reactions
Intravenous Iron Dextran
Iron dextran is a mixture of synthetic glucose polymers that is involved in systemic reactions. The allergic reaction to iron dextran is due to this compound, which occurs at 0.6–1% of the recipients. Clinical practice guidelines of the National Kidney Foundation’s recommend that resuscitation equipment and healthcare personnel should be available whenever iron dextran is used. High and low molecular weight dextran iron preparations differ in the frequency of allergic reactions, which latter appear to be safer. The exact mechanism of dextran-induced allergic reactions is unclear, but dose-dependent histamine releases from basophils, which may be the cause of cardiovascular failure, seems to occur. Due to this dose-related toxicity, 0.5–1 mg of iron dextran should always be used as a starting test dose so that staff could deal with the reaction in time. If the test dose is safe, a course of treatment with ten doses of 100–200 mg per session of dialysis can be safely provided. An alternative to iron dextran including intravenous administration of iron gluconate and saccharin may cause less allergic reactions, but it has been recognized that free iron released from these drugs can lead to inflammatory reactions.
Heparin
Patients are rarely highly sensitive to heparin preparations, and allergic reaction usually occurs only when beef heparin and porcine heparin are exchanged.
Desferrioxamine
Desferrioxamine or iron chelation in the treatment of aluminum poisoning can lead to hypotension during dialysis, hearing toxicity, bone pain, gastrointestinal disorders, visual loss, or even rare allergic reactions or aggravation of aluminum encephalopathy.
17.5.2.4 Intradialytic Hypotension
Hypotension during dialysis requiring drug intervention occurs in 10–30% of treatments. Although intradialytic hypotension can often be asymptomatic, it may be accompanied by a severe decrease in blood perfusion in vital organ, leading to loss of consciousness, seizures, and even death. There are a lot of reasons for intradialytic hypotension. First, blood volume may decrease excessively. Excessive weight gain between two dialysis sessions or inappropriate dry weight may lead to plasma refilling failure during dialysis, which is a crucial reason that accounts for hypotension. The rapid clearance of uremic toxins results in a low osmotic pressure of interstitial fluid and blood leading to the shift of water into the cells and eventually causes a decrease in blood volume. Second, there may be a decrease in vascular tone. The inability of the body to make a normal compensatory response to hypovolemia, characterized by a central redistribution of blood volume and an increase in peripheral vascular resistance, is a common mechanism of hypotension. Several patient-related factors are involved in the mechanism of the failed compensatory response, which include arrhythmia, taking antihypertensive drugs, structural heart disease, bleeding, anemia, autonomic neurological dysfunction (especially in patients with diabetes and elderly), food intake during dialysis, venous stasis during dialysis, fervescence, and sepsis. Reduced sensitivity to renin-angiotensin, adrenergic, and arginine vasopressin systems may also result in insufficient vasoactive response to hypovolemia induced by HD. Third, a disturbance in cardiac constructive and dilated function can also contribute to intradialytic hypotension.
Strategies for intradialytic hypotension
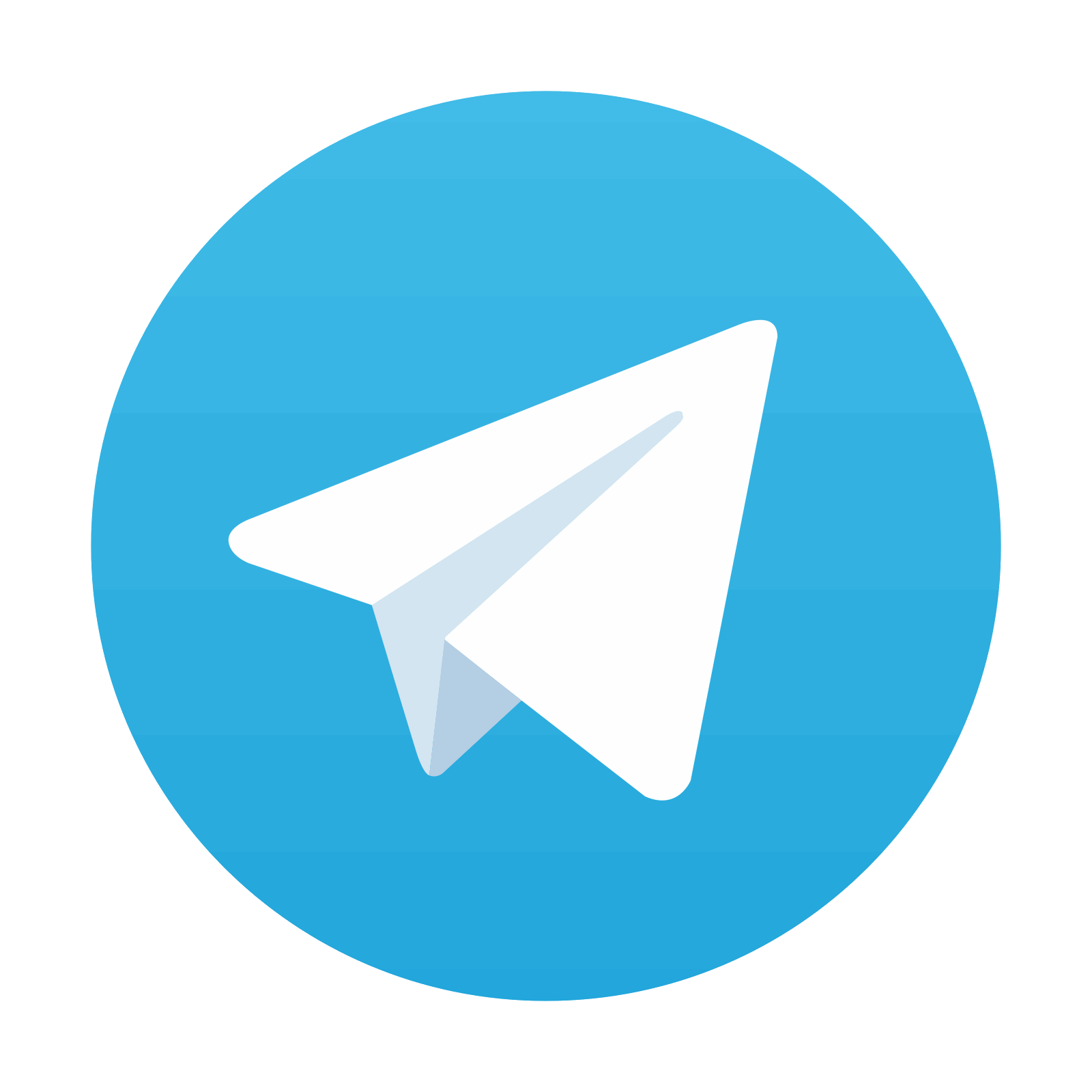
Stay updated, free articles. Join our Telegram channel

Full access? Get Clinical Tree
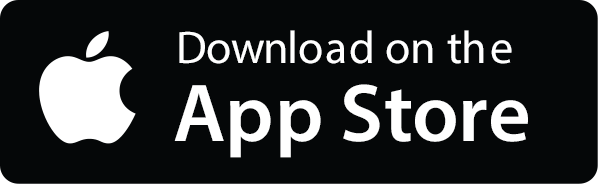
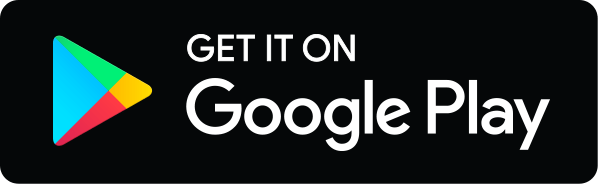