Degree of hypoxemia
Alveolar–arterial gradient (mmHg)
Pa02 (mmHg)
Mild
≥15
≥80
Moderate
≥15
≥60 and <80
Severe
≥15
≥50 and <60
Very severe
≥15
50
The following are hepatopulmonary syndrome (HPS) diagnostic criteria:
Chronic liver disease (portal hypertension with/without cirrhosis) |
AND |
Evidence for hypoxemia (Pa02 < 80 mmHg) a |
AND |
Evidence for intrapulmonary vascular dilatations (via contrast “bubble” echocardiogram) |
Screening for HPS
Given the frequency of HPS in patients with cirrhosis, a screening as well as diagnostic algorithm should be followed for the detection of HPS. Patients with cirrhosis can be screened for HPS via pulse oximetry. Patients with screening Sp02 values ≥96 % have Pa02 values consistently greater than 60 mmHg (Arguedas et al. 2007). Alternatively, all patients with cirrhosis presenting for transplant evaluation can undergo a room air arterial blood gas (ABG) in the sitting position. Patients with a sitting room air Pa02 < 80 mmHg can be then evaluated further with a bubble echo study to confirm HPS (Krowka et al. 2006b). One must remember that hypoxemia is a continuum and the prevalence of HPS in patients with cirrhosis will vary significantly based on the criteria used to define hypoxemia (A-a gradient vs. different Pa02 cutoff values) (Schenk et al. 2002) . In patients with low Sp02 or Pa02 values, a bubble echo study will confirm the presence of intrapulmonary shunting as well as exclude structural heart disease, intracardiac shunts, and coexistent pulmonary hypertension. The last point is very important because portopulmonary hypertension (POPH) can also present with significant hypoxemia but requires a markedly different diagnostic and therapeutic strategy as discussed elsewhere in this chapter (see main differences between POPH and HPS in Table 2). Room air and 100 % oxygen blood gases can be obtained in the supine and sitting position to quantify the degree of hypoxemia and estimate the shunt fraction (Rodriguez-Roisin and Krowka 2008). A chest X-ray will identify the presence of obvious pulmonary parenchymal disease. A chest CT is very helpful in clarifying and quantifying abnormalities seen on the chest X-ray and is crucial in patients with coexistent pulmonary parenchymal disease (such as emphysema or interstitial lung disease). Pulmonary function tests typically show a reduced diffusing capacity that is reduced in proportion to the severity of the hypoxemia. Nonspecific spirometry abnormalities including restriction may also be noted. Pulmonary angiography is not extensively used nowadays due to easy availability of chest CT scans, but can reveal pleural-based and pulmonary arteriovenous malformations along with a generalized increase in pulmonary capillary size and density giving a “spongy” appearance on angiography (Afessa et al. 1993). A summary of initial testing is presented in Table 3.
Table 2
Differences between portopulmonary hypertension (POPH) and hepatopulmonary syndrome (HPS)
POPH | HPS | |
---|---|---|
Primary pathophysiology | Pulmonary arterial hypertension (PAH) | Intrapulmonary shunting |
Pathology | PAH due to plexiform lesions, thrombosis, obliterative pulmonary arteriopathy | Intrapulmonary vascular dilatations (IPVDs) causing intrapulmonary shunting and consequent hypoxemia |
Severity of hypoxemia | + (typically mild) | +++ (mild to very severe depending on degree of shunting) |
Right ventricular function | Significantly elevated right ventricular systolic pressure (RVSP) with right ventricular (RV) dilatation/systolic dysfunction and low cardiac output | Normal or mildly elevated RVSP (due to high flow state) with normal RV size and function |
Clinical findings | Loud 2nd heart sound, systolic murmur, RV heave, lower extremity edema along with features of portal hypertension (varices, splenomegaly, ascites, etc.) | Clubbing, cyanosis, systolic flow murmur, platypnea, orthodeoxia along with signs of end-stage liver disease |
Treatment | Pulmonary arterial-specific therapy (e.g., ambrisentan, sildenafil, epoprostenol, etc.) | Supportive care and management of underlying liver disease until liver transplantation (which is curative for HPS) |
Is liver transplantation recommended/feasible? | Only in patients where the pulmonary hypertension is adequately controlled prior to transplantation | Recommended/feasible in all patients (even in severe hypoxemia) |
MELD exception points available | Yes | Yes |
Table 3
Initial workup of portal hypertension /cirrhosis patient with hypoxemia
Test | Rationale |
---|---|
Transthoracic echocardiography with “bubble” study | Confirms HPS (presence of intrapulmonary shunt) and excludes other entities (POPH, intracardiac shunting, valvular disease, left ventricular systolic/diastolic dysfunction) |
Pulmonary function testing with diffusing capacity | Identifies obstructive (e.g., COPD) and restrictive (e.g., interstitial lung disease) pulmonary disease and establishes degree of impairment |
Room air sitting and standing Pa02 | Establishes degree of hypoxemia |
100 % oxygen shunt study (sitting and standing) | Establishes degree of hypoxemia and shunting |
Chest X-ray and/or chest CT scan | Identifies other pulmonary pathologies (e.g., COPD, interstitial lung disease, chest wall and pleural disease) |
Overnight oximetry | Establishes need for nocturnal O2 supplementation. Also useful in identifying sleep apnea if clinically suspected |
Oxygen titration study | Establishes supplemental O2 needs at rest and during exercise |
Detecting Intrapulmonary Vascular Dilatations
The most sensitive method of detecting IPVDs in HPS is the contrast-enhanced transthoracic echocardiogram (bubble study). The most commonly used method involves injection of agitated saline via either a central or peripheral intravenous catheter. The saline bubbles are typically 10–90 μm in diameter and considerably larger than the diameter of normal pulmonary capillaries (8–15 μm) which do not allow passage into the left heart (Krowka et al. 2006b). However, IPVDs (typically ≥ 15 μm) allow free passage of these bubbles into the left heart resulting in the detection of an intrapulmonary shunt (Rodriguez-Roisin and Krowka 2008). In patients with an intracardiac shunt, bubbles typically appear in the left cardiac chambers within 1–2 cycles of their appearance in the right atrium. In a “positive” bubble study for intrapulmonary shunting, bubbles will appear in the left atrium 3–6 cardiac cycles after their first appearance in the right ventricle. The degree of shunting can be visually (semiquantitatively) estimated as being trivial, mild, moderate, or severe based on the quantity of bubbles passing through to the left heart. Although very sensitive for detecting IPVDs, the bubble study is not specific for the diagnosis of HPS, and many patients with liver cirrhosis may have some degree of intrapulmonary shunting and not otherwise qualify for a diagnosis of HPS due to lack of hypoxemia (Abrams et al. 1998). A more specific test appears to be the 99 m technetium-macroaggregated albumin perfusion lung scan (Abrams et al. 1998). A significantly abnormal shunt fraction (normal ≤6 %) is almost always associated with a clinical diagnosis of moderate to severe HPS (Abrams et al. 1998). As mentioned earlier, the 99 m technetium-macroaggregated scan can also be useful in quantifying the degree of hypoxemia due to intrapulmonary shunting versus coexistent intrinsic lung disease.
Management and Treatment
Medical therapies in HPS: Several medical treatments have been tried for HPS over the years in an attempt to manipulate the inducible nitric oxide synthase (iNOS) or endotoxin pathways (Miyamoto et al. 2010; Afessa et al. 1993; Schenk et al. 2000) (Schiller et al. 2011; Tanikella et al. 2008). Norfloxacin was shown not to be effective in one randomized blinded crossover trial in nine patients (Gupta et al. 2010). Anecdotal case reports have documented a beneficial response from inhaled nitric oxide (Durand et al. 1998) as well as inhaled iloprost (Krug et al. 2007). These agents probably work by redistributing ventilation perfusion imbalances in the lung by causing a generalized pulmonary vasodilatation. One of the more promising recent reports has been a randomized controlled trial of oral garlic in HPS (Nath et al. 2004). Patients in this study were followed over a period of 9–18 months and showed a significant reduction in the degree of hypoxemia. Partial or complete resolution of HPS was also seen in a substantial proportion of patients. Overall, medical therapies other than liver transplant have not achieved consistent results in large cohorts of patients, and these therapies should only be resorted to when other options do not exist in critically ill patients with refractory hypoxemia.
Liver transplantation: The first attempt at human liver transplantation was made in 1963, and the first successful transplantation occurred in 1967 (Starzl et al. 1982). Since then, the role of liver transplantation in HPS has undergone a significant evolution. In the 1980s, HPS was first considered to be an absolute (Van Thiel et al. 1984) and then a relative contraindication to LT (Maddrey and Van Thiel 1988). A series of case reports in the 1990s documented essentially complete reversal of intrapulmonary shunting and clubbing in a number of patients with severe HPS (Stoller 1990; Laberge et al. 1992; Schwarzenberg et al. 1993; Hobeika et al. 1994). Oxygenation normalized from severely low values and pulmonary diffusing capacity showed continued improvement even 2 years after transplantation in one report (Laberge et al. 1992). These initial reports led to a wave of subsequent studies that looked at post-LT outcomes and resolution of HPS in these patients (Gupta et al. 2010; Lange and Stoller 1995; Arguedas et al. 2003; Taille et al. 2003; Krowka et al. 2006b; Schiffer et al. 2006; Iyer et al. 2013). These studies are summarized in Table 4. Earlier series reported higher mortality rates for HPS patients post-LT (Schiffer et al. 2006). However, recent reports (Gupta et al. 2010; Iyer et al. 2013) challenge the notion that HPS is associated with higher post-LT mortality. Recent reports from our group (Iyer et al. 2013) and Gupta et al. (Gupta et al. 2010) have challenged the traditional notion that severely hypoxemic HPS patients have inferior post-LT outcomes (Fig. 1). A large number of patients in both these series (11/21) Gupta and (23/49) Iyer had Pa02 ≤ 50 mmHg. No increase in perioperative mortality was noted in either series in these patients. Further studies exploring this difficult subgroup of patients will likely be very influential in modifying current apprehensions regarding transplantation for these patients.
Study | N | Early mortalitya | Late mortalityb | Pre-LT Pa02 c |
---|---|---|---|---|
Scott et al. (1993) | 6 | 0 % | 0 % | 59 |
Hobeika et al. (1994) | 9 | 44 % | 0 % | 59 |
Fewtrell et al. (1994) | 8 | 13 % | 0 % | 83d |
Barbe et al. (1995) | 11 | 36 % | 0 %; 48 months f/u | 57 |
Egawa et al. (1999) | 21 | 10 % | 28 %; 12 months f/u | 57 |
Collisson et al. (2002) | 6 | 0 % | 50 %; 28 months f/u | 52 |
Taille et al. (2003) | 23 | 9 % | 22 %; 72 months f/u | 52 |
Arguedas et al. (2003) | 25 | 29 % | 0 %; 12 months f/u | 54 |
Schenk et al. (2003) | 7 | 0 % | 43 %; 24 months f/u | 75 |
Kim et al. (2004) | 13 | 8 % | 0 %; 90 days f/u | NR |
Krowka et al. (2004) | 32 | 17 % | no f/u beyond Tx hosp | 51 |
Schiffer et al. (2006) | 9 | 32 % | 0 %; 6 months f/u | 60 |
Deberaldini et al. (2008) | 25 | 32 % | 8 %; 48 months f/u | 75 |
Gupta et al. (2010) | 21 | 0 % | 5 %; 70 months f/u | 51 |
Iyer et al. (2013) | 49 | 10 % | 20 %; 120 months f/u | 58 |
Before 2002, organ allocation for LT was based on both disease severity and waitlist time. In 2002, implementation of standard MELD criteria standardized organ allocation across the country and prioritized severity of liver dysfunction over waitlist times. The understanding that patients with some diseases such as HPS, hepatocellular carcinoma, etc., were at a disadvantage with the new point allocation system leads to the development of MELD exception criteria. MELD exception points for HPS were formalized in 2006 and allowed for the granting of additional MELD points to HPS patients with a room air sitting Pa02 < 60 mmHg. Prior to 2006, extra MELD points were based on the requests of individual centers and were not standardized. Our center has published on LT outcomes in the MELD exception era and has shown excellent outcomes in these patients (Iyer et al. 2013). However, there continues to be some debate about the utility and implementation of MELD exception points for HPS (Goldberg et al. 2014) .
Post-liver transplantation management: HPS patients require additional care in the posttransplant setting mainly as a result of persistent hypoxemia. Over the past two decades, tremendous strides have been made in the understanding of critical illness and lung protective ventilation. These changes have significantly improved the care of HPS patients in the postoperative period. Even severely hypoxemic patients can be safely managed using a variety of ventilatory support tools (Fig. 2). Our group reported on posttransplant care details on 32 HPS patients undergoing LT (Iyer et al. 2013). No patient required a tracheostomy for prolonged mechanical ventilation. The median ICU length of stay (LOS) was 2 days with a median hospital LOS of 14 days. Median duration of intubation and mechanical ventilation post-LT was 10 h (range 1–230 h). It is important to note that 13 of these 32 patients had very severe HPS with baseline Pa02 values <50 mmHg. Most importantly, post-LT survival was essentially identical between HPS and non-HPS patients in our cohort (Iyer et al. 2013). Based on contemporary experiences from our group and others, it appears that LT can be safely accomplished with excellent outcomes even in patients with profound baseline hypoxemia (Gupta et al. 2010; Iyer et al. 2013).
Resolution of HPS is almost universal post-LT. This has been confirmed across multiple studies from different centers (Gupta et al. 2010; Iyer et al. 2013). The time to resolution is varied, and it is generally thought that the cases with the most severe hypoxemia pre-transplant generally take the longest to resolve posttransplantation. Improvements in oxygenation generally precede improvements in pulmonary diffusing capacity (Laberge et al. 1992).
Development of POPH Post-LT in Patients with HPS
The coexistence of POPH and HPS as well as the occurrence of POPH post-LT in HPS patients has been reported in the literature (Atz and Wessel 1999; Galie et al. 2004; Aucejo et al. 2006; Pham et al. 2010). It is important to note that HPS patients have been shown to have features of right atrial and ventricular enlargement along with RV hypertrophy (Fallon et al. 2008). Activated CD 68 (+) macrophages in the pulmonary circulation may be the common pathophysiological link for the development of both HPS and POPH given the angioproliferative, vasodilatory, and prothrombotic cytokines produced by them (Thenappan et al. 2011). One explanation for the emergence of POPH post-LT may be the fact that nitric oxide-mediated vasodilatation is reversed post-LT due to the presence of a functioning liver. This then leads to the emergence of unopposed vasoconstriction and development of PAH in a pulmonary circulation that had been previously vasodilated by nitric oxide. Clinicians should remain vigilant about this possibility and should appropriately investigate persistent or new dyspnea or hypoxemia with an echocardiogram or cardiac catheterization.
Portopulmonary Hypertension (POPH)
Portopulmonary hypertension (POPH) is a serious pulmonary vascular complication of portal hypertension that is associated with significant mortality and is related to neither the etiology of liver disease nor the severity of portal hypertension. The first clinical and pathological report of what we now know as POPH was provided by Mantz and Craige in 1951 (Mantz and Craige 1951). These authors described necropsy results of a 53-year-old female with spontaneous portacaval shunt (due to a probable congenital portal vein narrowing) that originated at the confluence of the portal, splenic, and mesenteric vein, coursed through to mediastinum, and was lined by varying amounts of thrombus thought to have embolized via the innominate vein into the right heart and pulmonary arteries. In addition to embolized small pulmonary arteries, an extreme endothelial proliferation and recanalization process was documented (Mantz and Craige 1951). Since the 1980s, enhanced recognition and renewed importance of POPH have evolved with the evolution of liver transplantation (LT) and potential outcomes associated with POPH. Specific screening recommendations and diagnostic criteria are now clearly defined for this syndrome. Despite the lack of randomized controlled trials for its medical treatment, extrapolation of the therapeutic advances in treating pulmonary arterial hypertension with specific effects in POPH has stimulated ongoing interest and importance in this syndrome.
Definition of POPH
Portopulmonary hypertension (POPH) is defined as pulmonary arterial hypertension (PAH) that occurs as a consequence of portal hypertension with or without cirrhosis (Simonneau et al. 2013). During the 5th World Symposium on Pulmonary Hypertension, POPH was included in group 1 because of its pathological and hemodynamic similarities with other causes of precapillary pulmonary hypertension (Table 5) (Simonneau et al. 2013). In the presence of documented portal hypertension, POPH is defined according to the following hemodynamic data obtained during a right heart catheterization (RHC):
- A.
Mean pulmonary artery pressure (MPAP) ≥25 mmHg
- B.
Pulmonary vascular resistance (PVR) ≥ 240 dyn/s/cm−5 or ≥3 Wood units
- C.
Pulmonary arterial wedge pressure (PAWP) ≤15 mmHg
Table 5
Updated classification of pulmonary hypertension by the 5th World Symposium on Pulmonary Hypertension (Simonneau et al. 2013)
1. Pulmonary arterial hypertension
1.1 Idiopathic PAH
1.2 Heritable PAH
1.2.1 BMPR2
1.2.2 ALK-1, ENG, SMAD9, CAV1, KCNK3
1.2.3 Unknown
1.3 Drug and toxin induced
1.4 Associated with:
1.4.1 Connective tissue disease
1.4.2 HIV infection
1.4.3 Portal hypertension
1.4.4 Congenital heart diseases
1.4.5 Schistosomiasis
1′ Pulmonary veno-occlusive disease and/or pulmonary capillary hemangiomatosis
1′′. Persistent pulmonary hypertension of the newborn (PPHN)
2. Pulmonary hypertension due to left heart disease
2.1 Left ventricular systolic dysfunction
2.2 Left ventricular diastolic dysfunction
2.3 Valvular disease
2.4 Congenital/acquired left heart inflow/outflow tract obstruction and congenital cardiomyopathies
3. Pulmonary hypertension due to lung diseases and/or hypoxia
3.1 Chronic obstructive pulmonary disease
3.2 Interstitial lung disease
3.3 Other pulmonary diseases with mixed restrictive and obstructive pattern
3.4 Sleep-disordered breathing
3.5 Alveolar hypoventilation disorders
3.6 Chronic exposure to high altitude
3.7 Developmental lung diseases
4. Chronic thromboembolic pulmonary hypertension (CTEPH)
5. Pulmonary hypertension with unclear multifactorial mechanisms
5.1 Hematologic disorders: chronic hemolytic anemia, myeloproliferative disorders, splenectomy
5.2 Systemic disorders: sarcoidosis, pulmonary histiocytosis, lymphangioleiomyomatosis
5.3 Metabolic disorders: glycogen storage disease, Gaucher disease, thyroid disorders
5.4 Others: tumoral obstruction, fibrosing mediastinitis, chronic renal failure, segmental PH
It has been described that approximately 20 % of patients with cirrhosis have a moderate increase in pulmonary arterial pressures as assessed by echocardiography; however, only a fraction of these patients truly have POPH (Castro et al. 1996). This observation is likely related to other pulmonary hemodynamic patterns encountered in advanced liver disease such as excess volume due to fluid retention (with increased left-sided filling pressures) or a hyperdynamic state with increased cardiac output (Rodriguez-Roisin et al. 2004; Krowka et al. 2006b). Distinguishing these patterns by RHC is important in order to provide correct management (Krowka and Edwards 2000) (Table 6). Impaired sodium and water handling is a common problem in chronic liver disease patients, and excess volume due to fluid retention is very often seen in these patients and is reflected by increased PAWP during RHC. An increase in both PVR and PAWP can confuse the interpretation of pulmonary hemodynamics (Krowka et al. 2006b), a phenomenon that is seen in up to 25 % patients with POPH (Krowka 2012). In these cases, true precapillary pulmonary hypertension (POPH) is manifest by an increased transpulmonary gradient (MPAP-PAWP > 12 mmHg). These patients should not be excluded from the diagnosis of POPH due to an elevated PAWP alone.
Table 6
Pulmonary hemodynamic patterns documented by right heart catheterization in advanced liver disease
Mean pulmonary artery pressure (normal 9–18 mmHg) | Pulmonary vascular resistance (normal <2 Wood units) | Cardiac output (normal 4.0–8.0 L/min) | Pulmonary artery wedge pressure (normal 6–12 mmHg) | |
---|---|---|---|---|
Vasoconstriction with vasoproliferation (POPH) | Elevated | Elevated | Low or normal | Normal |
Fluid overload or pulmonary venous hypertension (excess volume) | Elevated | Normal or elevated | Elevateda | Elevated |
Hyperdynamic circulatory state (high flow) | Elevated | Normal | Elevated | Normal |
POPH should be distinguished from hepatopulmonary syndrome (HPS) (Rodriguez-Roisin et al. 2004; Rodriguez-Roisin and Krowka 2008). In HPS, arterial hypoxemia (which may be severe) is caused by intrapulmonary vascular dilatations, as opposed to vascular obstructions of POPH. HPS presents with normal PVR and a high flow state characterized by increased cardiac output (CO). This distinction is important if liver transplantation (LT) is being considered due to differences in risk, treatment options, and outcomes (Rodriguez-Roisin and Krowka 2008).
Epidemiology of POPH
This pulmonary vascular condition affects predominantly adults and is notably rare in the pediatric age group (Krowka 2012). Autoimmune liver disorders and female gender are more frequently associated with POPH (Kawut et al. 2008). Poor correlation exists between the severity of POPH and the degree of liver dysfunction as characterized by the Child–Turcotte–Pugh (CTP) or Model for End-Stage Liver Disease (MELD) scores (Hadengue et al. 1991; Krowka 2012). When compared to idiopathic pulmonary arterial hypertension (IPAH) hemodynamics, POPH is characterized by higher CO and less severity as measured by MPAP and PVR (Kuo et al. 1997b; Krowka et al. 2012; Chiva et al. 2014).
The term POPH was apparently first coined by Yoshida et al. in 1993, as they described the first case of POPH to undergo successful LT (Yoshida et al. 1993). Subsequently, several small series and case reports with autopsy results have described pulmonary arterial obstruction and pulmonary plexogenic arteriopathy (Naeye 1960; Lebrec et al. 1979; Matsubara et al. 1984; Edwards et al. 1987; Sankey et al. 1993). An unselected series of 17,901 autopsies revealed that PAH was five times more likely in cirrhotic patients than those without liver disease (McDonnell et al. 1983). Within the 1981–1987 NIH national registry of “primary” pulmonary hypertension from 32 centers reported by Rich (Rich et al. 1987), additional analyses by Groves concluded that 8.3 % likely had POPH (17/204; 187 had primary pulmonary hypertension) (Groves et al. 1990). Hadengue reported the largest prospective study of patients with portal hypertension (n = 507) in which portopulmonary hemodynamic measurements concluded that 2 % had POPH (Hadengue et al. 1991).
Prospective studies have demonstrated that POPH is a relatively common condition among LT candidates and in pulmonary hypertension registries. In the French pulmonary hypertension registry experience over a 12-month period (2002–2003), Humbert reported a 10.4 % frequency of POPH (70/674) from 17 university hospitals (Humbert et al. 2006). In the United States, the REVEAL (Registry to Evaluate Early and Long-Term Pulmonary Arterial Hypertension Disease Management) registry documented a 5.3 % POPH frequency (174/3,525), in which there were 68 % prevalent and 32 % incident cases satisfying the criteria of a MPAP ≥ 25 mmHg, PVR ≥ 240 dyn.s.cm−5, and PAWP ≤ 15 mmHg (Krowka et al. 2012). Following slightly different PVR diagnostic criteria as part of outpatient RHC diagnostic assessments, the largest POPH-liver transplant center experiences reported to date are as follows: 8.5 % (Baylor Dallas, 102/1,205; PVR > 120 dyn.s.cm−5), 6.1 % (Clichy, France 10/165; PVR > 120 dyn.s.cm−5), and 5.3 % (Mayo Clinic 66/1,235; PVR > 240 dyn.s.cm−5) (Ramsay et al. 1997; Colle et al. 2003; Krowka et al. 2006b).
Pathophysiology and Pathogenesis
The pulmonary histopathology of POPH individuals is indistinguishable from other PAH phenotypes (Edwards et al. 1987; Krowka and Edwards 2000). Based upon autopsy and lung explant studies, POPH is characterized by a spectrum of obstructive and remodeling changes in the pulmonary arterial bed. Initially, medial hypertrophy with smooth muscle proliferation and a transition to myofibroblasts has been documented. As this proliferative pathologic process advances, plexogenic arteriopathy eventually develops (Edwards et al. 1987; Krowka and Edwards 2000).
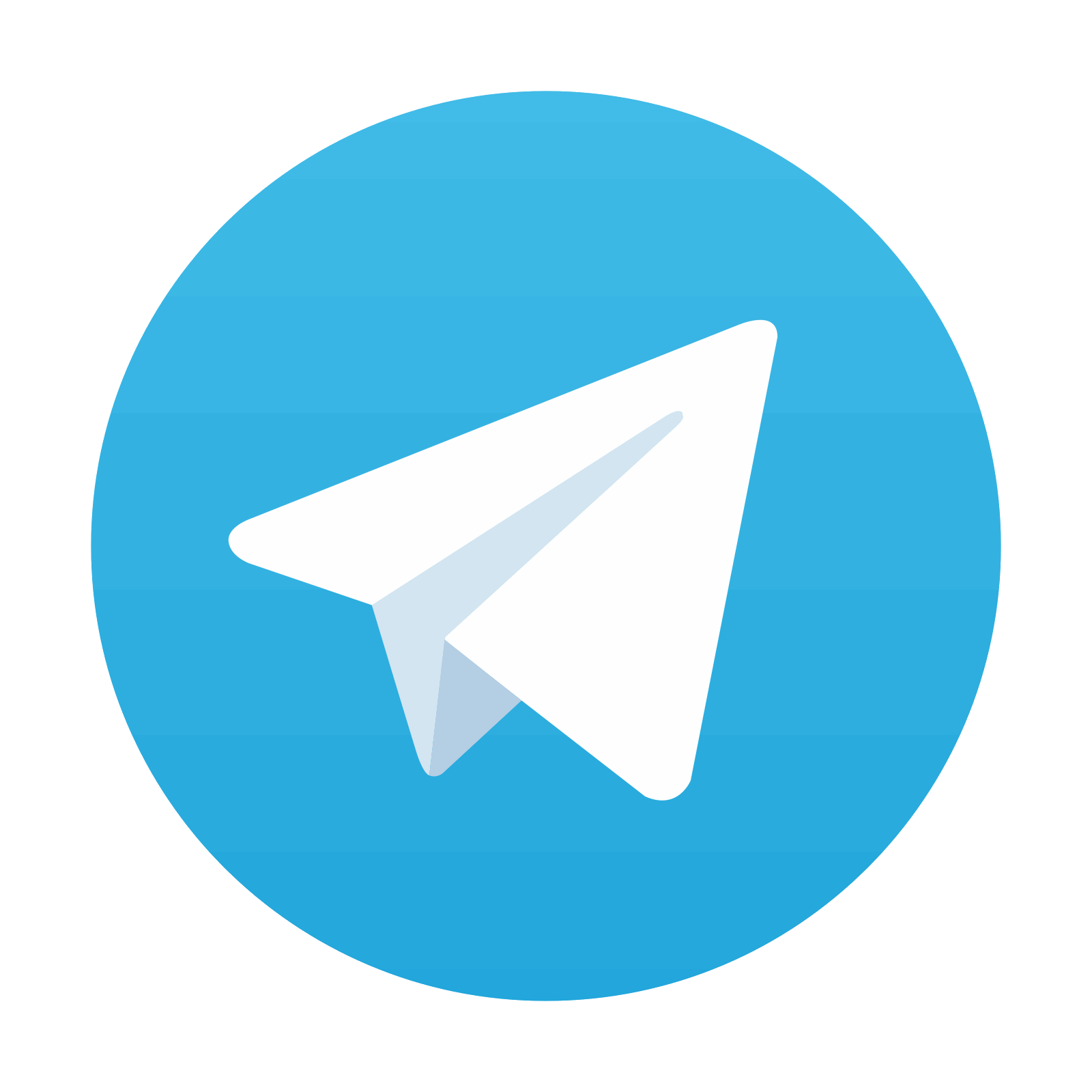
Stay updated, free articles. Join our Telegram channel

Full access? Get Clinical Tree
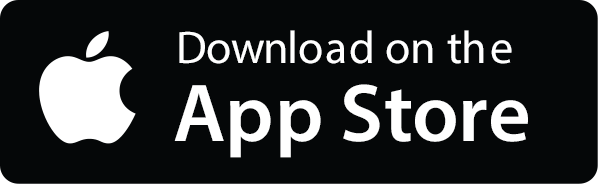
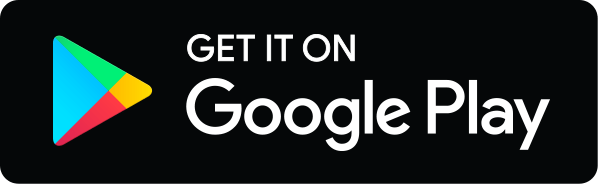