Fig. 1
Pig-to-human xenotransplantation as a potential solution to the organ shortage for human end-stage organ diseases
Despite the above advantages of using pigs as a donor organ source, there are also disadvantages. Pigs have a shorter life span than humans, so the organ transplant will have to be performed more than once since the pig organs have the potential to deteriorate at a much faster pace than an actual human organ. In addition, as pigs have a distant evolutionary relationship with humans, the human immune system would mount a very strong response to pig organs, leading to the organ transplants being rejected quickly, even when the immunosuppressive drugs that are supposed to prevent rejection of human transplants are used. It seems that drugs are simply not powerful enough to prevent rejection when pig organs are transplanted to humans.
To make pigs more suitable as the organ source for xenotransplantation, the problem of xenograft-induced immunological rejection needs to be solved. One solution for this is to take advantage of genetic engineering methods to modify pigs so that their organs will appear to be a part of the human body and will not be recognized as “nonself” when transplanted into humans. Genetically modified (GM) pigs have thus been produced for xenotransplantation research around the world. Although these GM pigs are still in the laboratory, progress made in the last decade suggests that the move to the clinic is not too far away, with cell xenotransplantation probably more feasible in the near future.
In order for GM pigs to serve well as an organ source for xenotransplantation, we need to know what the immunological challenges are and how to prevent them.
Immunological Challenges
The most profound obstacle to a successful pig-to-primate xenotransplantation is the rejection of the organ transplant by a cascade of immune responses commonly known as hyperacute rejection, acute humoral xenograft rejection, cell-mediated immune rejection, and chronic rejection.
Hyperacute rejection is a rapid process of powerful immune responses that lead to the rejection of xenografts within a few minutes or hours after the surgery of xenotransplantation. It mainly destroys the vasculature of the xenografts, with subsequent interstitial hemorrhage, edema, and thrombosis of the small vessels. The process is initiated by binding of the host antibodies to the xenograft antigens that trigger the complement activation, resulting in endothelial damage, inflammation, and necrosis of the xenografts and leading to transplant failure. It is widely accepted that the host xenograft antigen’s active IgM or IgG initiates the hyperacute rejection process. Thus, hyperacute rejection represents the first barrier to clinical solid-organ xenotransplantation.
The main target antigen of the pig organs recognized by the primate immune system is α-1,3-galactose (α1,3Gal), an oligosaccharide that is produced by an enzyme called α-1,3-galactosyl transferase (α1,3GalT) (Galili et al. 1988). As most non-primate animals, including pigs, have this enzyme, the α1,3Gal is naturally expressed in endothelial cells and becomes the target of the host immune system. However, primates, including humans, actually lack α1,3GalT, so no α1,3Gal is expressed in the primates; however, primates have been exposed to α1,3Gal-similar epitopes derived from gut bacteria and have a high titer of anti-α1,3Gal antibodies in the body (spleen, lymph nodes, and bone marrow) already, which is why primates can mount an immune response to this antigen so quickly.
To reduce the frequency of hyperacute rejection, many approaches have been pursued to either remove the preexisting anti-α1,3Gal antibodies or control their effectors’ functions by inhibiting complement cascade. Among them, the most well-known approach is to generate GM pigs by knocking out the gene that is responsible for α1,3GalT, so no α1,3Gal will be produced (Phelps et al. 2003).
Acute humoral xenograft rejection is a later immune response reaction that follows the hyperacute rejection. This delayed process is much more complex than hyperacute rejection and is mainly driven by interactions between the xenograft endothelial cells and host antibodies, leading to loss of the xenograft within days or weeks of transplantation (Crikis et al. 2006). An inflammatory infiltrate of mostly macrophages and natural killer (NK) cells, intravascular thrombosis, and fibrin deposition are involved in the rejection. The detailed mechanism of acute humoral xenograft rejection is currently not completely understood. Recent evidence demonstrates that anti-non-α1,3Gal antibodies directed against both carbohydrates and proteins play a critical role in the acute humoral xenograft rejection (Breimer 2011). Due to its multifactorial features, strategies to overcome acute humoral xenograft rejection require the combination of different approaches to generate synergistic effects. Thus, the use of immunosuppressive drugs along with GM pigs as organ donor should result in improved survival.
Cell-mediated immune rejection, which is different from hyperacute rejection and acute humoral xenograft rejection which are both xenograft antigen dependent, is mainly mediated by T cells. T cells play a role in the induction of anti-xenograft antibodies, but their role in direct involvement of rejection has not completely been clarified. However, it is clear that T cells can recognize xenograft antigens presented by major histocompatibility complex (MHC) molecules in direct and indirect pathways. Direct xenorecognition is via CD4 T cells, in which xenograft antigens are presented directly by MHC class II molecules on antigen-presenting cells from the xenograft; indirect xenorecognition is via CD8 T cells, in which xenograft antigens are first phagocytosed by the host antigen-presenting cells and then presented by MHC class I molecules on the host antigen-presenting cells. The indirect xenorecognition is thus expected to be stronger than its allogeneic counterpart, since the large number of antigens from xenografts is more readily recognized as foreign and elicits stronger immune responses.
Evidence obtained from pig-to-primate xenotransplantation experiments has demonstrated that CD8 T cells, monocytes/macrophages, B cells, and some NK cells are the predominant cells detected in the xenograft; CD4 T cells are only described in a limited number of cases (Ashton-Chess et al. 2003; Hisashi et al. 2008). It is generally believed that the cell-mediated immune rejection can be controlled by using the current immunosuppressive regimens.
Chronic rejection usually occurs in the xenografts after the initial acute antibody-based and cellular rejections. It is relatively slow and progressive. Knowledge in this area is poor as most xenografts rarely survive long enough for it to be studied. However, it is known that fibrosis of the xenograft vessel wall is the major cause of chronic rejection. Arteriosclerosis occurs as a result of the combinatorial effects of T cells, macrophages, cytokines, and healing, leading to the hardening and narrowing of the vessels within the xenograft. Chronic rejection is believed to be more aggressive in xenografts than in allografts. As chronic rejection causes pathological changes in the organ, the xenograft will have to be replaced after several years.
In addition to immunological challenges, dysregulated coagulation remains another barrier to successful xenotransplantation, particularly pig-to-primate xenotransplantation. There is a difference in the coagulation dysfunction in different organ xenotransplants. Lung xenotransplant is the most rapidly damaged organ xenograft by coagulation dysfunction and rarely lasts more than a day in nonhuman primates. Kidney xenotransplants have a higher degree of coagulation dysfunction than that of the heart, while liver xenografts are more easily affected by thrombocytopenia. Coagulation dysfunction is one of the molecular incompatibilities in pig-to-primate xenotransplantation and represents the most problematic issue. Strategies to overcome coagulation dysfunction in xenotransplantation will include the combination of GM pigs to reduce the effects of clotting cascade in the xenograft donor and the systemic treatment of the recipient to aid ready acceptance of the xenograft.
Among these immunological rejections, hyperacute rejection, acute humoral xenograft rejection, and acute cellular rejection are generally controllable when an adequate immunosuppressive regimen is given, but chronic rejection in the form of xenograft vasculopathy has been documented in cardiac transplants that survive for several months. Xenograft vasculopathy could be increasingly delayed as the immunological challenges of xenotransplantation are overcome. One of the practical approaches is to use GM pigs.
Genetically Modified Pigs
The ultimate goal of generating GM pigs is to reduce or eliminate immunological responses of the donor organs and make the xenografts more acceptable by the recipient.
As described above, the pig is the preferred species as an organ donor for xenotransplantation due to its comparable organ size, rapid growth rate, large litters, and a more manageable ethical profile in comparison with other species. However, it is well documented that the existence of xenoreactive natural antibodies (XNA) in the recipient can recognize the α1,3Gal epitope and triggers a hyperacute rejection, a very rapid immune response that results in irreversible xenograft damage and loss within minutes to hours after the transplantation. Nevertheless, selection of donor organs that do not express α1,3Gal would be a better strategy for xenotransplantation. Thanks to the development of modern molecular biology, this can be achieved by generating GM pigs that lack the expression of α1,3Gal through genetic engineering.
The production of α1,3Gal is catalyzed by an enzyme α1,3GalT, which is encoded by the gene GGTA1. This gene is expressed in fetal fibroblasts and the α1,3Gal is readily detectable on the cell surface. To make the cell lack α1,3Gal expression and, hence, the epitopes to XNA, inactivation of the GGTA1 gene is needed. α1,3GalT, a 371 amino acid protein, is encoded by 4–9 exons of GGTA1. The gene’s endogenous translation start codon ATG is in exon 4, while the major portion of the protein including the catalytic domain is in exon 9. Thus, both exons have become the targets for the functional inactivation of GGTA1 in transgenic pigs. The first transgenic pig (α1,3GalT−/−) lacking the α1,3GalT was generated using a somatic cell nuclear transfer (SCNT) method in 2002 (Lai et al. 2002). Grafts from α1,3GalT−/− pigs can generally achieve an extended graft survival time and allow the use of reduced levels of the immunosuppressive therapy. The associated rejection is not caused by the classical acute humoral xenograft rejection, but predominantly by the development of a thrombotic microangiopathy that can ultimately result in coagulopathy. Moreover, the level of antibodies against non-α1,3Gal epitopes has been found to be elevated at the time of rejection, indicating the importance of antibodies against non-α1,3Gal antigens in xenotransplantation. It appears that the anti-non-α1,3Gal antibodies represent a major critical barrier for the successful clinical application of xenografts. Apparently, transgenic pigs will be used with a combination of not only the functional inactivation of GGTA1 but also other genetic factors for the further reduction of the rejection process in xenotransplantation.
Another xenoantigen, N-glycolylneuraminic acid (Neu5Gc), has been identified recently (Song et al. 2010). Humans do not produce Neu5Gc as humans have a DNA mutation that can cause the functional inactivation of cytidine monophosphate-N-acetylneuraminic acid hydroxylase (CMAH), the enzyme responsible for Neu5Gc production, but CMAH is synthesized in pigs and other mammals, including nonhuman primates. It is therefore expected that deleting both the GGTA1 and CMAH genes to create double-knockout (KO) pigs may further reduce the xenoantigenicity of pig organs in humans and thus further reduce the severity of humoral rejection as seen in the GGTA1 single KO mentioned above.
To generate double-KO pigs, a zinc-finger nuclease (ZFN) technology has been used with sequential disruption of the GGTA1 and CMAH genes in cultured cells followed by SCNT to yield viable GM pigs. Compared with the standard technique based on homologous recombination, ZFN technology is more efficient and is able to knock out more than one gene at a time, which should accelerate the development of GM donor pigs to evaluate for clinical xenotransplantation.
Transgenic pigs expressing human complement regulatory proteins have shown great promise in reducing the rejection of pig organs following transplantation into nonhuman primates. Various transgenic pigs expressing a single gene product of CD46, CD55, and CD59 have been produced. Double- and triple-transgenic pigs are also established. The gene expression levels vary in different transgenic pigs. In order to control the expression of transgenic genes, the tetracycline-regulated Tet-On and Tet-Off system is used. This system allows the transgene expression in a controllable way by exogenous stimuli.
Transgenic pigs expressing the enzyme α-1,2-FT can reduce expression of the major pig xenoantigen α-1,3-Gal by enzymatic competition between α-1,3-GalT and other terminal glycosyltransferases for the common acceptor substrate, resulting in a reduction in xenoreactive human natural antibody binding and complement activation. Therefore, pigs transgenic for the human α-1,2-FT gene can be comparable with their α-1,3-Gal-deficient counterparts.
It is expected that donor organs from GM pigs with a combination of some specific gene KOs and transgenes might even further reduce the immunological rejection rates for clinical xenotransplantation. Indeed, such multi-transgenic pigs with α-1,3-Gal KO and other transgenes such as CD55 or/and α-1,2-FT have been produced.
With regard to cell-mediated immune rejection, expression of human tumor necrosis factor (TNF)-α-related apoptosis-inducing ligand (TRAIL) in transgenic pigs has been used as a strategy to control post-hyperacute rejection mechanisms mediated by cellular components of the immune system. Another strategy is to inhibit the activity of NK cells by expressing HLA transgenes, mainly HLA-E. In addition to T cells and NK cells, macrophages play an essential role in both innate and adaptive immune responses. Signaling regulatory protein (SIRP)-α is expressed on macrophages that can recognize CD47, a cell surface protein expressed ubiquitously on most cells as a marker of “self.” “Self” cells thus use this SIRP-α–CD47 interaction to avoid being phagocytosed by macrophages (Ide et al. 2007). Hence, transgenic pigs expressing human CD47 are likely to contribute to the xenotransplantation by inducing immune tolerance in xenografts.
With the availability of a plethora of GM pigs, it is expected that various immunological rejections can be reduced and xenotransplantation will likely become a clinical reality in the not-too-far future, at least as a bridge to allotransplantation.
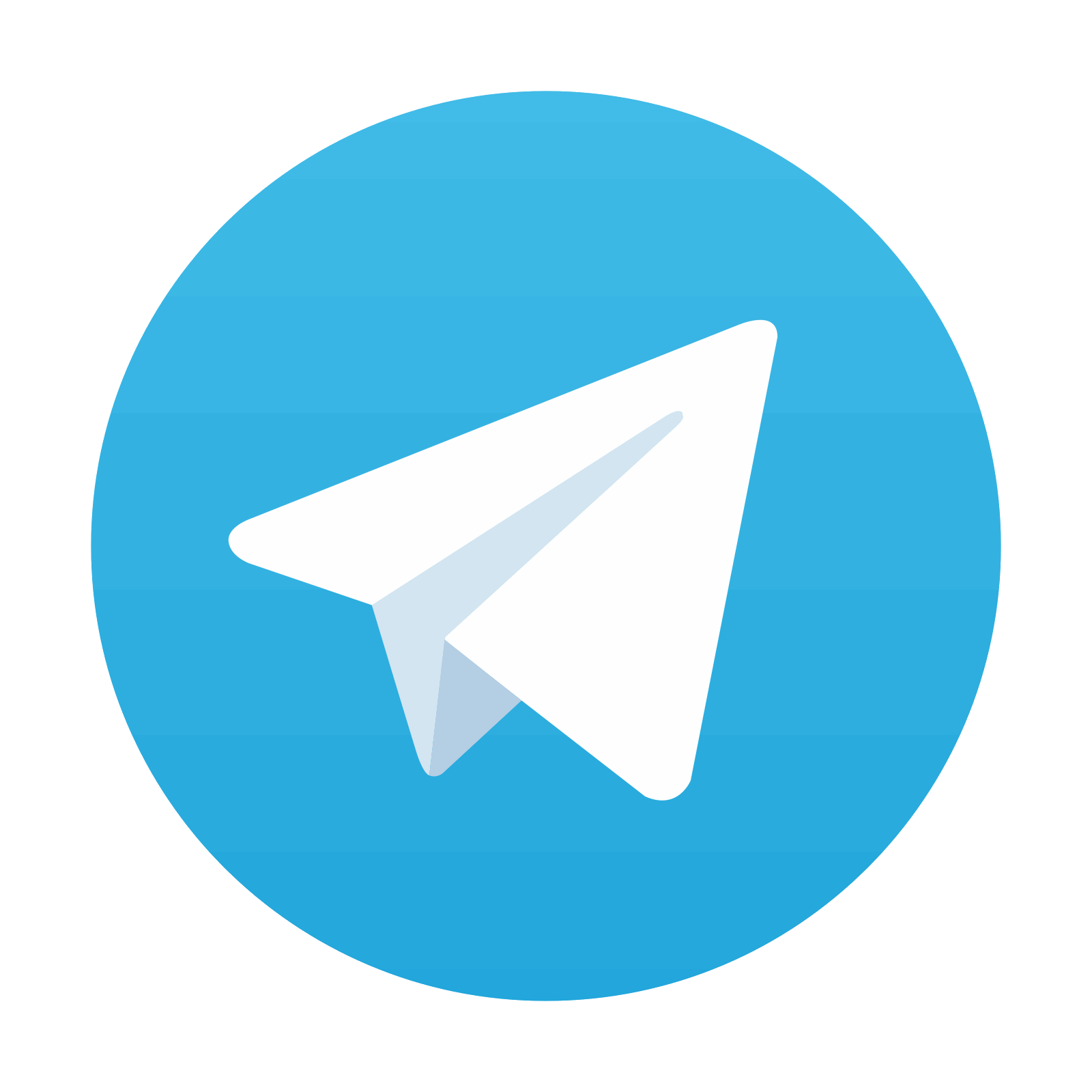
Stay updated, free articles. Join our Telegram channel

Full access? Get Clinical Tree
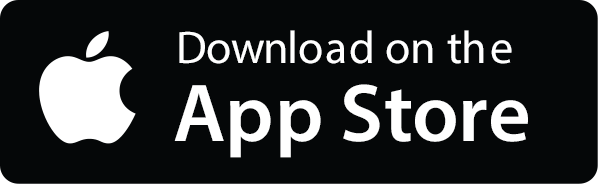
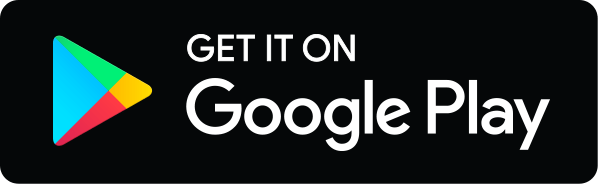