Epidemiology
Approximately 100,000 aortic valve replacement procedures have been performed in the United States in the last 10 years. Most patients present with aortic valve pathology that requires replacement between ages 60 and 80. Replacement for aortic stenosis is performed much more commonly (85 percent) than is replacement for aortic insufficiency.
Pathophysiology
With aortic stenosis, there is a decrease in the effective valve orifice area, resulting in progressive obstruction of the left ventricle. The ventricle adapts through concentric hypertrophy, which increases diastolic stiffness and impairs the efficacy of diastolic coronary blood flow. Diastolic dysfunction precedes systolic dysfunction. With aortic insufficiency, gradual ventricular dilatation occurs, causing an increase in wall stress. This, in conjunction with the decrease in diastolic pressure, reduces diastolic coronary blood flow. With continued dilatation, fibrosis occurs, increasing ventricular stiffness and impairing diastolic relaxation. Eventually, the preload reserve of the ventricle is reached and further dilatation and fibrosis result in a decline in systolic function that may not be recoverable after aortic valve replacement.
Clinical features
Aortic stenosis patients complain of combinations of symptoms of chest pain, syncope, and congestive heart failure. The life expectancy of patients with untreated aortic stenosis who present with angina is 50 percent at 5 years, 50 percent at 3 years with syncope, and 50 percent at 2 years with congestive heart failure symptoms. A small proportion of patients with aortic stenosis present with sudden death. Aortic insufficiency has a more insidious onset and usually is characterized by the development of slow congestive heart failure and fatigue symptoms. Angina pectoris and syncope are rare in these patients. These patients may be managed on medical therapy for protracted periods with an acceptable quality of life.
Diagnostics
The work-up for candidates for aortic valve replacement includes echocardiography to estimate ventricular function and the degree of stenosis or insufficiency and cardiac catheterization to measure cardiac output, calculate aortic valve area, and image the coronary arteries for significant lesions. A careful oral examination or dental consultation is very important to prevent postoperative prosthetic valve infection.
Treatment
Aortic valve replacement options include homograft, stented and stentless xenograft tissue, and mechanical valves. The decision to use each of these is dependent on patient age and valve preference, comorbid conditions, and contraindications to the use of anticoagulants. A variety of options are available to manage potential patient–prosthesis mismatch at the time of surgery.
Outcomes
The outcomes of tissue valve replacement show 80 to 93 percent freedom from structural valve failure at 10 to 14 years. Bleeding and thromboembolic events complicate mechanical valve replacement at a rate of approximately 4 percent per patient-year. Homograft valve replacements have the highest freedom from structural valve deterioration, with rates of 81 to 93 percent at 15 years. Survival after aortic valve replacement is dependent more on patient comorbidities than on the requirement for valve replacement. Postoperative complications specific for aortic valve replacement include complete heart block (1 percent) and perivalvular leaks (1–2 percent).
The first attempt to open a stenotic aortic valve in a patient was performed by Theodore Tuffier on July 13, 1912.1 This effort was accomplished by invaginating the ascending aortic wall with a finger and pushing the aorta through the valve. The 26-year-old male patient recovered and returned to his home in Belgium. Russell Brock dilated calcified aortic valves in humans in the late 1940s with instruments passed from the innominate artery or other arterial sources.2 His results were initially poor, and he3 as well as Bailey et al.4 later used different dilators in various approaches with somewhat better results. However, the mortality for these patients remained high. Horace Smithy, a South Carolina surgeon, suffered from severe rheumatic aortic stenosis (AS). Having achieved national acclaim for the development and successful use of a valvulotome for closed commissurotomy in patients with rheumatic mitral disease, he developed an aortic valvulotome5 and enlisted Dr. Alfred Blalock to perform the operation on him. Dr. Blalock agreed provided that he and Dr. Smithy perform the procedure together on another patient first. A patient was scheduled but unfortunately that patient died of ventricular fibrillation during induction of anesthesia. Shortly thereafter, Dr. Smithy died of complications related to his own severely stenotic aortic valve before the operation could be carried out.
In the early 1950s, Hufnagel6 and Campbell7 independently developed and implanted artificial valves in the descending thoracic aorta of dogs. In his paper published in 1954, Hufnagel reported a series of 23 patients starting in September of 1952 who had this operation for aortic insufficiency (AI).8 These efforts preceded the evolution of the most successful of the caged ball valves, the Starr–Edwards prosthesis. This valve, created by M. Lowell Edwards, a retired engineer, and Albert Starr, a cardiac surgeon in Portland, OR, was originally implanted in the mitral position in August of 1960. The first clinical Starr–Edwards aortic valve implantation was performed in September of 1961.9 Shortly after the introduction of the cage ball concept, a number of similar designs appeared in clinical use, which are beyond the scope of this chapter. However, excellent reviews are available.9,10
The concept of the tilting disc valve arose out of the concerns that cage ball valves were bulky and that the hemodynamics achieved were less than ideal. In an attempt to reduce the profile of these valves, a flat disc instead of a ball was used as an occluder. The earliest examples of the tilting disc concept were flat valves. These had a ring with a straight segment on which a disc was hinged much like the lid of a toilet seat. Other modifications included free-floating, polypropylene discs such as that introduced by Melrose and colleagues,11 and other interesting designs such as those produced by Water and McLeod in Edinburgh.12 In 1969, Bjork and Shiley produced a valve in which a free-floating disc was restrained by two low-profile M-shaped struts.13,14 The first implantation of the Bjork–Shiley prosthesis was in 1975. In 1978, the disc profile was changed from plano-convex to convexo-concave (C-C model) and struts were modified such that the disc moved 2.5 mm downstream as it tilted.14 In 1981, the valve ring was altered and a single heavier hook replaced the outlet strut, all of which was machined from one piece of metal with no welds (the monostrut valve).15 Other examples of tilting discs valves included the Lillehei–Caster, Omniscience, and Omni Carbon valves. Some were removed from use because of the high incidence of thrombotic obstruction,16 and others such as the Medtronic-Hall D16 Valve and the Bjork–Shiley C-C valves because of an unacceptable incidence of strut fractures.17
The earliest bileaflet mechanical valve designs were introduced by Gott and Daggett,18 Kalke and Lillehei,19 and Wada20 in the early- to mid-1960s. These prostheses failed due to mechanical malfunctions and high thrombogenicity. However, from these failures arose the realization that bileaflet prostheses had hemodynamic characteristics superior to that of all of the prostheses available at the time. In 1976, the bileaflet design was modified and manufactured entirely from pyrolytic carbon.21 Subsequent studies showed that the bileaflet design was very durable and that in addition, pyrolytic carbon was quite biocompatible and thromboresistant. The prototype pyrolytic carbon bileaflet valve, the St. Jude Medical Prosthesis, was first implanted clinically in 1977.22 This valve has undergone a number of modifications since the original standard St. Jude valve was introduced including the lower profile Hemodynamic Plus (HP) Valve,22 the Masters Valve (which allows internal rotation of the leaflets to get a better seating once the valve is implanted),23 and the Regent Valve (with a modified external profile to create an even larger internal orifice area per valve size).24 Other bileaflet valves are currently in use, such as the Carbomedics25 (released for clinical use in December, 1986) and Sorin Bicarbon (released in 1990) models.26
With the introduction of glutaraldehyde for the fixation of biological tissue by Carpentier and colleagues in 1969,27 the use of bioprosthetic material, both porcine valves and bovine pericardium, became feasible alternatives to mechanical designs for valve replacement. The University of Padua has the most extensive experience with the Hancock standard porcine bioprosthesis, which was first clinically implanted in the aortic position in March of 1970.28 The Hancock modified orifice (MO) valve was introduced in the wake of concerns about high postimplantation gradients with small valve sizes in the aortic position. This problem, due to the bulky muscle bar under the right coronary cusp of the valve, was reconciled with the MO design, a composite valve that replaces the right coronary cusp with a cusp from another valve that does not contain the muscle bar. The Carpentier–Edwards standard porcine bioprosthesis was introduced in 1975.29 Second-generation bioprostheses include the Carpentier–Edwards supra annular (SAV) porcine bioprosthesis, available since 1981,30 the Hancock II porcine bioprosthesis, which was introduced in 1982,31 and the Medtronic intact porcine bioprosthesis available since 1985.32 In general, these valves offer improved zero-pressure fixation that preserves subtle but important histological features of the valve leaflets, and antimineralization treatments to reduce calcific degeneration of the valve tissue. In addition, more flexible, lower profile stent materials and reduced sewing rings are employed to create a larger effective orifice area (EOA). Numerous other porcine bioprosthesis are under evaluation.
The first bovine pericardial bioprosthesis was the Ionescu–Shiley valve developed by Marian I. Ionescu and G. Wooler in 1971.33 In 1981, a low-profile model was released, but had a much higher rate of failure than the standard Ionescu valve. A final model III was implanted and used clinically before Shiley decided to cease the production of pericardial valves in October of 1977.34 The Mitroflow pericardial valve was designed by Siegel and Totten and first clinically implanted in 1982.35 The most common currently used pericardial valve, the Carpentier–Edwards pericardial valve, was first clinically implanted in Paris, France, in July 1980.36 This valve has undergone a number of modifications, including the Perimount RSR37 and Magna series.
The first clinically applicable bioprosthetic stentless aortic valve was the Toronto stentless porcine valve (SPV) as introduced by Dr. Tirone David at the University of Toronto in 1991.38 This valve offered an increased EOA of the valve by minimizing sewing ring and eliminating the supporting struts. The stentless valve design consisted of a native porcine valve reinforced by a small amount of fabric sewn to the patient’s annulus in a planar fashion followed by implantation of a small amount of porcine aorta around the valve commissures directly to the patient’s own native aorta. Other stentless valves currently in use include the Medtronic free-style porcine prosthesis39 and the Edwards Prima Plus porcine prosthesis.40 Other stentless valves, such as the ATS-3F Therapeutics stentless equine bioprosthesis,41 have been evaluated in clinical trials and are available for clinical use.
The first experimental animal studies involving homograft aortic tissue were performed by Lamb et al. in 1952, who implanted an allograft aortic valve into the descending aorta of the dog.42 This was followed within 3 years of the clinical reimplantation of the allograft aortic valve into the human descending aorta by Murray43 and subsequently by Heimbecker and associates in Toronto.44 The technique for subcoronary implantation of allograft tissue using the single suture method was reported by Duran and Gunning in 196245 and implemented the same year by Donald Ross46 in patients with a freeze-dried aortic valve, a feat performed shortly thereafter by Barrett–Boyes.47 Sterilization of homograft valves has undergone a number of changes from formaldehyde, chlorhexidine, propiolactone, methylene oxide, gamma radiation, and storage using a carbon dioxide freezer at –70°C. Currently, most homograft valves are cryopreserved with low-dose antibiotics.
The aortic valve develops from three swellings or ridges of endomyocardial tissue at the proximal orifice of the septating truncus arteriosus. These ridges become hollowed out and reshaped to form three thin-walled cusps or pockets at approximately the sixth to seventh week of development.48 The three aortic valve cusps are semilunar in shape and are named depending on whether or not the sinuses they subtend give rise to coronary artery ostia. Therefore, a right and a left coronary aortic cusp and one noncoronary cusp exist. The base (semilunar point of attachment to the aorta) of the leaflet is approximately one and a half times as long as its free margin. Portions of the noncoronary and a portion of the left coronary cusps are in continuity with the anterior leaflet of the mitral valve (the aorto-mitral continuity), which is an anatomical consideration that can become very important in the case of valve replacement for endocarditis. The aortic valve annulus is variable in its definition but is toughest in approximately the noncoronary section, constituting tissue between the left and right fibrous trigones of the heart. The ascent of the aortic attachment of the noncoronary cusp toward the right coronary/noncoronary commissure lies directly over the atrioventricular conduction system, which sits in the right fibrous trigone or central fibrous body of the heart. Sutures placed too deeply in this area during valve replacement will result in atrioventricular conduction block. It is important to understand that the aortic valve leaflets are attached to the aortic root in a scalloped fashion. Most techniques of valve replacement involve conversion of this scalloped aortic annulus to a relatively planar orientation, which can have important anatomic considerations. Certain stented bioprostheses are designed to maintain some of the scalloped architecture when implanted. Each aortic valve leaflet has a small nodular excrescence at its midportion called the node of Arantius or the nodulus Arantii.49
An aortic valve leaflet has three layers: the fibrosa or arteriosa (arterial side), the spongiosa (middle), and the ventricularis (ventricular side). These layers are composed of varying proportions of elastin, collagen and glycosaminoglycans. The outer layers of the valve cusps are covered in endothelial cells oriented perpendicular to the direction of blood flow.50
Aortic valve replacement (AVR) is performed in patients in whom there is an underlying morphologic or anatomic abnormality of the aortic valve independent of its supporting structures, which causes it to become stenotic or regurgitant.
AS typically arises as a result of degenerative calcification in the elderly or as a result of rheumatic degeneration in those individuals that have a history of rheumatic fever. Certain congenital conditions also predispose to AS. The most common of these is congenital bicuspid aortic valves, which occur in approximately 5 percent of the population.49 It is believed that bicuspid aortic valves create abnormally turbulent flow during systole, which in turn causes recession of the leaflet intimal lining and predisposes the valve to calcification and development of stenosis. Irrespective of the cause, failure of the aortic valve to open completely subjects the left ventricle to a persistent and abnormally high pressure load that results in the manifestation of two distinct processes.51–53 The first process is adaptive and is characterized by left ventricular (LV) hypertrophy aimed at off-setting the inciting pressure overload while the second process is maladaptive and is associated with continued myocyte hypertrophy in combination with progressive myocardial fibrosis, a process known to reduce ventricular compliance and to contribute to impairments in diastolic filling.52,54–59 As LV wall thickness increases, further reductions in diastolic compliance are observed, the efficacy of diastolic coronary blood flow is impaired, and coronary flow reserve is reduced in the setting of increased oxygen requirements by the larger cardiomyocyte mass. As a result of these responses, patients may actually suffer from heart failure symptoms in the absence of overt LV systolic dysfunction. Without appropriate and timely surgical intervention, chronic LV pressure overload secondary to AS, with the attendant signs and symptoms of diastolic dysfunction, can progress to frank systolic heart dysfunction, a particularly ominous condition. In addition, severe ventricular hypertrophy may create intracavitary gradients proximal to the valve, which further compound ventricular unloading and that may not be correctable with AVR.
The effects of aortic valve stenosis on myocardial structure and function, prior to, and following AVR, have been extensively chronicled.55–57 Krayenbeuhl and colleagues examined patients with AS prior to AVR and established that patients with AS exhibited significant increases in myocyte diameter and interstitial fibrosis compared to control patients while Villari and associates reported that LV relaxation was significantly prolonged prior to surgical correction.56,57 These investigations linked changes within the cellular and extracellular compartments with the clinical syndrome of pressure overload induced diastolic dysfunction. Moreover, they also demonstrated that corrective surgery abolished many of these preoperative characteristics though to different degrees and over variable time courses.55 Importantly, these investigations demonstrated that pressure overload induced abnormalities within the myocardium persist years beyond surgical correction.
Isolated AI usually resolves as a consequence of structural abnormalities of the aortic valve, causing leaflet elongation and prolapse as seen with myxomatous degeneration or in various connective tissue disorders such as Marfan syndrome. Other causes include infective endocarditis, traumatic disruption of a valve leaflet from severe acceleration/deceleration injuries, rheumatoid arthritis, Reiter’s syndrome, ankylosing spondylitis, psoriasis, and Takayatsu’s disease. Patients with calcified degenerative aortic valves may undergo leaflet perforation or tearing, especially in the belly of the leaflet, which results in combined stenosis/regurgitation.
Acute severe incompetence of the aortic valve causes a large volume of diastolic flow reversal into the left ventricle. The ventricle does not have time to adapt to this sudden event and a rapid rise in diastolic pressure occurs, causing the pulse pressure to decrease forcing early mitral valve closure to prevent back flow into the left atrium. There is a concomitant increase in heart rate and a decrease in cardiac output. The result is a rapid onset of congestive heart failure.
Chronic, progressive valve incompetence allows time for the accommodation of a smaller gradation in flow reversal. Thus, gradual ventricular dilatation occurs, with preservation of diastolic compliance such that end-diastolic pressure is not elevated in well-compensated AI. The enlarging ventricular chamber causes an increase in wall stress (as an extension of Laplace law), which will result in some ventricular hypertrophy, but overall the chamber diameter to wall thickness ratio continues to increase. The preceding events, in conjunction with the decrease in diastolic pressure, will reduce diastolic coronary blood flow. With continued dilatation, fibrosis occurs, which increases ventricular stiffness further and impairs diastolic relaxation. Eventually, the preload reserve of the ventricle is reached and further dilatation and fibrosis result in a decline in systolic function, which may not be recoverable with AVR.
Patients with AS typically present to physicians in their 60s and 70s. Patients complain of combinations of symptoms composing the classic triad of chest pain, syncope, and congestive heart failure. Chest pains in the form of classic angina pectoris are the initial symptom in 50 to 70 percent of patients. Syncope is the presenting symptom in 15 to 30 percent of patients. The life expectancy of untreated AS in patients presenting with angina is 50 percent at 5 years, 50 percent at 3 years with syncope, and 50 percent at 2 years with congestive failure symptoms such as exertional dyspnea, peripheral edema, orthopnea, and paroxysmal nocturnal dyspnea.60 A small proportion of patients with AS present with sudden death, most likely due to life-threatening arrhythmias resulting from severe LV hypertrophy. However, this seldom occurs in patients who have not had antecedent symptoms. In patients who have congenital conditions predisposing to AS, such as congenital bicuspid aortic valves, presentation may occur 10 to 15 years earlier.
AI has a more insidious onset and is usually characterized by the development of slow congestive heart failure and fatigue symptoms.61 Angina pectoris and syncope is rare. The patients may be managed on medical therapy for protracted periods of time with an acceptable quality of life. It is possible (but becoming less common) for patients with AI to be managed nonoperatively to the point where the myocardium is so decompensated that surgical treatment becomes untenable.
Patients with AS present with a typical murmur, which consists of a blowing crescendo–decrescendo type pattern best heard in the left sternal border with radiation to both carotid arteries. The heart is hypertrophied but not enlarged, so typically patients do not have palpable enlarged hearts with this condition. Palpation of the pulse reveals low volume and a delayed upstroke (pulsus parvus et tardus), owing to the gradual severity of the stenosis.
With AI, gradual LV dilatation is observed. On cardiac auscultation, an early diastolic murmur radiates to the point of maximal intensity (apex), and may be associated with a middiastolic murmur caused by fluttering of the anterior mitral valve leaflet (Austin–Flint murmur). In addition, a number of interesting physical exam signs have been noted in these patients, including “water-hammer” (Corrigan) pulses with wide pulse pressures and low diastolic pressure, DeMusset’s sign (bobbing of the head), Muller’s sign (wobbling of the uvula), pistol shots, Durozier’s sign (murmur heard over the femoral arteries), and Quinke’s sign (cyclical blanching of the nail beds).
An extremely important aspect of the physical examination in patients being considered for aortic valve surgery is the examination of the mouth and teeth. Dental disease is a major cause of prosthetic valve endocarditis (PVE) and no AVR, unless under extremely urgent circumstances, should be performed without having appropriate dental clearance when deemed necessary.
AS and AI are not associated with any specific serum laboratory abnormalities.
The chest radiograph shows calcification of the aortic valve, and a subtle convexity of the LV silhouette due to LV hypertrophy. The electrocardiogram shows LV hypertrophy, and left or right bundle branch block if the calcific process causing stenosis involves the conduction system significantly. Transthoracic echocardiography shows the calcified valve with poorly mobile leaflets in the parasternal long-axis view. A short-axis view of the valve may show whether the valve is bi- or tricuspid. Continuous-wave Doppler is used to calculate valve gradients and valve area. The peak valve gradient is calculated as a function of the highest measured flow velocity (defined as peak gradient = 4V2, where V = velocity), which is often demonstrated in the four-chamber view. The mean gradient is calculated as a function of the area of the continuous wave flow velocity curve. Valve area is calculated by the continuity equation and utilizes both continuous wave- and pulse wave Doppler. The calculation entails multiplying the LV outflow tract (LVOT) area by the LVOT flow velocity as measured by pulse-wave Doppler and then dividing the derived value by the continuous wave velocity. The accuracy of echocardiographic measurements is confounded by the presence of extensive calcification, basal septal hypertrophy, and probe orientation. In addition to the above, severe LV hypertrophy may manifest with subvalvular, intracavitary gradients. If present, this may present a significant challenge to the surgeon at operation.
At catheterization, aortic valve area is estimated at cardiac catheterization using the Gorlin formula, which, in the simplified version, is defined as valve area = cardiac output/gradient−2.62,63 A caveat to the Gorlin equation is that it is very sensitive to flow. Therefore, patients who have reduced ejection fraction may have a falsely reduced valve area, as calculated by the Gorlin equation. In these cases, correlation to the echocardiographic calculation is helpful.
The degree of AI can be estimated by echocardiography, where the rapidity of equilibration of aortic and LV diastolic pressure determines the degree of insufficiency. Accordingly, a diastolic pressure half-time of <300 ms, a short mitral deceleration time (<150 ms), and premature closure of the mitral valve all indicate severe AI. LV cavity dilatation is also assessed.
At catheterization, the degree of AI can be estimated by the degree of leakage of contrast into the left ventricle during diastole. However, this estimation is relied upon less frequently in lieu of the echocardiographic methods described above.
Patients with AS and AI should be initially evaluated with a transthoracic echo. If transthoracic echocardiography confirms the suspected diagnosis and the patient’s symptoms warrant operation, it is logical to proceed with coronary catheterization to exclude the presence of coronary artery disease (CAD). In females less than 40 years of age and males less than 35 years of age with no cardiac risk factors, coronary catheterization can be omitted relatively safely.
There is no acceptable medical therapy for symptomatic AS. The use of antihypertensive medications and angiotensin converting enzymes inhibitors must be carried out with caution because of the risk of profound hypoperfusion. Use of beta-blockade may slow the heart rate and provide for a longer systolic interval potentially resulting in higher stroke volume in patients with stenotic valves. Digitalis glycosides may provide a mild positive inotropic effect, which may be of benefit. Percutaneous valvotomy64 will reduce a tight stenosis to moderate stenosis with a final valve area between 0.7 and 1.1 cm2, still inferior to the valve area obtained with a prosthetic valve replacement, which usually provides a valve area over 1.5 cm2. The hospital mortality associated with this procedure varies from 3.5 to 13.5 percent, and within 24 h 20 to 25 percent of the patients have at least one serious complication, in particular vascular complications at the puncture site. Despite a relatively modest improvement in valve function, the quality of life benefit rarely lasts beyond a year. This technique is useful for improving the clinical status of patients with severe AS as a preamble to conventional valve replacement.65 Without valve replacement, however, the prognosis is poor. Currently, it is generally felt that valvotomy alone does not change the natural course of the disease. The poor midterm results are mainly due to the clinical status of the patients and to the moderate and transient improvement in valve function obtained by valvotomy.66 More recently, and as discussed below, percutaneous valve deployment has been successfully employed in patients deemed too high-risk for surgery.67 These valves consist of pericardial valve substitutes that are mounted on expandable stents. Results of these procedures are encouraging, but significant long-term results are not available for this technology.
The therapy for aortic valve regurgitation is primarily afterload reduction with vasodilating agents to discourage regurgitation and prevent pathologic remodeling of the myocardium.68
All patients with severe aortic valve stenosis and symptoms should be considered for traditional valve replacement. These patients tend to have aortic valve areas of less than 1 cm2 associated with a mean gradient exceeding 40 mm Hg.68 Asymptomatic patients with AS meeting these criteria should also be considered for valve replacement secondary to the small but real incidence of sudden death associated with the natural history of this lesion. The benefits of early surgical intervention for asymptomatic patients with severe AS are clearly identifiable in a clinical study recently reported by Kang and associates.69 These investigators identified patients with severe asymptomatic AS and compared the long-term outcomes of those treated with conventional therapy versus those treated with early elective valve replacement. Importantly, the latter group realized a significantly higher estimated actuarial 6-year cardiac mortality compared to those patients treated with conventional therapy.69 Thus, early surgical intervention for asymptomatic patients with severe AS and relatively low operative risk may be a worthwhile treatment strategy.
Severe AI associated with gradual ventricular dilatation and at least New York Heart Association Class II symptoms of fatigue and congestive heart failure are deemed appropriate indications for surgery. In addition, symptomatic, moderate AI with mild-to-moderate (EF >25 percent) LV dysfunction should be referred for AVR. A symptomatic patients should be considered for surgery if there is LV systolic dysfunction or severe LV dilatation (end-diastolic dimension >75 mm or end-systolic dimension >55 mm).68,70–73
The patient is placed in the supine position. Monitoring lines such as a radial arterial catheter and pulmonary arterial catheter can be placed in the holding area under sedation or after the patient is brought into the operating room. Despite excellent advances in cardiothoracic anesthesia, the occasional patient with severe AS will suffer cardiac arrest during anesthetic induction. It is therefore advisable, in cases of severe AS, for the surgeon to be immediately available from the time the patient is brought into the operating room. The patient’s chest, abdomen, perineum, and lower extremities are prepped and draped in sterile fashion. Median sternotomy is the classic mode of access to the heart, but minimally invasive approaches such as upper hemisternotomy74 and right parasternotomy75 have been described. Tacking only the right side of the incised pericardium to the retractor or skin allows the ventricular mass to rotate posteriorly and improve access to the aortic root. The patient is systemically heparinized to reach a blood activated clotting time (ACT) of 480 s. Cardiopulmonary bypass is established using dual-stage or bicaval cannulation with a LV vent placed across the mitral valve via the right superior pulmonary vein or through a stab incision in the LV apex. Mild-to-moderate systemic cooling (32°C) is used. If the valve is not regurgitant, cold blood cardioplegia should be delivered antegrade via the aortic root for more uniform myocardial distribution, then intermittently via a retrograde coronary sinus catheter for the duration of the cross-clamp period. If the retrograde catheter cannot be inserted blindly, options include bicaval cannulation and insertion of the retrograde coronary sinus catheter across the visualized coronary sinus orifice, or delivery of antegrade cardioplegia via perfusion catheters positioned or sewn into the coronary ostia. Numerous approaches to aortotomy have been described, the most common being the oblique aortotomy and near-transection aortotomy (Fig. 32-1). The oblique aortotomy begins in the anterior midline of the ascending aorta or just along the left anterior side and extends to the right and inferoposterior into the midportion of the noncoronary sinus. The near-transecting aortotomy should be made 1.0 to 1.5 cm above the origin of the right coronary artery for the best visualization. Therefore, added dissection is required on the anterior surface of the aorta to identify the right coronary artery takeoff, which can occasionally result in damage to the right coronary artery necessitating either repair or vein graft bypass of the right coronary artery. The near-transecting aortotomy is relatively easy to close but troublesome bleeding may occur posteriorly, which can be difficult to manage. In addition, if a small aortic root is encountered such that annulus enlargement is necessary, a right-angle incision must be made in the aorta and extended across the annulus, which can be very challenging to close later. Finally, since the sino-tubular junction is 10 to 15 percent smaller in diameter than the annulus, occasionally it will be very difficult to pass an appropriately sized aortic valve across the aortotomy to the annulus, especially if the aorta has lost some of its elastic properties due to calcification. The oblique aortotomy eliminates many of these concerns and is recommended by the authors. The aortic valve is inspected and the locations of the coronary ostia are identified. The valve is then carefully excised, taking care not to button-hole the aorta or damage the aorto-mitral continuity by incising too deeply. Careful debridement of all calcific matter is then undertaken where necessary followed by irrigation with cold saline to remove particulate matter (Fig. 32-2). The choice of valve and techniques of implantation are discussed below. Systemic rewarming is begun at an appropriate point in the implantation procedure so as to not impose extra time waiting for the patient to reach normothermia prior to separation from cardiopulmonary bypass. Following valve implantation, a careful inspection is performed to confirm correct seating of the valve and ensure that both coronary artery ostia are unobstructed. Also, a final check for the absence of residual particulate matter should be undertaken. The aortotomy is closed with running polypropylene suture and a de-airing vent is placed in the most anterior portion of the ascending aorta. The ventricular vent is removed. A warm cardioplegic infusion is given and the cross-clamp is removed with the patient placed in the Tendelenburg position. Ventricular and atrial pacing wires are placed and mechanical ventilation is resumed. De-airing maneuvers such as lung hyperinflation, side-to-side shaking of the chest, and manual agitation of the heart are performed with the heart partially ejecting. Transesophageal echocardiography is very helpful in assessment of de-airing, and also in the interrogation of the valve for correct leaflet function and absence of perivalvular leaks. The patient is weaned from bypass at a systemic temperature of 36.5°C with good ventilation parameters. Protamine is given slowly by the surgeon directly into the ascending aorta, and the patient is decannulated. Meticulous hemostasis is obtained, a midline mediastinal drain (36F thoracostomy tube) is placed, and the patient is closed in the standard fashion.
Subcoronary homograft aortic valves are technically more difficult to implant than stented and stentless bioprostheses and should be considered for younger patients in centers that have extensive experience with homograft valve replacements.
Prosthetic valves have the advantage of relative ease of implantation. Bioprosthetic valves have no requirement for anticoagulation postoperatively but there are two drawbacks associated with these valves. The first is the introduction of a residual transvalvular gradient (especially with smaller valve sizes), which may impede the rapidity and extent of LV mass regression after valve replacement. The second drawback, the true “Achilles’ heel” of tissue bioprostheses, is structural valve deterioration requiring reoperation for valve re-replacement. The latest-generation tissue bioprostheses such as the Carpentier–Edwards bovine pericardial76–78 and Hancock II31,79 porcine valves are comparable in results and have 80 to 93 percent freedom from structural valve failure at 10 to 14 years. These failure rates are accelerated in younger patients and in patients with renal failure. This type of valve is clearly most appropriate for older patients (i.e., greater than 70 years of age). However, concomitant cardiac disease (e.g., CAD) lowers the age at which one tissue valve will suffice for the patients’ lifetime by 5 to 10 years.80 Also, concomitant use of oral lipid lowering agents appear to have an important protective effect, with reduction of valve deterioration.81,82 Having stated the above, the risk of operative mortality with isolated aortic valve reoperation is only 5 to 10 percent,83–85 making tissue valve replacement a reasonable option even in younger patients not interested in taking oral anticoagulants and also in centers that do not perform a high volume of more complex aortic valve and root procedures.
Mechanical valves leave significant residual transvalvular gradients in smaller sizes as with stented bioprostheses, and all require oral anticoagulation. Use of oral warfarin is associated with a significant incidence of bleeding and thromboembolic complications.86,87 A recent long-term retrospective review performed by Toole and colleagues reported that freedom from thromboembolism and bleeding at 25 years was 69 ± 5 percent and 67 ± 3 percent, respectively, in patients that underwent AVR with a St. Jude Medical mechanical prosthesis at a single-center institution.86 Thus, a younger individual requiring AVR must make a choice between accepting the risk of an aortic valve reoperation with bioprosthetic valves versus the risk of bleeding and thromboembolic complications related to long-term oral use of warfarin.
Following excision of the native aortic valve, the annulus is measured using the sizers provided by the company for the valve type selected. Valve sutures are placed in horizontal mattress fashion across the remaining aortic annulus and sequentially placed across the sewing ring of the prosthesis. The prosthetic valve is then carefully seated so as to avoid obstructing the coronary artery ostia, followed by tying and cutting of all of the sutures and closure of the aorta. Certain valves are designed to be implanted in an intraannular position (such as certain St. Jude models), necessitating placement of everting (aorta to ventricle) sutures, and others are designed for supraannular seating (such as the Carpentier–Edwards pericardial prostheses), requiring placement of noneverting (ventricle to aorta) sutures. Pledgetted or nonpledgetted sutures are used; there are no conclusive data supporting superiority of one over the other. In addition, some surgeons implant the prosthesis with a continuous running suture (Fig. 32-3). While it is intuitively obvious that the largest valve size possible should be implanted, patients with severe LV hypertrophy resulting in intracavitary (i.e., subannular) gradients may actually be reliant on the stenotic native valve to impose sufficient afterload to prevent systolic ventricular collapse before ejection of an adequate stroke volume. In this uncommon situation, it may be appropriate to implant a smaller valve to impose a higher residual transvalvular gradient to encourage proper emptying of the ventricle. Alternatively, an interventricular septal myectomy/myotomy may help eliminate the intracavitary gradients in these unusual situations.88
Figure 32-3
Annular suturing techniques. A. Interrupted sutures. B. Noneverting mattressed pledgets. C. Everted mattress pledgets with replacement of ascending aorta and aortic valve. D. Continuous running suture. (Reproduced with permission from David TE. Mechanical and bioprosthetic aortic valve replacement. In: Edmunds LH (ed). Cardiac Surgery in the Adult. New York: McGraw-Hill, 1997:859–910.)

The results with stented tissue prostheses continue to improve with each successive iteration. The latest-generation tissue bioprostheses such as the Carpentier–Edwards bovine pericardial76–78 and Hancock II31,79 porcine valves are comparable in results and have 80 to 93 percent freedom from structural valve failure at 10 to 14 years.
Important clinical results pertaining to the use of mechanical valves in the aortic position primarily focus upon risks associated with bleeding and thromboembolic events. The longest data are available for the St. Jude mechanical prosthesis, where actuarial freedom from reoperation, thromboembolism, and bleeding following AVR at 25 years is 90 ± 2 percent, 69 ± 5 percent, and 67 ± 3 percent, respectively.86
This valve is usually implanted via a transecting aortotomy approximately 1.0 to 1.5 cm distal to the origin of the right coronary ostium. After the native valve is excised, the sewing ring is attached in planar fashion at the aortic annular level with simple interrupted sutures. Following this (since this valve has no supporting stent), the valve is fixed to the patient’s own aorta by sewing the rim of porcine aorta to the patient’s aorta. The valve must therefore be carefully seated with the first set of interrupted sutures so that the second suture line does not obstruct the coronary ostia with proper orientation of the valve leaflets. This valve is therefore more challenging to implant than the stented prostheses, but offers the advantage of lower valve gradients, especially with smaller valve sizes.89 The most recent data show freedom from hemodynamically significant valve insufficiency to be 82.5 percent at 9 years with 90.1 percent freedom from reoperation.90 Anticoagulation is not required with this valve.
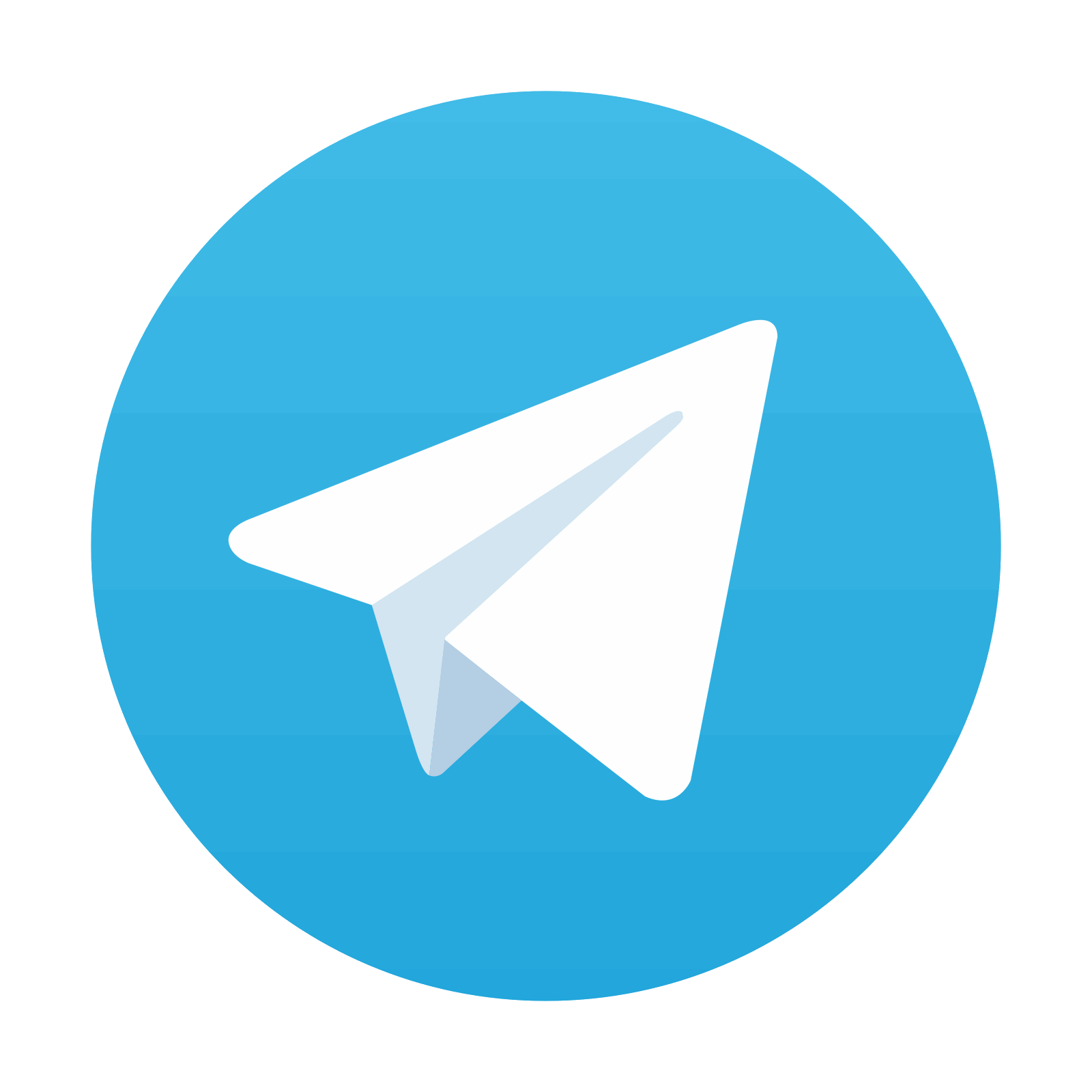
Stay updated, free articles. Join our Telegram channel

Full access? Get Clinical Tree
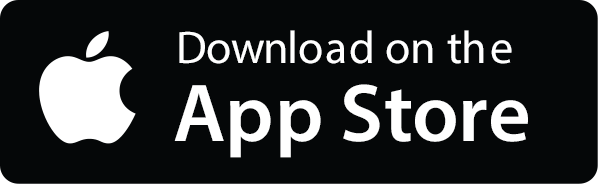
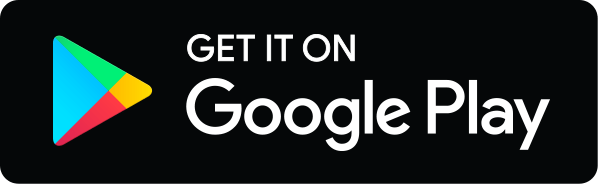