Epidemiology
Ventricular septal defect (VSD) is the most common noncyanotic cardiac anomaly (20 percent of all malformations); it is present in over 50 percent of children with complex congenital heart disease. VSDs occur in 0.5 of 1000 live births, and 5 percent are related to chromosomal syndromes.
Morphology
VSDs vary in size, number, and location along septum. They are classified as (1) perimembranous (PM, most common), (2) inlet type, (3) nonmuscular outlet, and (4) muscular. The conduction system and aortic valve leaflets are in anatomic proximity and are at risk during repair.
Pathophysiology
Degree and direction of shunting between the right and left ventricles depend on size (restrictive or nonrestrictive) and balance between the systemic vascular resistance (SVR) and pulmonary vascular resistance (PVR). Aortic regurgitation can ensue as a consequence of leaflet prolapse through the defect.
Clinical features
Small, restrictive defects are often asymptomatic. Symptoms of overcirculation are present in unrestrictive VSDs; with the development of irreversible pulmonary vascular changes in untreated large shunts, fixed pulmonary hypertension and cyanosis develop (Eisenmenger syndrome).
Diagnosis
Echocardiography accurately defines the anatomy of VSD and associated lesions. Cardiac catheterization is rarely required.
Treatment
Timing of closure is indicated by the degree of shunting and aortic valve involvement, and is most typically performed in infancy. Transatrial or transventricular approaches are most commonly utilized, with minimal morbidity and mortality. Percutaneous or periventricular device closure is still experimental, while a staged approach (with pulmonary arterial banding and delayed complete repair) is now considered only in selected cases.
A ventricular septal defect (VSD) is a deficiency in the ventricular septum that can vary in size, number, or location on the septum. All three determine its physiology, while the location alone determines its nomenclature. This is the most commonly recognized congenital heart defect excluding bicuspid aortic valve. Approximately 20 percent of patients with cardiac malformations have isolated VSDs and, if one includes VSDs in combination with other defects, VSDs are diagnosed in 50 percent of all patients with congenital heart disease.1 VSDs occur at a rate of 0.5 per 1000 live births and in 4.5 to 7 of 1000 premature infants,1,2 with a slightly higher prevalence in females (56 percent).3 About 5 percent of VSDs are related to chromosomal syndromes (such as 22q11 deletion and trisomy 21), in which VSD is the most common cardiac defect identified.4
In 1891, Dupren coined the term Maladie de Roger in honor of Henry-Louis Roger, who first described the clinical and pathologic findings of a VSD in 1879.5 Eisenmenger then chronicled the natural history of an unrestrictive VSD by his account of the postmortem findings in a cyanotic patient who died at age 32 with a large VSD, a severely hypertrophied right ventricle (RV), pulmonary valve (PV) and tricuspid valve (TV) insufficiency, and thickened pulmonary arteries (PAs).6 However, the term Eisenmenger syndrome was not introduced until Abbott delineated the pathophysiology of a VSD in the 1930s.7
Muller was the first to surgically address a VSD in 1952 by placement of a pulmonary artery band (PAB).8 Lillehei, using controlled cross-circulation (see Chapter 58), was the first to perform a VSD repair in 1954.9 DuShane reported transventricular repair in 1956, while a transatrial approach was introduced the following year by Stirling.10,11 Truex’s description in 1958 of the atrioventricular node (AVN) and the conduction pathway in patients with VSDs is an integral part of all modern surgical techniques of VSD closure.12 Kirklin and associates established in 1961 the ability to repair VSDs in small infants, therefore avoiding the two-staged approach of banding of the PA followed by VSD closure.13
In order to comprehend the different nomenclatures that are used for VSDs and the approaches and techniques for repairing this anomaly, one must understand the anatomy of the RV, the conduction system, and the TV.
The TV has three leaflets: septal, anterior, and posterior (Fig. 65-1). The posterior papillary muscles, which are located on the inferior wall of the RV near the septum, give rise to the chordae of the septal and posterior leaflets. The anterior papillary muscle gives rise to the chordae of the anterior and posterior leaflets. It is anchored at the acute margin of the RV and fuses with the RV muscle to become the moderator band, which travels inferiorly and becomes the septal band (alternatively termed trabecula septomarginalis or septomarginal trabeculation), which travels toward the RV outflow tract. The septal band then divides into its posterior and anterior arms. Between these two arms is the infundibular septum, also known as the conal, outlet, or supracristal septum. The medial papillary muscle (the muscle of Lancisi), which gives chordae to the anterior and septal leaflets of the TV, is most prominent and identifiable during infancy and attaches to the septal band or its posterior arm. The parietal band is the continuation of the septal band’s posterior arm anteriorly onto the RV free wall. The parietal band and both arms of the septal band join to form the supraventricular crest (crista supraventricularis), the C-shaped nonobstructive entrance into the outlet region (subpulmonary conus) of the RV. This muscle shelf between the TV and PV creates PV–TV discontinuity.
Figure 65-1
Anterior view of the right ventricle in the anatomic position, as seen after removal of the anterior wall. *, moderator band; A, anterior leaflet of the tricuspid valve; IS, infundibular septum; M, medial papillary muscle of Lancisi; PB, parietal band; PV, pulmonary valve; RAA, right atrial appendage; RCA, right coronary artery; RV, trabecular portion of the right ventricle; SB, septal band; TV, tricuspid valve. (Reproduced with permission from Fuster V, Walsh RA, Harrington RA. Hurst’s the Heart, 13th ed. www.accessmedicine.com. Copyright The McGraw-Hill Companies, Inc., all rights reserved.)

It is essential to know the location of the AVN and the bundle of His in order to perform safe closure of a VSD. The AVN is located in the triangle of Koch (formed by the tendon of Todaro, the ostium of the coronary sinus, and the septal leaflet of the TV). More precisely, it is in the muscular region right below the triangle’s apex and directly on the right atrial side of the central fibrous body. From another perspective, the AVN is under the nadir of the noncoronary (posterior) cusp of the aortic valve (Fig. 65-2). The AV bundle (bundle of His) arises from the AVN and exits at the apex of the triangle of Koch, passing to the ventricular side through the right aspect of the central fibrous body. At this point, the bundle is on the posteroinferior margin of the membranous septum, which lies just posterior to the commissure of the septal and anterior leaflets of the TV (Fig. 65-3). The bundle penetrates the ventricular septum and continues on the left ventricular (LV) side in 75 percent of patients. Whether on the right or left, it courses along the inferior border of the membranous septum and begins to give off fibers to the left bundle branch over a distance of 1 to 2 cm. When the membranous septum is intact, this area is just below and to the left of the commissure between noncoronary and right coronary cusps of the aortic valve. In considering the bundle from the aortic valve perspective, one should imagine its path as coursing a few millimeters below the area between the right-noncoronary (posterior in Fig. 65-2) commissure and the nadir of the right coronary sinus. The remaining fibers of the bundle, which now surface to the anteroinferior border of the membranous septum, become the right bundle branch. The left bundle branch fans out over the septum while the right bundle branch courses as a single radiation (Fig. 65-3). From the anteroinferior border of the membranous septum, the right bundle passes below the muscle of Lancisi and then to the inferior borders of the septal and moderator bands until it reaches the anterior papillary muscle, where it disperses to innervate the RV. Therefore, the bundle of His is most often in harm’s way during operations on a perimembranous (PM) VSD. The bundle of His runs along a PM VSD’s inferior border from where it penetrates the TV annulus to the most inferior papillary muscle on the VSD’s muscular rim (the muscle of Lancisi) (Fig. 65-4). The fact that the AVN and bundle of His are specialized myocytes and thus exist only in muscular tissue and not in fibrous tissue is an important fact to consider when placing sutures for VSD repair. The conduction system can also be at risk at the superior edge of a muscular inlet VSD, where the conduction system runs in the muscle between the VSD and the membranous septum.
Figure 65-2
A view of the atrioventricular conduction system from the left ventricle and its relationship to the membranous septum and the aortic valve. AVN, atrioventricular node; MS, membranous septum; fasc, fasciculus; RBB, right bundle branch; LBB, left bundle branch. (From Titus JL. Normal anatomy of the human cardiac conduction system. Mayo Clin Proc 1973;48:24–30. With permission.)

Figure 65-3
Depiction of the atrioventricular node and its course on the inferior border of a perimembranous VSD; viewed from a right atrial surgical approach. (Drawing by Rachid Idriss. Used with the artist’s permission, who reserves all rights. The drawing has not been modified; however, the labeling is that of the authors.)

Figure 65-4
Anatomy of the atrioventricular conduction system. A. The atrioventricular bundle and its right branch dissected in the right ventricle. B. The atrioventricular bundle and its left branch dissected in the left ventricle. (From Sobotta. Atlas der Anatomie des Menschen, 16th ed. Munich: Urban & Schwarzenberg, 1963. With permission.)

There are many classifications of VSDs that have been proposed and used; our preference is a modification of the classic Anderson classification. However, to make our classification useful to the reader, we will compare other terms and classification to this terminology.
The ventricular septum can be sectioned into four regions: (1) the inlet septum, which is the area of the septum bounded by the attachments of the TV; (2) the muscular septum, which is the area from apex to the crista supraventricularis outside these attachments; (3) the outlet septum, which is the area from the crista supraventricularis to the PV, and (4) the PM septum, which is quite small and lies under the commissure of the anterior and septal leaflets of the TV. The muscular region is further subdivided into anterior, posterior, apical, and midmuscular regions. The Anderson classification names VSDs according to the region in which they are located. However, from a surgical perspective, one wants to know not only the location of the defect but also its relation to the conduction system. The latter can be transmitted to the surgeon by first defining whether the defect is PM (an absence of the membranous septum) or not (all other types of defects). This automatically tells the surgeon whether or not the conduction system is remote, and if not remote, where it is (inferior to a PM defect for a d-looped heart and superior to a PM defect for an l-looped heart, see Chapter 75). The exception to this rule is an inlet VSD, which is discussed below. One can also describe the type of PM VSDs by the areas defined by Anderson. Therefore, a PM VSD can be one with muscular extension, outlet extension, inlet extension, or any combination. Non-PM VSDs can be muscular in type (outlet, inlet, anterior, posterior, apical, midmuscular) or outlet or inlet types bound by valve tissue (Fig. 65-5). The synonyms in other classifications for all the terms used above can be found in Table 65-1. The table lists all the different names by which a VSD within a certain region can be designated. The different names listed under a certain region are sometimes used imprecisely to denote any VSD in the area, but most of the terms are not interchangeable and refer to a very specific type of VSD. For example, some might use the terms subaortic and supracristal to refer to the same VSD; however, the former is a general term that refers to any VSD abutting the aortic valve, whereas the latter specifically refers to an outlet VSD abutting a semilunar valve.
Preferred Terminology | Synonyms |
---|---|
Perimembranous VSD | |
PM VSD with inlet extension | Membranous, infracristal, paramembranous |
PM VSD with outlet extension | Membranous, conoventricular, subaortic |
PM VSD with muscular extension | Membranous, infracristal, paramembranous |
Nonmuscular-outlet VSD | Conal, supracristal, subarterial, subaortic, subpulmonary, infundibular, intracristal, doubly committed, conalseptal, juxta-arterial |
Inlet VSD | Canal type, atrioventricular |
Muscular | |
Inlet | Canal type |
Outlet | Conal, supracristal, subarterial, infundibular, conalseptal |
Midmuscular VSD | Central |
Apical muscular VSD | — |
Anterior muscular VSD | Marginal |
Posterior muscular VSD | Inferior |
The PM VSD represents approximately 80 percent of all VSDs; the remaining 20 percent are evenly distributed among inlet, outlet, and muscular VSDs. A notable exception to this distribution is the almost 30 percent overall prevalence of outlet-type VSDs in the Asian population with VSDs.14 Small asymptomatic muscular VSDs, which are probably the most common heart defect (present in about 5 percent of all neonates), are grossly underestimated in these statistics, since the vast majority close in the first months of life without recognition.15
PM VSDs are intimately related to the TV and aortic valve. In fact, the absence of the PM septum often creates TV–aortic valve fibrous continuity. “Spontaneous closure” of PM VSDs usually occurs from partial or complete occlusion of the VSD by aneurysmal TV tissue. This aneurysmal tissue is formed from the sheer force of the left-to-right (L–R) shunt resulting in creation of fibrotic tissue, “accessory valve tissue,” and/or, perhaps, remnants of endocardial cushion tissue. Some have described a defect of just the atrioventricular septum causing a shunt from the left ventricle to the right atrium, known as a “Gerbode defect.”16 When seen on echocardiography, this almost always represents a PM VSD with a jet directed through a cleft in the TV or the septal–anterior commissure, giving the echocardiographic appearance of a Gerbode defect. A consequence of this physiologic shunting from the LV to the right atrium can be extensive right atrial enlargement and, if an atrial septal defect exists, streaming of the blood can possibly cause right-to-left atrial (LA) shunting with resulting cyanosis.
Malalignment of the infundibular septum is also associated with PM VSDs, usually with outlet extension. If one considers the infundibular septum as being in the coronal plane with the RV outflow tract above and the LV outflow tract below, then comprehension of the malaligned VSDs is more straightforward. In an anteriorly malaligned VSD, the infundibular septum is deviated anteriorly into the RV outflow tract, causing obstruction. The aortic valve also moves anteriorly, so that it overrides the ventricular septum. In a posteriorly malaligned VSD, the infundibular septum deviates posteriorly into the LV outflow tract, causing obstruction.
Outlet defects are often differentiated between those that are completely bound by muscle and those that are bounded on one side by aortic valve tissue (subaortic, juxta-arterial VSDs), by PV tissue (subpulmonary VSDs), or both (doubly-committed, subarterial VSDs). This differentiation is useful in terms of the pathophysiology. The nonmuscular outlet defects along with PM VSDs with outlet extension can both be associated with aortic valve insufficiency, characterized by lengthening and eventual prolapsing of the right coronary leaflet (outlet defect) or noncoronary leaflet (PM VSD). This valve tissue can partially or completely close the defect. The conduction system is remote to outlet defects.
Inlet defects are beneath the septal leaflet of the TV, just inferior and posterior to the membranous septum. These defects can be an atrioventricular septal defect or a muscular inlet defect. The former is an endocardial cushion defect, characterized by absence of the PM septum, atrioventricular valve abnormalities, and a conduction system traveling along the inferior aspect of the defect. A muscular inlet defect is a VSD that can be remote to the conduction system or have the conduction system border the defect superiorly.
Muscular VSDs can be multiple or appear to be so because of the overlying trabeculae in the RV. This topography and multiplicity may cause difficulty in closure of these defects. When these impediments become prohibitive to conventional surgery, the term “Swiss cheese septum” is usually used to describe them. However, it should be noted that some believe that a Swiss cheese septum is actually an entity distinct from multiple VSDs. The morphology of the Swiss cheese septum is believed to originate from septal noncompaction during embryologic development; thus, unlike a group of muscular VSDs, Swiss cheese defects cannot close spontaneously.17,18
The pathophysiology of a VSD is determined by the size of the defect and its location. If the defect is smaller than the aortic annulus (a restrictive VSD), then the shunt’s direction and volume is determined by the difference in systolic pressures of the ventricles. If the defect is larger than the aortic valve, then the defect is nonrestrictive and the pressure in the ventricles should equalize. The direction and volume of this shunt is determined by the difference in PVR and systemic vascular resistance (SVR).
Restrictive VSDs can be further categorized as small or moderate in size. A small VSD has a large resistance to flow, resulting in a small L–R shunt, and the pulmonary vasculature is well protected. The small shunt volume does not increase ventricular work or volume, and the LA and LV tend not to dilate. The large pressure gradient across the small VSD favors L–R flow throughout the cardiac cycle, which can produce a continuous murmur. Moderate-sized defects remain restrictive but allow a large enough L–R shunt to cause left-sided volume overload, characterized by LA and LV dilation. The pulmonary vasculature remains protected; however, some pulmonary hypertension can exist, which will cause RV pressure and work to increase. This results in mild RV hypertrophy.
Nonrestrictive VSDs allow for RV and LV to have equal pressures, with the determination of flow across the defect resulting from outflow resistance. In the LV, this is determined by SVR or by LV outflow tract anomalies (subaortic stenosis, aortic stenosis, coarctation, etc.). In the RV, this is determined by PVR or by RV outflow tract anomalies (PV stenosis, tetralogy of Fallot, etc.). PVR is high at birth and lessens the amount of L–R shunt; however, the PVR naturally decreases as the pulmonary vascular bed matures. The decline in PVR occurs mostly over the first few days, but the process is not completed until 2 to 6 weeks after birth.19,20 The thinning of the vascular media, the enlargement and proliferation of the peripheral PAs, and the regression of the PA’s perinatal muscularity mark the normal maturation of the pulmonary vessels. However, a large L–R shunt disturbs the growth and remodeling of the pulmonary vasculature, so that medial hypertrophy develops, muscularization of PA’s persists, and the size and number of peripheral PAs is reduced. These structural changes are the basis of pulmonary vascular disease.21,22 Any patient with a nonrestrictive VSD and no RV outflow tract obstruction has pulmonary hypertension, but this does not necessarily indicate that the patient has pulmonary vascular disease. The latter is a disease state of the pulmonary vasculature correlating to specific pathologic changes in the PAs, while pulmonary hypertension is simply a hemodynamic state of the PAs at any given time. A nonrestrictive VSD is characterized by a large L–R shunt, which will cause volume overload of the LA and LV, resulting in significant dilation of these chambers. This shunt will also result in pulmonary hypertension, which in time will cause RV hypertrophy and increasing PVR. As the PVR increases, the L–R shunt decreases. When end-stage pulmonary vascular disease ensues, the shunt can even reverse direction to a R–L shunt (i.e., Eisenmenger syndrome). Reversal of shunting and cyanosis rarely present in children below age 5 with isolated VSDs and is usually seen in adolescents and adults.
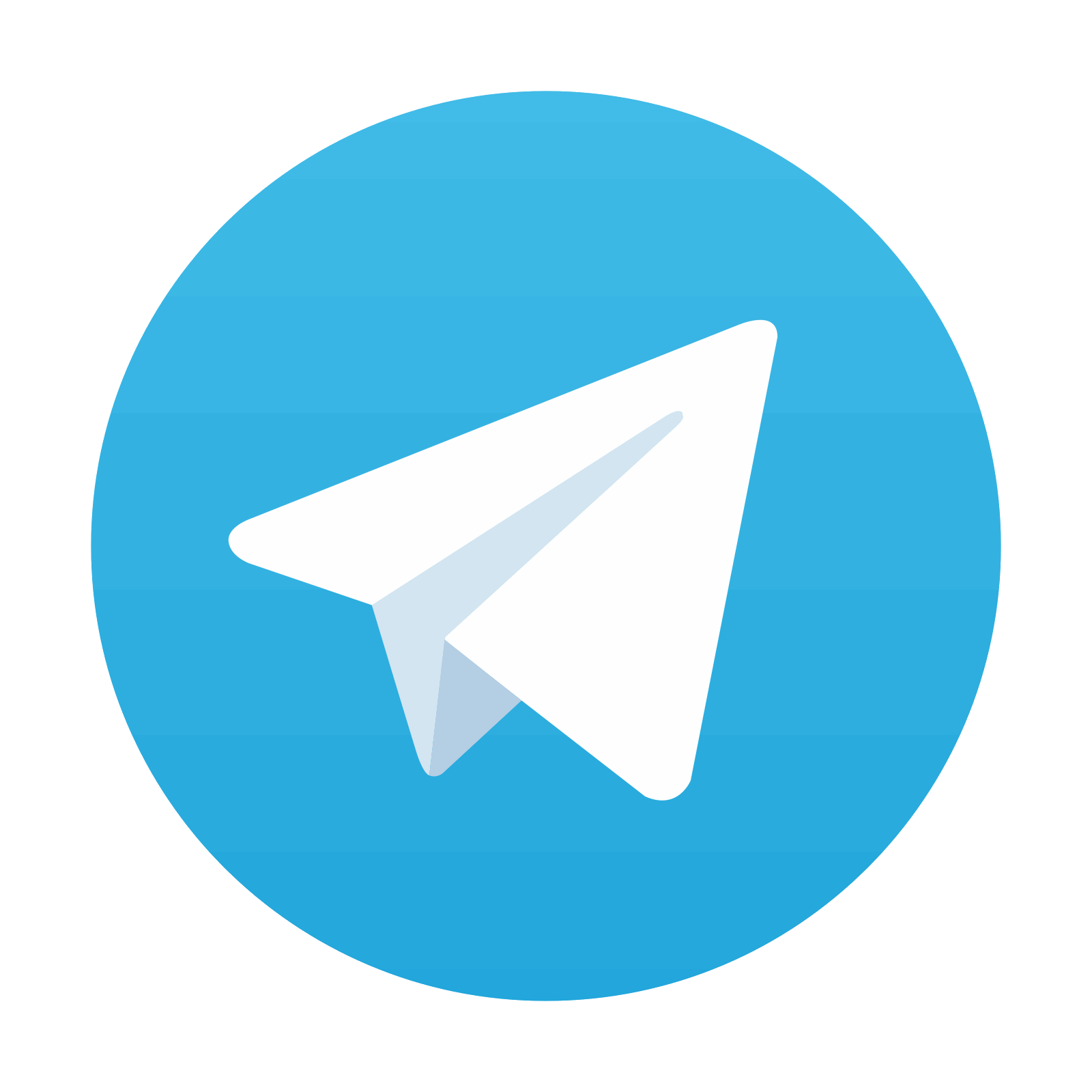
Stay updated, free articles. Join our Telegram channel

Full access? Get Clinical Tree
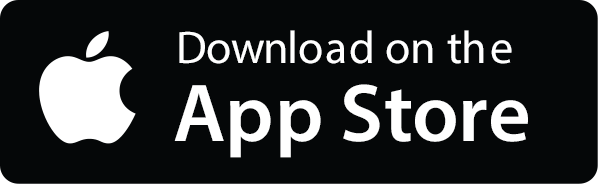
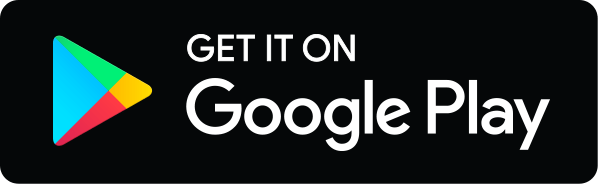