Chapter 2 Ovarian Hormones: Structure, Biosynthesis, Function, Mechanism of Action, and Laboratory Diagnosis
INTRODUCTION
The main function of the ovaries, maturation and release of oocytes, is accomplished via the production of several steroidal and nonsteroidal hormones that locally modulate a series of complex events. Peripherally, these hormones act on various target organs, including the uterus, vagina, fallopian tubes, mammary glands, adipose tissue, bones, kidneys, and liver, leading to the female phenotype.
The Ovary as an Endocrine Organ
The two-cell theory describes the sequence of events that occurs during ovarian follicular growth and steroidogenesis. According to this theory, LH primarily stimulates thecal cells to produce androstenedione and testosterone, both 19-carbon steroids. In contrast, FSH primarily stimulates granulosa cells to aromatize these 19-carbon steroids into estrogens.1,2
The ovarian production of steroid hormones is regulated both within the ovary, by paracrine (intercellular) and autocrine (intracellular) mechanisms, and by endocrine regulation of FSH secretion by the pituitary. Central to this regulation are several nonsteroidal hormones and factors produced by the ovary.3 This chapter focuses on these aspects of the ovary and discusses the biochemistry, biosynthesis, regulation, and actions of both steroidal and peptide ovarian hormones.
STEROIDOGENESIS AND STEROID HORMONES OF THE OVARY
Steroidogenesis
Steroid hormone formation in the steroid-producing endocrine glands follows the same fundamental pathway and mainly relies on exogenous (or plasma) cholesterol, with the exception of the liver and intestinal mucosa, which are capable of synthesizing cholesterol endogenously from acetyl-coA. The primary source of cholesterol for steroidogenesis in the ovary is derived from the uptake of plasma LDL.4 The rate-limiting step in steroidogenesis is transfer of cholesterol from the cytosol to the inner membrane of the mitochondria.5 This is mediated by an LH-induced mitochondrial enzyme called steroidogenic acute regulatory (StAR) protein.6 The StAR gene is located on chromosome 8p11.2 and codes for a 285-amino acid precursor protein, of which 25 amino acids are cleaved off after transport to the mitochondria.7,8 Nonsense mutations of the StAR gene that result in premature stop codons have been identified as a cause of congenital lipoid adrenal hyperplasia, which is characterized by the presence of intracellular lipid deposits that destroy steroidogenesis.7
Ovary steroid hormones are synthesized in both interstitial and follicular cells. The basic structure of cholesterol is three hexagonal carbon rings and a pentagonal carbon ring to which a side chain is attached (Fig. 2-1). Two important methyl groups are also attached at positions 18 and 19. Progestins and corticosteroids (pregnane series 21-carbon steroids) are produced by partial cleavage of the side chain (i.e., the desmolase reaction). Androgens (androstane series 19-carbon steroids) are produced by the total removal of the side chain. Estrogens (estrane series 18-carbon steroids) are produced by aromatization of one of the three hexagonal carbon rings to a phenolic structure with loss of the 19-methyl group.
The first step in steroidogenesis is the conversion of cholesterol to pregnenolone via hydroxylation at the carbon 20 and 22 positions, which is followed by cleavage of the side chain (Fig. 2-2). From pregnenolone, steroid hormones are produced by one of two general pathways.5 The pregnenolone (Δ5) pathway produces androgens and estrogens (pregnenolone→17OH-pregnenolone →dehydroepiandrosterone [DHEA]→testosterone→estrogen). The progesterone (Δ4) pathway produces androgens and estrogens (pregnenolone→progesterone→17OH-progesterone→androgen →estrogen). In the adrenal gland, the Δ4 pathway produces mineralocorticoids and glucocorticoids.
The enzymes involved in the intracellular synthesis of steroid hormones include five hydroxylases, two dehydrogenases, a reductase, and an aromatase. The hydroxylases and aromatase belong to the cytochrome P450 (CYP) supergene family (Table 2-1). These enzymes exist on both the mitochondria and endoplasmic reticulum.
Table 2-1 Enzyme Reaction and Cellular Location of Steroidogenic Enzymes
Enzyme Reaction | Gene (Enzyme) | Cellular Location/Tissue Location |
---|---|---|
Cholesterol side chain cleavage | CYP11A (P450scc) | Mitochondria (theca; granulosa) |
17α-hydroxylase | CYP17 (P450c17) | ER (theca) |
17,20-hydroxylase (lyase) | CYP17 (P450c17) | ER (theca) |
Aromatase | CYP19 (P450arom) | ER (granulosa) |
3β-hydroxysteroid dehydrogenase | 3βHSD | ER (theca; granulosa) |
17β-hydroxysteroid dehydrogenase | 17βHSD | ER (granulosa) |
21-hydroxylase | CYP21 (P450c21) | ER (adrenal) |
11β-hydroxylase | CYP11B1 (P450c11) | Mitochondria (adrenal) |
ER, endoplasmic reticulum
Of these nine enzymes, four key enzymes regulate the main steps of steroidogenesis (see Fig. 2-2): CYP11A (P450scc), a side chain cleavage enzyme that catalyzes the conversion of cholesterol to pregnenolone; 3βHSD, or 3 βa-hydroxysteroid dehydrogenase, which converts pregnenolone to progesterone; CYP17 (P450c17), an hydroxylase that converts pregnenolone to androgens; and CYP19 (P450arom), an aromatase that converts androgens to estrogens. Most reactions are irreversible (denoted by a single arrow in Fig. 2-2). The few reversible reactions (denoted by double arrows) are dependent on cofactor availability (e.g., NADP/NADPH ratio).
The kind of hormone produced depends on the nature of the cell and the presence or absence of the inherent steroidogenic enzymes in the tissue. The adrenal cortex lacks 17βHSD; hence, adrenal androgen production is limited to DHEA and androstenedione. In the testes, LH controls 17βHSD activity and testosterone production. The steroid-producing cells of the ovary (granulosa, theca, corpus luteum) contain the full enzymatic complement for steroid hormone synthesis. In the thecal cells, LH also controls 17βHSD activity and androstenedione production, whereas CYP19 (P450arom) activity in the granulosa cells is controlled by FSH and hence estradiol production. These relationships are the basis for the two-cell, two-gonadotropin system (Fig. 2-3). Aromatization occurs in the endoplasmic reticulum.
In each of the two cell types, the amount of the various enzymes differs depending on the stage of follicle development. CYP11A (P450scc) and 3βHSD are expressed in both thecal and granulosa cells of antral and preovulatory follicles and in the luteinized granulosa and thecal cells of the corpus luteum. In contrast, CYP17 (P450c17) is expressed only in the thecal cells of antral and preovulatory follicles and of the corpus luteum (see Fig. 2-3).
Steroid Hormones of the Ovary
Estrogens
Physiologic Role
Estrogens are essential in the development and maintenance ofthe female phenotype, germ cell maturation, and pregnancy. In addition to their reproductive effects, estrogens also have many other nonreproductive systemic effects, such as bone metabolism/remodeling, nervous system maturation, and endothelial responsiveness.9
At puberty, estrogen stimulates breast development and enlargement and maturation of the uterus, ovaries, and vagina.10,11 Estrogen also works in concert with growth hormone and insulin-like growth factor I (IGF-I) to produce a growth spurt and stimulates the maturation of chondrocytes and osteoblasts, which ultimately leads to epiphyseal fusion.12,13 After midpuberty, estrogen begins to exert a positive feedback on gonadotropin-releasing hormone (GnRH) secretion, leading to the progressive increase of LH and FSH production, culminating in the LH surge, ovulation, and the initiation of the menstrual cycle.
In the adult female, estrogen plays a critical role in maintaining the menstrual cycle.14 The cyclical changes in estradiol, progesterone, and pituitary hormones are illustrated in Figure 2-4. In the early follicular phase of the menstrual cycle, FSH stimulates granulosa cell aromatase activity, resulting in increased follicular concentrations of estrogen. The rising estrogen level further increases the sensitivity of the follicle to FSH and estrogen by increasing the number of estradiol receptors on the granulosa cells. Follicular growth and antral formation is also promoted by estrogen. This sets up a positive feedback cycle, which culminates in one dominant follicle producing an exponential rise in estrogen levels. This exerts a negative feedback on FSH so that falling FSH levels contribute to atresia of other nondominant follicles. The dominant follicle secretes large quantities of estrogen; estradiol levels must be greater than 200 pg/mL for approximately 50 hours before a positive feedback on LH release is achieved.13,15 Once the LH surge is initiated, luteinization of the granulosa cells and progesterone production occurs. In pregnancy, estrogen augments uterine blood flow, although it is not required in itself for the maintenance of pregnancy.16
In the central nervous system, estrogen withdrawal at menopause has been associated with reduced libido, altered mood, and cognitive disturbances. These effects have been attributed to estrogen’s ability to modulate the synthesis, release, and metabolism of many neuropeptides and neurotransmitters.17 Estrogen acts as a serotoninergic agonist by increasing serotonin synthesis in the brain, which may positively influence mood.18 Although prospective observational studies in postmenopausal women have suggested that estrogen replacement therapy might protect against cognitive decline19 and the development of dementia,20 randomized trials of estrogen in the treatment of Alzheimer’s disease have shown no evidence of benefit.21–24
In the skeletal system, estrogen antagonizes the effect of parathyroid hormone by directly inhibiting the function of osteoclasts, which decreases the rate of bone resorption and diminishes bone loss. The Postmenopausal Estrogen/Progestin Interventions (PEPI) trial was a prospective, placebo-controlled trial designed to study the effects of hormone replacement on bone density in postmenopausal women. After 12 months of treatment with estrogen, bone mineral density increased by 1.8% at the hip and by 3% to 5% at the spine.25 The Women’s Health Initiative (WHI) showed that estrogen reduced the risk of both hip and vertebral fractures by 30% to 39%.26
In the cardiovascular system, there is strong evidence that estrogen has a natural vasoprotective role. At a cellular level, estrogen receptors are found on the smooth muscle cells of coronary arteries27 and the endothelial cells of various sites.28 Estrogen causes short-term vasodilation by increasing nitric oxide and prostacyclin release in endothelial cells.29 Several large observational studies, including the Framingham study and the Nurses Health Study, have shown that cardiovascular incidence rates are lower in premenopausal than postmenopausal women.30 There was also a significant association between a younger age at menopause and a higher risk of coronary artery disease.31 These studies led to the conviction that estrogen replacement therapy would consequently prevent the progression of atherosclerosis and coronary heart disease. However, the WHI study and the Heart and Estrogen/progestin Replacement Study (HERS), both large randomized, prospective trials designed to specifically address this issue, have not shown any benefit of estrogen for either the primary or secondary prevention of coronary artery disease, respectively.26,32
Biosynthesis and Metabolism
Estrogens are 18-carbon steroids derived from cholesterol (see Fig. 2-1). The three forms of naturally occurring estrogen include estrone, 17β-estradiol, and estriol. In nonpregnant females, estrone and estradiol are the main biologically active estrogens secreted by the ovary. Estradiol is almost 2 to 5 times more potent than estrone.33 The circulating levels of estradiol are 2 to 4 times higher than those of estrone in premenopausal women. Estradiol concentrations in postmenopausal women are one tenth of those in premenopausal women. Estrone concentrations do not differ with menopausal status; thus, over time, the premenopausal estradiol-to-estrone ratio is reversed.34 In contrast, estriol is not the secretory product of the ovary but is the peripheral metabolite of estrone and estradiol.
The main estrogen in premenopausal women is estradiol, which is produced primarily by the granulosa cells of the ovary. Androstenedione is converted to testosterone via 17βHSD, which is rapidly demethylated at the C-19 position and aromatized to estradiol. Estradiol is also generated to some degree from androstenedione via estrone. Estrone is also a secreted product of the ovary. It constitutes the remaining circulating estrogen (40%) and is mainly derived from the extragonadal peripheral aromatization of adrenal androstenedione.35 Peripheral conversion of androgens to estrogens occurs in skin, muscle, and adipose tissue and in the endometrium.36
In the normal adult female, the production of estradiol varies according to the phase of the menstrual cycle. During the mid luteal phase, for example, the production rate is about 100 to 270 μg/day. In comparison, the production rate for androstenedione is about 3 mg/day, and with its peripheral conversion rate to estrone of about 1.5%, it accounts roughly for about 10% to 30% of estrone production per day. Secondary increases in estrone formation occur in patients with polycystic ovaries or with ovarian cancer characterized by increased androgen production. In such patients, the increased estrogen can disturb the menstrual cycle. In postmenopausal women, the ovarian contribution shrinks, leaving estrone, derived from adrenal androstenedione, as the main source of circulating estrogen.37
In the pregnant woman, the placenta becomes the main source of estrogen in the form of estriol. The placenta is unable to synthesize steroids de novo and depends on circulating precursors from both fetal and maternal steroids. Most of the placental estrogens are derived from fetal androgens (e.g., DHEA sulfate), produced by the fetal adrenal gland.38 Fetal DHEA sulfate is converted to free DHEA by placental sulfatase and then to androstenedione and testosterone before being aromatized to estrone and estradiol. Finally, it is hydroxylated to form estriol.
Estradiol is rapidly converted in the liver to estrone by 17βHSD. Estrone can be further metabolized via three pathways. First, it can be hydroxylated to 16α-hydroxyestrone, which is then converted to estriol. Estriol is further metabolized by sulfation and glucuronidation, and the conjugates are excreted into the bile or urine. Secondly, estrone can be conjugated to form estrone sulfate, which occurs primarily in the liver. Estrone sulfate is biologically inactive and is present in concentrations that are 10-fold to 20-fold higher than concentrations of estrone or estradiol.39 Estrone sulfate can be hydrolyzed by sulfatases present in various tissues to estrone and may serve as a reserve of estrogen in an inactive form. Estrone sulfate may be of some importance in assessing estrogenicity in women and can be detected in serum as well as in urine.40 Thirdly, estrone can also be metabolized by hydroxylation to form 2-hydroxyestrone and 4-hydroxyestrone, which are known as catechol estrogens. These are then converted to the 2-methoxy and 4-methoxy compounds by catechol-O-methyltransferase.
Progesterone
Physiologic Role
During pregnancy, progesterone increases insulin resistance in concert with the production of the other placental counterregulatory hormones, including placental growth hormone, placental lactogens, placental corticotropin-releasing hormone, and cortisol.41
Biosynthesis and Metabolism
Progesterone is part of the group of 21-carbon steroids, which also includes pregnenolone and 17OH-progesterone. Progesterone is responsible for all the progestational effects, whereas pregnenolone is the precursor for all steroid hormones. 17OH-progesterone has little biologic activity. Progesterone and 17OH-progesterone are mainly produced by the corpus luteum in the luteal phase of the menstrual cycle and by the placenta if pregnancy occurs. Circulating levels of progesterone at concentrations greater than 4 to 5 ng/mL (12.7 to 15.9 nmol/L) are indicative of ovulation.42
Androgens
Physiologic Role
In women, androgens originate as 19-carbon steroids from the adrenals and ovaries. The major androgens produced in the ovary, primarily by the thecal cells and to a lesser degree by the ovarian stroma, include DHEA, androstenedione, and a small amount of testosterone. Both DHEA and androstenedione serve as precursors to estrogen synthesis and have little, if any, androgenic activity. However, these biologically inactive androgens are converted by extraglandular metabolism to biologically active androgens such as testosterone and dihydrotestosterone (DHT). Normally, the levels of these potent androgens are low in females and have no significant physiologic function. Excessive production of androgens by the ovary or adrenals has been implicated as the cause of hirsutism and virilization in women.43 In contrast, in the male the androgens, of which testosterone and DHT are the most crucial, are of primary importance.
Biosynthesis and Metabolism
Androgens are 19-carbon steroids derived from cholesterol. The rate-limiting step in androgen synthesis is the conversion of cholesterol to pregnenolone, which is mediated by the action of LH on the ovary and testes. In a normal ovulatory woman, the ovaries secrete approximately 1 to 2 mg of androstenedione, 1 mg of DHEA, and approximately 0.1 mg of testosterone. The majority (≈0.2 mg) of circulating testosterone is derived from peripheral metabolism of DHEA and androstenedione. Overall, testosterone production in women is about 0.3 mg/day; roughly 50% of this is derived from peripheral conversion whereas the remaining 50% is secreted equally by the ovary and the adrenals.44
Transport of Ovarian Steroid Hormones in Plasma
Steroid hormones are not water-soluble and require transport proteins to be carried to their target tissues. The two types of transport proteins are general carrier proteins such as albumin and transthyretin and specific carrier proteins such as thyroxine-binding globulin, sex hormone-binding globulin (SHBG), and transcortin. Both types of proteins are produced in the liver. Less than 2% of ovarian steroid hormones are free in the circulation; the remainder are mostly bound to SHBG and albumin.45,46
Sex hormone-binding globulin, a β-globulin of 95 kDa, is synthesized in the liver. Its gene is localized on the short arm of chromosome 17 (p12-13).47 It is a homodimer composed of two polypeptide chains and has a single binding site for androgens and estrogens. Dimerization is a necessary step in the binding process.48 The bound and free fractions appear to exist in a steady state of equilibrium. The amount of free fraction depends on the concentration of steroid hormone and on the levels and binding affinities of the binding proteins.
Of all the steroid hormones, DHT has the highest affinity for SHBG. Approximately 98% of testosterone circulates bound to SHBG (≈65%) and albumin (≈33%). Estradiol is primarily bound to albumin (≈60%) but also to SHBG (38%); about 2% circulates as the free fraction.49 Progesterone, on the other hand, is mainly bound to albumin (≈80%) but also to transcortin (≈18%). Only approximately 0.6% of progesterone is bound to SHBG and about 2% exists in the free state.
The metabolic clearance of these steroids is inversely related to their binding affinity to SHBG. Thus, conditions that affect levels of SHBG (e.g., pregnancy, oral contraceptives) directly affect the levels of free hormone. Because estrogens increase SHBG synthesis and androgens decrease its synthesis, SHBG levels are twice as high in women compared to men. Several other hormones and other factors are known to influence SHBG levels. Thyroid hormones increase its synthesis and release by the liver.46 Insulin, IGF-I, and prolactin have been shown to inhibit SHBG production in cultured cells.50,51 Furthermore, serum concentrations of SHBG are increased in many disease states, including hyperthyroidism and liver cirrhosis. Certain drugs, including estrogen, tamoxifen, and phenytoin, can also increase serum SHBG concentrations. Carrier protein levels are decreased by hypothyroidism, obesity, and acromegaly and by administration of exogenous androgens, glucocorticoids, and growth hormones.
For many years, only the free fraction of testosterone was regarded as the biologically active component. However, researchers noted that steroid hormones bind with greater affinity to their specific carrier proteins and with much less affinity to albumin. In addition, studies of tissue delivery in vivo showed that the dissociation of albumin-bound testosterone can occur rapidly in a capillary bed so that the active fraction can be larger than the free fraction measured under equilibrium conditions in vitro.52 Thus, unconjugated steroids that are bound to albumin may be treated as free and biologically available.53,54
As mentioned above, SHBG levels can be influenced by numerous disease states. As such, changes in SHBG concentrations can result in large shifts in the free and SHBG-bound fractions. Hence, measurement of SHBG is of great clinical interest because it allows more accurate assessment of free hormones. SHBG is measured by a technique called saturation analysis, in which specific binding capacity of 3H-labeled testosterone is detected.55,56 With modifications, this method can also measure the non-SHBG bound fraction (bioavailable).57 Recently, specific nonisotopic two-site immunoassays for SHBG have become available and are used in most clinical laboratories.
Measurement of Steroid Hormones in Circulation
The technique responsible for the accurate measurement of low concentrations of various steroid hormones and metabolites is competitive inhibition immunoassay or radioimmunoassay (RIA), which was originally described in 1960 by Yalow and Berson.58 However, the development of steroid immunoassays presented several problems. First, they are not immunogenic and have a similar structure—they all have a same cyclopentahaptene nucleus with only minor structural variations—which makes it difficult to generate specific antibodies. Steroids can be made immunogenic via chemical coupling to a carrier protein known as hapten, and antibodies can be raised by immunization with haptens.59 However, the site of the steroid where the protein is conjugated has a significant impact on the specificity of the resulting antibody.59 Antibodies raised to conjugates of BSA coupled at the 19th position show higher specificity than those coupled at the 3rd or 17th positions.60 For accurate clinical interpretation, it is important to know the cross-reactivity data on each antibody that is selected for a given assay. Most commercial assay reagent manufacturers provide cross-reactivity data, but it may not always be reliable and must be evaluated in the clinical laboratories performing the assay.61
Second, the high-affinity binding proteins such as SHBG in the serum compete with the antibody and thus interfere with the measurement of steroid molecules by RIA. This makes direct measurement difficult and necessitates a preassay extraction procedure with organic solvents and often a chromatographic separation of the steroid. Alternatively, the use of certain chemicals, such as 8-anilinonaphthalene sulfonic acid can inhibit the binding of steroids to proteins, which allows the direct measurement of steroid hormones without the extraction step. Direct assays are fast and can be automated. Several automated platforms for measuring estradiol, progesterone, and testosterone are commercially available and are used in most clinical laboratories. These assays, however, have a low sensitivity and, when used to measure very low concentrations, have poor reliability.62 Therefore, they may not be the best choice for clinical applications that require the ability to measure very low hormone concentrations such as estradiol measurement in children and in men (<100 pg/mL),63 testosterone measurement in children and women(<1.5 ng/mL),64 or progesterone measurement during ovarian stimulation (<1 ng/mL).65 To overcome this problem, serum can be extracted with hexane-ethyl acetate (3:2 by volume), dried, and reconstituted in steroid-free serum, which can then be assayed in the automated platform.66
Gas chromatography combined with mass spectrometry (GC-MS) addresses many of the shortcomings of immunoassays and is considered more reliable and accurate than immunoassays. However, this technique requires multiple steps, including solvent extraction, chromatographic fractionation, and chemical dramatization before instrumental analysis, and it is often less sensitive than some immunoassays. This technique has now been superseded by liquid chromatography combined with tamdem mass spectrometry. This newer technique has a higher sensitivity and throughput than GC-MS and is considered a reference methodology.67,68 The technique has been used to simultaneously measure estradiol and estrone in human plasma with no cross-reactivity.69 These methods are recommended as reference methods and can be used to standardize and validate immunoassays, which provide the simplicity and rapid throughput needed for clinical use.
Estrogens
Measurement of estradiol is important in the assessment of female reproductive function. It can be used as an aid in the diagnosis of infertility and oligomenorrhea and to determine menopausal status (Table 2-2). In addition, measurement of estradiol is widely used to monitor ovulation induction and in vitro fertilization protocols.70,71 Estrone levels are of limited clinical value in nonpregnant women because their levels closely parallel those of estradiol, except in the postmenopausal woman, in whom estrone becomes the main form of circulating estrogen. Also, as mentioned above, a specific RIA for the measurement of estrone sulfate has been described and made available commercially. Because estrone sulfate has a large circulating pool, it can serve as a marker of estrogenicity, especially in women on estrogen replacement therapy, in whom estradiol measurements are of little value due to the variable cross-reactivity of conjugated estrogens in the estradiol assays.72,73 In pregnant women, estriol is the main form of estrogen produced, and the amount of estrogen secreted increases from microgram quantities to milligram quantities.
Table 2-2 Assay Techniques and Clinical Applications of Ovarian Steroid Hormones
Hormone | Assay Techniques | Clinical Application |
---|---|---|
Estradiol | ||
Estrone sulfate | Radioimmunoassay | Marker of estrogenicity in women on hormone replacement therapy |
Progesterone | ||
Total testosterone | ||
Free testosterone |
Progesterone
Although GC-MS has been recommended as the reference method for the measurement of progesterone,74 immunoassays using steroid-specific antibodies are again the preferred mode of measurement in most clinical laboratories.75,76 Both RIA and nonisotopic immunoassays for progesterone are available for commercial use. Progesterone measurement is routinely used to detect ovulation and luteal phase defects (see Table 2-2).77 In the follicular phase, progesterone levels are low (<5 nmol/L or 1.5 ng/mL). In the luteal phase, they range between 9 and 79 nmol/L (3 to 25 ng/mL). As noted, progesterone is required to maintain pregnancy, and progesterone measurement in early pregnancy can be valuable in the diagnosis of defects or threatened abortion.78
Androgens
The measurement of androgens, including androstenedione and total testosterone or DHT, can also be accomplished using immunoassays.79–81 The one main drawback of these assays is the cross reactivity with other steroid hormones. Interference with cross-reactive testosterone in the androstenedione assay has been overcome by the use of specific testosterone antiserum to neutralize plasma testosterone.81 Direct measurement of androstenedione and testosterone without extraction is now possible with new commercial assays. However, commercial assays demonstrate high variability, which is greatest in samples from females.82 Total testosterone in hirsute women overlaps significantly with levels seen in normal women, and measurement of free testosterone correlates better with disease.83 Free testosterone has been measured by equilibrium-dialysis, which is a time-consuming and difficult technique for most clinical laboratories to perform. An ultrafiltration technique can be used instead, which depends on MPS-1 centrifugal gel filtration devices and correlates well with the equilibrium-dialysis method83,84 as well as with GC-MS.85 Direct, single-step, nonextraction immunoassay methods using 125I-labeled testosterone analog have been developed commercially and are used in a number of laboratories. The accuracy and validity of this direct assay has been questioned.86,87 Alternatively, an indirect parameter of free testosterone—FAI—can be calculated as a ratio of testosterone to SHBG.88 FAI is a better discriminator of hirsutism than either total testosterone or SHBG levels.87–89 When bound with albumin and transcortin, testosterone dissociates more quickly than when bound with SHBG. This loosely bound teatosterone may be biologically available through dissociation during capillary transit. Cumming and Wall provided evidence for this hypothesis and suggested that this non-SHBG bound testosterone is a marker of hyperandrogenism.57,90
Saliva Measurements
SHBG is either undetectable or minimally present in saliva. Thus, this biologic fluid may reflect the free fraction of plasma steroids. Therefore, measurement of steroid hormones in saliva has attracted considerable attention.91–93 The ease of noninvasive collection combined with the simplicity of measurement makes salivary measurement a promising and attractive alternative to measurement of steroids in plasma. In the future, salivary assays may become useful adjuncts to those performed in plasma.94–97
PEPTIDE HORMONES AND INTRAOVARIAN GROWTH FACTORS OF OVARY
The role of gonadotropins and gonadal steroids in the process of folliculogenesis in the ovary is well recognized. However, multiple other phenomena within the ovary suggest the presence of other intraovarian factors that may fine-tune the effects of gonadotropins and gonadal steroids. For example, the initiation and arrest of meiosis and the different rates of follicular growth leading to the selection of a dominant follicle point toward the existence of an intraovarian modulatory system. The concept of a gonadal factor with endocrine action at the pituitary level can be traced back more than 70 years to Mottram and Cramer.98 The first moiety was identified and named inhibin for its inhibitory effect on the pituitary.99 Since then, there has been an explosion of information regarding multiple and potential intraovarian regulators and their physiology, biochemistry, and biosynthesis as well as the identification of their receptors. Some of these compounds, which have been the subject of intense investigation, include peptide hormones/growth factors, cytokines, and neuropeptides. These factors may act in either an endocrine, autocrine, or paracrine fashion.
Peptide Hormones of the Ovary: Inhibins, Activins, and Follistatins
The first water-soluble peptide hormone in testicular extracts that exerted selective inhibitory activity at the pituitary level was described in 1932 and termed inhibin.99 It was not until 1985 (almost 50 years later) that inhibin was isolated and characterized.100,101 This was followed by the identification and characterization of two other related peptide factors (i.e., activin and follistatin).102,103 With the cloning of inhibin α and β subunit genes,104 it was recognized that inhibin and activin belonged to the transforming growth factor-β (TGFβ) superfamily of growth and differentiation factors. Both inhibin and activin are of particular clinical interest and have been extensively reviewed3,105,106 in recent years. They have been found to exert numerous different regulatory functions in a wide variety of both normal and neoplastic cells. Follistatin, a glycoprotein that is structurally distinct but functionally closely linked to inhibin and activin, is also discussed here. The site of production and areas of clinical interest of these peptide hormones are listed in Table 2-3.
Inhibins
The primary sources of inhibin production are the granulosa cells of the ovary and the Sertoli cells of the testis. Inhibin is also produced during pregnancy by the fetus, placenta, decidua, and fetal membranes. The main role of inhibin is to selectively suppress the production of FSH by the pituitary.107,108 This is achieved by modulating FSH biosynthesis through two main mechanisms: by reducing steady-state FSH mRNA in pituitary gonadotropes109 and decreasing the stability of FSH mRNA.110
Inhibin is a 32 kDa glycoprotein heterodimer consisting of 2 subunits, α and β, linked by disulfide bonds.111 There is a common α subunit but also two types of β subunits known as βA or βB. Thus, the two isoforms of inhibin are denoted inhibin A and inhibin B. As shown in Figure 2-5, each subunit derives from a separate precursor molecule called prepro-inhibin α (364 amino acid residues), prepro-inhibin βA (424 amino acid residues) and prepro-inhibin βB (407 amino acid residues). These are processed by proteolytic cleavage to yield the mature forms.104 In addition to the fully mature forms (αβ dimers, Mr∼32,000), larger forms of dimeric inhibins with amino terminally extended α and/or β subunits have been identified in follicular fluid, which also possess FSH-suppressing bioactivity.112,113 Furthermore, monomeric forms of both α and β subunits and certain fragments (αN and proαN-αC) generated during subunit processing are present in follicular fluid (see Fig. 2-1) and have intrinsic biologic activities distinct from classical inhibin-like bioactivity.114,115
Because the circulation contains multiple molecular forms of inhibin, it can be difficult to accurately measure inhibin levels using conventional RIAs. Most lack specificity for dimeric inhibin due to the variable cross-reactivity of monomeric forms and of various fragments with the antibody used in the assay.112,116,117 Also, conventional inhibin bioassays based on FSH suppression or release by cultured pituitary cells lack specificity due to the FSH-regulating activities of follistatin and activins. In addition, bioassays lack the sensitivity necessary to measure inhibin levels in the circulation. The development of two-site immunoassays utilizing αβ dimer specific antibodies overcame these problems and allowed specific measurement of the two forms of inhibin dimers (A and B).118 Use of these novel two-site assays allows measurement of inhibin levels throughout the normal menstrual cycle.119
Synthesis of the two isoforms of inhibin differ during the various phases of the menstrual cycle (see Fig. 2-4). Inhibin B levels are highest during the luteal–follicular transition and the early follicular phase, and studies on its presence in follicular fluid and basal granulosa cell secretion suggest that it is secreted by small developing antral follicles.120 In contrast, inhibin A levels in the early and midfollicular phase reflect the sum of FSH- and LH-stimulated inhibin A secretion from all antral follicles. Levels of inhibin A during the late follicular phase mainly reflect secretion from the dominant follicle. Hence, inhibin A and B levels can be used as markers of follicular development. Inhibins have also been investigated as prognostic markers for women undergoing assisted reproductive technologies. In particular, it was suggested that measuring inhibin B levels during the early stages of FSH stimulation for ovulation induction could predict the number of oocytes retrieved and may be useful in monitoring ovarian stimulation for in vitro fertilization. However, because there is a large overlap between normal and subnormal ovarian responses in terms of inhibin B levels, it may be just as effective to obtain Day 3 FSH or perform a clomiphene challenge test.121,122 Women in early perimenopause show significant decrease in inhibin B (no significant change in inhibin A and estradiol), which correlates with a mild increase in FSH levels. This perimenopausal decrease in inhibin B precedes a decrease in inhibin A, suggesting that inhibin B may serve as a sensitive marker for the onset of menopause.123 Studies investigating the role of inhibins in the pathophysiology of polycystic ovary syndrome (PCOS) show conflicting results. Serum inhibin B levels in early follicular phase show significant increase in some studies or no change in others124,125—this awaits future studies to confirm its role in PCOS.
During pregnancy, inhibin A is produced primarily by the fetoplacental unit, whereas inhibin B levels remain low throughout the pregnancy.126 Because there is a twofold elevation of circulating inhibin A levels in the second trimester of Down syndrome pregnancies, measurement of inhibin A is a clinically useful and important test.127 When measurement of inhibin A level is added to alpha-fetoprotein, maternal age, and β-hCG (quad screen), the detection rate for Down syndrome increases from 53% to 75%.128
Inhibin A has also recently been used as a tumor marker for ovarian sex cord tumors. This heterogeneous group of tumors accounts for 7% of all malignant primary ovarian neoplasms. They are composed of granulosa cells, thecal cells, Sertoli cells, Leydig cells, and other nonspecific stromal cells. It is important to distinguish this group of tumors from carcinomas and sarcomas because the former are low-grade tumors with a better prognosis. Inhibin A and its α-subunit have been found to be sensitive immunohistochemical markers of most ovarian sex cord-stromal tumors.129 Inhibin B, however, has been found to be elevated in both sex cord-stromal and epithelial tumors and hence is of limited value in differentiating between the two entities. In addition, low levels of inhibin A in the cyst fluid of epithelial ovarian tumors has recently been reported to be associated with a worse prognosis.130
Inhibin B has been found to be the predominant inhibin secreted in males and is produced by the Sertoli cells of the testes. It also has a negative feedback role on FSH from the pituitary, and its production is regulated by spermatogenesis. Inhibin B levels correlate with sperm count and testicular volume131–133 but cannot distinguish between spermatid arrest and obstructive azoospermia—a condition in which sperm counts are normal.134 As such, it is unlikely to replace testicular biopsy in the evaluation of male infertility.
Activins
Activins are made up of dimers of the inhibin β subunit (βAβA, βAβB, or βBβB) and have a molecular weight of approximately 25 kDa (see Fig. 2-5).135 They are predominantly produced by the granulosa cells of the ovary. Activin/inhibin mRNA and protein have also been detected in extragonadal sources, including the placental trophoblast and decidua, testes, adrenal cortex, brain, spinal cord, and anterior pituitary. This implies that activin has diverse physiologic roles that are not confined to the reproductive system.
Acting either alone or with FSH, activin exerts an autocrine effect on granulosa cells to promote and maintain granulosa cell differentiation. It promotes FSH receptor expression on small undifferentiated granulosa cells136, enhances their response to FSH and LH, and hence increases aromatase activity and estrogen production.137 This may explain how small preantral follicles progress from a gonadotropin-independent to a gonadotropin-dependent stage of development. After the granulosa cells acquire FSH receptors, further growth and differentiation of those cells to a preovulatory stage would be driven by activin acting in concert with FSH. Activin also inhibits both spontaneous and LH/hCG-induced increases in progesterone output by human follicles,137 implying that it plays a role in delaying the onset of premature luteinization.
Activin also has a paracrine effect on thecal steroidogenesis by inhibiting thecal androgen output. It has been proposed that at earlier stages of follicular development, when the androgen requirements are low, thecal androgen synthesis is kept in check due to the relative excess of activin over inhibin and follistatin. However, as dominant follicles approach preovulatory status, increasing granulosa cell expression of inhibin and follistatin upregulates thecal androgen synthesis and ensures that the granulosa cells receive an adequate supply of aromatase substrate for conversion to estradiol. Activin stimulates pituitary FSH production, acting as a functional antagonist to inhibin (Fig. 2-6).102 It achieves this effect by increasing FSH mRNA synthesis as well as by increasing the stability of produced mRNA. Its actions are intimately modulated by intrapituitary concentrations of follistatin, which bind to activin to limit its bioavailability.
Free activin levels as measured by competitive protein binding assay, using follistatin as binding protein, show very little change over the menstrual cycle.138 However, activin levels were elevated throughout the cycle in older versus younger women, suggesting that activin may play an endocrine role in maintaining FSH elevation in reproductive aging.139 Lower levels of activin are detected in PCOS patients with a simultaneous increase in inhibins and follistatin, suggesting that an imbalance in these hormones may contribute to an abnormal LH/FSH ratio.125,140
Follistatin
In the ovary, follistatin is produced by the granulosa cells in antral follicles as well as by luteinized granulosa cells, which are under the positive regulation of FSH. It modulates the function of granulosa cells in favor of luteinization or atresia by neutralizing the effects of activin. It may also directly modulate progesterone metabolism by granulosa cells.141
Follistatin is a single-chain polypeptide (315 amino acids) that functions as the binding protein for activin, thereby neutralizing it. Most of its biology is explained by its antagonism with activin. Follistatin exists in two forms; as full-length follistatin (FS 315) in the circulation and as processed follistatin (FS 288) in follicular fluid142 and the pituitary. It is part of an intrapituitary negative feedback loop where activin promotes FSH biosynthesis and the increased expression of follistatin limits its bioavailability for binding to the activin receptor on target cell membranes. It has been shown that the processed isoform of follistatin (FS 288) binds to cell-surface heparan sulfate proteoglycans with a higher affinity than FS 315.143 Because proteoglycans are anchored to cell membranes, this suggests that this would limit follistatin diffusion from the site of release, leading to high local concentrations of follistatin being maintained. The membrane-anchored follistatin would be able to compete with activin receptors on nearby cells, thus modulating the biologic effects of activin. Once bound to activin, follistatin is able to accelerate the endocytotic internalization and lysosomal degradation of activin by pituitary cells.144
Follistatin levels as measured by sensitive and specific two-site enzyme immunoassay are significantly higher in women with PCOS in comparison to controls. Higher follistatin levels combined with lower activin levels in these patients suggest its role in the lack of pre-ovular follicle development and FSH suppression.125
Growth Factors/Cytokines As Intraovarian Regulators
Certain growth factors have been implicated in cellular communications within the ovary, including insulin-like growth factors IGF-I and IGF-II, epidermal growth factor (EGF), transforming growth factor-α (TGFα), basic fibroblast growth factor (bFGF), cytokines such as interleukins (IL-1 and IL-6), and tumor necrosis factor (TNFα). Growth factors and cytokines that are important as intraovarian regulators are outlined in Table 2-4.
Insulin-like Growth Factors
IGF-I and IGF-II promote cellular mitosis and differentiation in a variety of systems and play an important role in modulating folliculogenesis in an autocrine/paracrine fashion.145–147 Insulin-like growth factors consist of two single-chain polypeptide growth factors that are structurally and functionally similar to proinsulin. The IGF autocrine/paracrine system includes IGFs, their specific receptors in target cells, and a family of IGF-binding proteins that regulate their bioavailability. Both IGF-I and IGF-II are produced in the ovary and augment the effects of the gonadotropins, although the main IGF in human follicles is IGF-II.148 In small antral follicles, both IGF-I and IGF-II are expressed but restricted to thecal cells. However, IGF-I receptor mRNA has been detected only in granulosa cells.146 It serves as an autocrine regulator in thecal cells and as a paracrine regulator in granulosa cells. In dominant follicles, however, no IGF-I mRNA has been detected either in thecal or in granulosa cells, and IGF-II expression is restricted to granulosa cells only. IGF-I receptors are present in granulosa cells only, and IGF-II receptors are expressed in both cell types.146 Thus, in the dominant follicle, IGF-I functions mainly as a paracrine regulator149,150 and IGF-II acts as an autocrine factor. This suggests that IGF-II has an important role in coordinating differential follicular development within the ovary.
Epidermal Growth Factor (EGF), Transforming Growth Factor-α (TGFα), and Basic Fibroblast Growth Factor (bFGF)
A number of other peptide growth factors have been implicated as regulators of follicular development and steroidogenesis (see Table 2-4).151 These include EGF, TGFα, and bFGF. EGF is a single-chain polypeptide of 53 amino acids with three disulfide bonds and has mitogenic effects in a variety of ectodermal and mesodermal tissues. TGFα is a 50-amino acid peptide with 30% to 40% homology with EGF. The EGF receptor is a 170-kDa glycoprotein with tyrosine kinase activity. TGFα binds to EGF receptors with the same affinity as EGF.
The presence of EGF/TGFα and bFGF as well as their receptors in the ovary has been shown both at the protein and mRNA levels. The presence of immunoreactive EGF as well as EGF receptors has been identified in the preovulatory follicles152 and corpus luteum.153,154 Furthermore, studies have shown the presence of TGFα155 and bFGF156 mRNAs in follicular cells; studies have also shown that the TGFα message is upregulated by FSH in vivo. FSH plus TGFα or FSH plus EGF resulted in significantly elevated progesterone and 20α-hydroxyprogesterone levels in granulosa cells in culture.157 The presence of TGFα message in cultured granulosa cells and the fact that it mediates its action via binding to EGF receptor all point to its autocrine role in granulosa cell differentiation, follicle development, and selection. Expression of bFGF and its receptor mRNA has been detected in fetal ovaries and in granulosa cells.158,159 bFGF has been shown to be mitogenic for granulosa cells and to cause an inhibitory action on granulosa cell differentiation and thecal cell steroidogenesis.160,161 It also has potent angiogenic activity.162
Cytokines
Cytokines primarily produced by white blood cells modulate various cellular functions. The cytokines in the ovary are secreted both by the immune cells that are recruited from the circulation in the ovarian stroma and by the thecal and granulosa cells. A number of cytokines have been linked to modulation of ovarian function, including interleukins IL-1 and IL-6 and TNFα. Both IL-1 and IL-6 have been found in significant amounts in follicular fluid.163,164 Granulosa cells accounted for the majority of immunostaining for IL-1 and IL-6 in follicular aspirates, which suggests that these cytokines are produced in granulosa cells164,165 and that they affect granulosa function.166 During folliculogenesis, IL-I promotes proliferation and suppresses differentiation. In the ovulatory process, it promotes ovulation by increasing production of chemokines, steroids, ecosanoids, and vasoactive substances.167 IL-6 demonstrates inhibitory effects on both estradiol and progesterone secretion by FSH-stimulated granulosa cells.168 Elevated IL-6 levels during genital infections may provide a possible link to reproductive dysfunction. TNFα expression has also been detected in granulosa cells of human antral and atretic follicles by immunohistochemistry.169 Also, in vitro treatment with TNFα enhanced steroidogenesis in both healthy and atretic follicles,170 suggesting that TNFα has a paracrine and/or autocrine role. Nonetheless, the physiologic implications of these actions remain unclear and require further investigation.
Neuropeptides
Some evidence suggests that an independent ovarian-central nervous system axis exists.171,172 Electrical stimulation of the hypothalamus in hypophysectomized and adrenalectomized rats produced a change in ovarian steroidal synthesis that was independent of changes in ovarian blood flow.173 In addition, murine thecal cells can produce androgens under adrenergic stimulation.174 Adrenergic innervation of the ovary acts primarily on the thecal-interstitial cells through β2 receptors, synergizing with the effects of gonadotropins in the production of ovarian androgens.174 This may in turn play a role in the regulation of estrogen production by granulosa cells, thereby influencing follicle recruitment and selection.
REGULATION OF OVARIAN HORMONES
The hypothalamic-pituitary axis plays a key role in the regulation of hormonal synthesis by the ovaries. The hypothalamus is connected to the pituitary gland via a portal vascular system that allows transport of hypothalamic releasing factors from the brain to the pituitary (see Fig. 2-6). The hypothalamus, being the coordinating center, provides precise signals via pusatile release of GnRH to the gonadotrophs, which in turn secrete LH and FSH. Any interruption in this connection results in low gonadotropin levels, leading to failure of ovarian hormone secretion.
Hypothalamic Regulation
Gonadotropin-releasing Hormone
The gonadotropin-releasing activity in hypothalamic extracts was first demonstrated in the late 1950s. In 1971, almost 15 years later, GnRH was first isolated and characterized from a hypothalamic extract. This was followed by its synthesis and its clinical availability. However, early clinical studies proved disappointing until the nature of its pulsatile secretion was recognized. In their classic experiment in the rhesus monkey, Knobil and colleagues showed that normal LH and FSH release required the pulsatile infusion of GnRH at approximately 60-minute intervals175 Furthermore, a change in pulse frequency (lesser or greater) or continuous infusion resulted in failure of LH and FSH secretion and release (Fig. 2-7).176 These observations were confirmed in humans. Pulsatile GnRH infusion reproduces appropriate patterns of hormonal changes, resulting in ovulation and fertility in women with hypothalamic amenorrhea.
Physiologic Role of GnRH
GnRH is produced by secretory neurons located in the arcuate nucleus of the medial basal hypothalamus and the preoptic area of the anterior hypothalamus. The nerve terminals are found in the lateral portions of the external layer of the median eminence adjacent to the pituitary stalk.177 GnRH has an intrinsically pulsatile pattern of secretion, which is under the control of a hypothalamic pulse generator in the arcuate nucleus.178,179 The frequency and amplitude of the pulsatile rhythm of GnRH secretion are crucial in regulating gonadotropin secretion and hence gonadal activity.180,181 Physiologic frequency (approximately hourly pulses) tends to upregulate GnRH receptors, enhancing pituitary responsiveness to subsequent stimulation by GnRH. This leads to a “self-priming” effect, whereby LH levels have been shown to increase sequentially with sequential GnRH pulses. A longer frequency causes anovulation and amenorrhea; a shorter frequency or constant exposure to GnRH downregulates the GnRH receptors, inducing refractory gonadotropin responses.176,182–184
The “pulse generator” is subject to modification by two main inputs: (1) hormone-mediated signals and (2) neural signals. Hormone signals include negative and positive feedback from the gonadal steroids (e.g., estrogen and progesterone) as well as gonadal protein hormones. Neural signals may come from a wide variety of sources and are mediated by neurotransmitters, including acetylcholine, catecholamines, serotonin, opioids, and γ-aminobutyric acid.185 Norepinephrine is believed to stimulate GnRH release whereas opioids exert inhibitory effects. Dopamine can produce both inhibitory and excitatory GnRH responses, depending on the physiologic state.186
Biochemistry and Biosynthesis
GnRH is a linear decapeptide, derived from the post-translational processing of a large precursor molecule, prepro-GnRH. The prepro-GnRH molecule consists of 92 amino acids in a tripartite structure (Fig. 2-8). It begins with a signal peptide of 23 amino acids, which is followed by the decapeptide and then a Gly-Lys-Arg sequence needed for proteolytic processing and C-terminal amidation of GnRH molecules. The last 56 amino acid residues are collectively known as the GnRH-associated peptide (GAP), which may have prolactin-inhibiting activity.187,188 Knowledge of GnRH structure led to the development of many clinically important long-acting GnRH agonists, including buserelin, leuprolide, and nafareline (see Fig. 2-8).
Gonadotropin-releasing hormone is encoded from a single gene on the short arm of chromosome 8p21-p11. The human gene contains 4 exons; exon 2 encodes pro-GnRH, exon 3 and part of exon 2 and 4 encode the GAP protein, and exon 4 encodes a long 3′ untranslated region. Molecular processing occurs primarily in the nucleus of the cell body (soma). After transcription, the mRNA is transported to the cytoplasm where translation takes place and it is converted into the decapeptide. GnRH and its cleavage products, GAP and pro-GnRH, are then transported to the nerve terminals where they are secreted in tandem into the portal circulation.187,189,190
Measurement
GnRH has a short half-life (2–4 min) due to its degradation by peptidases in the hypothalamus and pituitary gland. These peptidases cleave the molecule at the Gly-Leu bond and at position 10. Due to the large dilutional effect, peripheral GnRH serum levels are too low for pulse characteristics to be determined reliably in humans. However, the simultaneous measurement of circulating levels of LH in hypophysial-portal and peripheral blood samples in other species has been shown to correlate closely with GnRH release.191,192 As such, frequent measurements of LH pulses can be used as an accurate indicator of GnRH secretion patterns. FSH also correlates with GnRH secretion, but is less useful due to its long half-life.
Regulation by Pituitary Hormones
As mentioned above, GnRH action on gonadotrophs stimulates gonadotropin production and release in a pulsatile fashion. In addition, LH and FSH release from the pituitary is also affected in both a positive and negative manner by estrogen and progesterone as well as by the protein hormones secretion by the ovaries (see Fig. 2-6). The positive effect of estrogen and the negative effect of progesterone on the gonadotropins depend on the level of steroid hormone and the duration of exposure to the gonadotrophs. On the other hand, both LH and FSH are required for ovarian estrogen synthesis and the level of estrogen production depends on the time of exposure and the level of gonadotropins.193,194 Nonetheless, a disordered signal from the pituitary gland may result in infrequent ovulation (oligo-ovulation) or absent ovulation (anovulation).
Regulation by Luteinizing Hormone and Follicle-stimulating Hormone
Physiologic Role
As discussed above, LH regulates ovarian steroidogenesis. The surge of LH in the middle of the menstrual cycle is also responsible for inducing ovulation. The surge occurs as a result of a dramatic rise in estradiol produced by the preovulatory follicle, which produces a positive feedback on LH. The midcycle surge stimulates the resumption of meiosis and the completion of reduction division in the oocyte with release of the first polar body. Proteolytic enzymes and prostaglandins are increased in response to LH, leading to a release of the oocyte from the ovary.195 Finally, the continued secretion of LH after ovulation converts the remaining follicular cells in the ovary to the corpus luteum and stimulates the corpus luteum to produce progesterone by enhancing the conversion of cholesterol to pregnenolone.
Follicle-stimulating hormone regulates ovarian estrogen synthesis by binding to the FSH receptor on the surface of the granulosa cell and is required for follicular maturation and growth.196,197 This results in elevated cyclic adenosine monophosphate (cAMP) levels and the induction of aromatase, which converts androstenedione from the neighboring thecal cells to estrone. FSH also induces expression of 17βHSD type 1, which converts estrone to estradiol. Increased secretion of estradiol leads to further proliferation of granulosa cells and follicular growth and an increase in the number of estradiol receptors.196,198,199 In the mature follicle, FSH and estradiol increase the LH receptors’ expression in granulosa cells, making these cells responsive to LH and augmenting progesterone secretion. Progesterone then increases FSH release in midcycle.
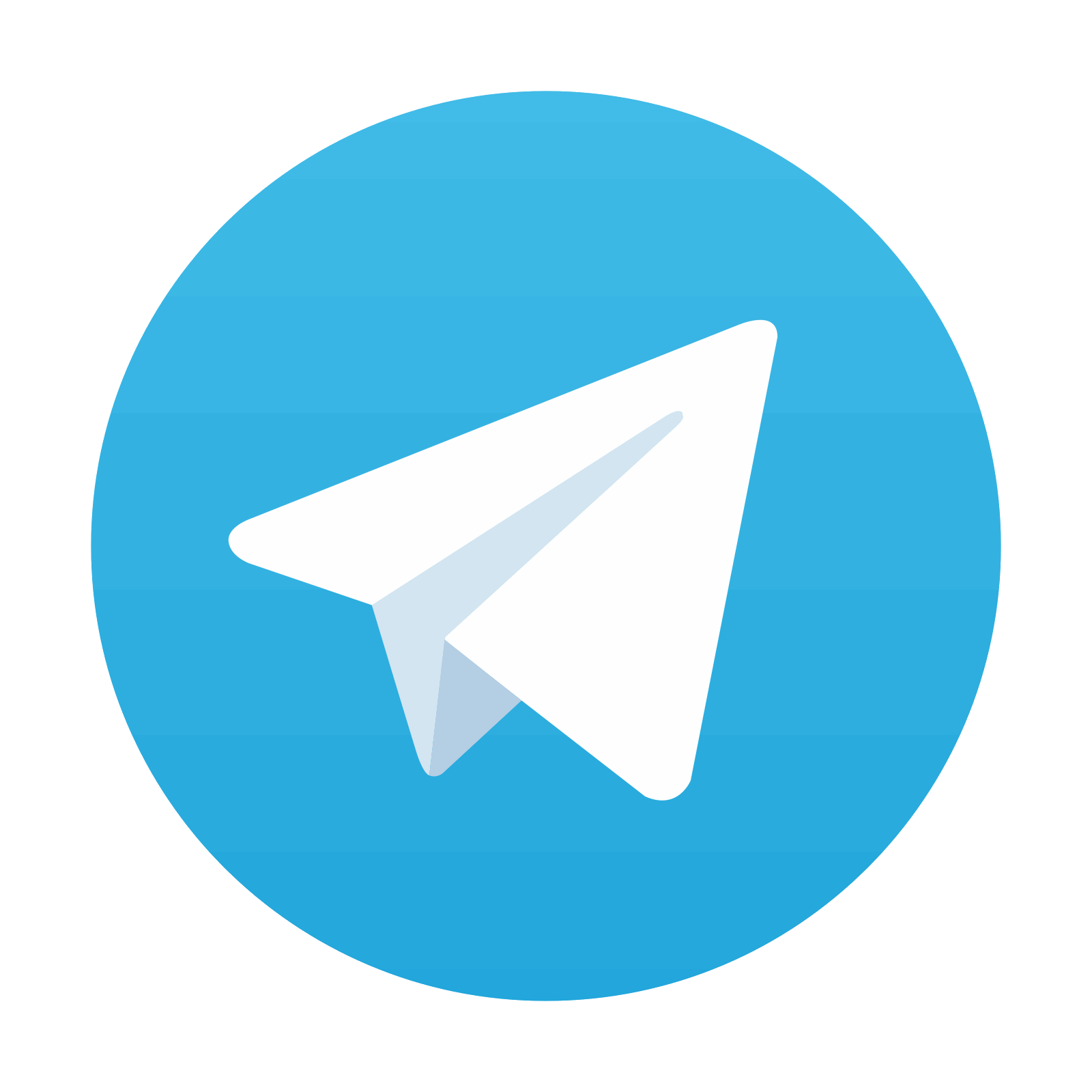
Stay updated, free articles. Join our Telegram channel

Full access? Get Clinical Tree
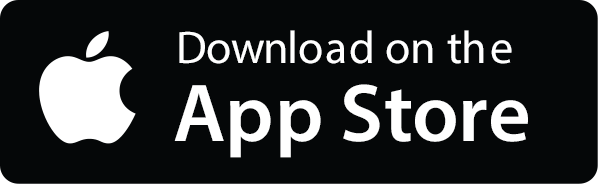
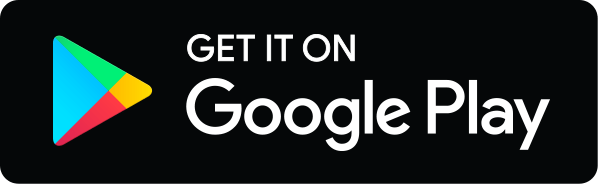