Light bending

Fiberoptic imaging
The performance of flexible ureteroscopy was first reported by Marshall with the use of their 9 French endoscope first during an open ureterostomy and subsequently during a transurethral procedure by his colleagues [7]. In 1968, Takagi and Asi reported on their work in developing flexible fiberoptic access to the upper tract of the urinary system [8]. They reported their successful attempts at visualizing the renal collecting system including pelvis and papillae utilizing an 8 French fiberoptic endoscope and evaluated its performance in both cadavers and patients. The Takagi group was the first to present their findings using still photographs of the renal papillae with their newly developed pyelo-ureteroscope [8]. Takayasu subsequently was the first to show video evidence of renal pelvis visualization with the ureteroscope in 1970 [9].
This group did much of the earliest work of flexible ureteroscope development , devising strategies for addressing various limitations. They also recognized difficulty in inserting the ureteroscope from the bladder into the ureter and initially addressed this by employing the cystoscope sheath before later developing a flexible polytetrafluoroethylene introducer sheath [10]. This difficulty was largely due to the struggle to successfully manipulate the endoscope tip. Passing the flexible ureteroscope through a catheter served to provide axial rigidity and transmitted torque along the length of the endoscope. This technique enabled the ability to maneuver the tip in the chosen direction. This experience led to the realization that a flexible tip was required for a successful endoscope.
In another breakthrough, Takayasu et al. realized that the lack of irrigation was problematic, and their first attempt at irrigation utilized the 12 Fr sheath as a means of ureteral luminal distension and better visualization. Continued innovation led to their group being the first to introduce a channel for irrigation and working instruments [9].

Rod-lens imaging
Over the next two decades, advances in rigid ureteroscope technology allowing for the fragmentation of ureteral and even renal pelvis calculi in some cases emphasized the need for ureteroscopes that could navigate the renal collecting system [12]. Changes to the original flexible ureteroscope began in the 1980s first with a passively deflectable instrument which was only able to access limited regions of the intrarenal collecting system. In 1983, Bagley and colleagues reported their experience with a new pyeloscope designed with a flexible tip that was able to be deflected 160° in one direction and 90° in the opposite direction all in the same plane [13]. This ureteroscope was also of a small enough diameter that it was able to be placed through the sheath of a rigid telescope on removing the actual telescope. This strategy of fitting the flexible ureteroscope through the rigid sheath mitigated two problems previously encountered with flexible ureteroscopy: difficulty in maneuvering the flexible ureteroscope in the renal pelvis and obtaining sufficient irrigation for visualization. The Lyon group later attempted to address the problem of ureteral access by using both a rigid and a flexible endoscope. This practice of using complimentary ureteroscopes, a semirigid ureteroscope for access to the distal ureter, and more proximal endoscopy with an actively deflectable flexible scope [13] has proven useful.
Urologists quickly recognized the need to visualize the entire collecting system which would require a steerable scope with a small deflectable tip [14]. Bagley and Rittenberg performed investigations to determine the tip deflection required for total visualization of the renal collecting system [15]. On evaluating patient radiographs, they found that the average angle between the major axis and the lower pole infundibulum was 140° and a maximum of 175°. Hence, they proposed a ureteroscope that would deflect 175°. However, on clinical use, the endoscope manufactured to these parameters often could not access the lower pole since deflection was frequently limited by instruments in the working channel and/or by variations of the collecting system itself. As such, active deflection angles continued to increase until they reached 270°, now considered the industry standard [16].
Also by the late 1980s, miniaturization and tighter packing of fiberoptic fibers produced smaller bundles, resulting in smaller-diameter flexible ureteroscopes. Eventually, several different flexible ureteroscope designs offered in sizes ranging from 8.1 to 10.8 Fr were introduced, all of which could be inserted over a wire and used to provide panoramic visualization of the intrarenal architecture without the need for a stabilizing sheath [17].
While access to portions of the lower pole could still be challenging, visualization was often able to be achieved using active (primary) and passive (secondary) deflection of the ureteroscope tip. Primary deflection up and down is controlled by the lever, while secondary deflection is a further degree of deflection that can be accomplished with an already deflected ureteroscope tip. This was achieved by introducing a more bendable segment approximately 6 cm proximal to the tip which allowed for passive endoscope buckling when the ureteroscope was maximally deflected. Secondary deflection proved particularly helpful in not only gaining access to the lower pole calyces but in allowing for the treatment or retrieval of calculi located there [18]. It has since become a standard component of flexible ureteroscope design.
Innovations in ureteroscope technology were inextricably connected to the development of working instruments. In fact, one might argue that the crucial phenomenon that made the use of ureteroscopes feasible was the development of smaller, functional instruments. In many cases the introduction of new or improved working instruments spurred the development of endoscopes to more appropriately take advantage of the advances allowed by the new or updated ancillary equipment. Many of the improvements in ureteroscope design paralleled advances in intracorporeal lithotripsy . In particular, the potential opportunity presented by the development of ever smaller electrohydraulic lithotripsy probes of 2.5, 1.9, and 1.7 French [19] encouraged the design of increasingly smaller ureteroscopes.
The introduction of the 3.6 French endoscope working channel facilitated placement of an ancillary instrument while still allowing the passage of irrigant. This design allowed the use of a wide assortment of working instruments, including guide wires, baskets and other stone retrieval implements , laser fibers , and electrohydraulic lithotripter probes . Most currently available flexible ureteroscopes continue to have a single 3.6 Fr working channel. One notable exception is the Wolf Cobra™ dual-channel ureteroscope with two 3.3 Fr channels [20]. This allows for simultaneous ancillary instrument use or for increased irrigation flow rate. However, the compromise for having two channels is a 9 Fr sheath diameter.
A successful strategy for stone treatment is the use of a small diameter ureteroscope along with an effective small diameter lithotrite. This has largely been achieved with the combination of flexible ureteroscopes with laser fibers. The first documented use of lasers was in 1966 as a result of work by Bush and coworkers who used a bovine renal model to evaluate the effect of a focused argon laser beam on renal calculi [21]. However, this and several subsequent lasers were inadequate or inappropriate for kidney calculus fragmentation [22, 23]. The development of pulsed lasers, where the energy is emitted in pulses of a given duration instead of in a continuous mode, was a major breakthrough for laser lithotripsy [24]. This type of laser allows for precision in control of the laser beam while minimizing lateral heat conduction and subsequent overheating of nearby tissue. The holmium: yttrium-aluminum-garnet (YAG) lase r, first championed for use in urology by Johnson and colleagues in the early 1990s [25, 26], has proven quite versatile and rapidly became the laser fiber of use for stone fragmentation [16]. As the laser becomes more accepted in ureteroscopy for its ability to fragment any stone with minimal tissue damage, ureteroscopes were devised to work in concert with this working instrument. Depending on fiber size, lasers can be advanced to the stone through flexible ureteroscopes with little loss of deflection capability of the scope [27].
Ureteroscope tip design has also undergone enhancements over the years. The earliest flexible ureteroscopes had flush “tin-can” tips. However, several subsequent scope iterations were introduced with beveled tips. The rationale for this engineering change was that these tips would better facilitate insertion of the scope into the ureteral orifice, thus decreasing injury. Most flexible ureteroscopes have a 0° angle of view.
There were several attempts in the 1990s to decrease ureteroscope diameter from approximately 10 Fr [28]. One of the more impactful offerings was from the Storz company in 1994; they produced an optical quality small diameter fiberoptic bundle. The miniaturization of the fiberoptic cable facilitated a substantial decrease in the size of the resulting ureteroscope’s outer diameter. Along with a corresponding increase in tip deflection, these innovations facilitated enhanced ureteral intubation and scope maneuverability throughout the upper tract. The collective work of several instrument makers that has since effected a decrease in ureteroscope diameter from 10 Fr to what is now 7.5 Fr has enabled the surgeon to gain routine endoscopic access to the intrarenal calyceal system often without a need for ureteral dilation, thus noticeably increasing treatment efficacy.
Limitations of Flexible Ureteroscopes
One of the major issues that has proven an enduring challenge for urologists is the ability to access the lower pole of the kidney using a flexible ureteroscope. While countless hours have undoubtedly gone into attempts at improving ureteroscope deflectability, natural variations in intrarenal architecture continue to make unimpeded access to all areas of the collecting system a challenge.
In their initial experiments with the flexible endoscope, Takayasu and colleagues noted significant challenges manipulating the tip. As such, they identified the need for a deflectable ureteroscope tip. The angle of scope deflection has increased over time from an initial 130° in the early 1990s [18] to a now-standard 270° two-way active deflection (upward and downward). Degree of deflection decreases, often dramatically, with the introduction of instruments via the working channel. The challenge of maintaining deflection is currently being addressed with the use of smaller instruments such as 200 μm laser fibers and 1.8 Fr baskets (as compared to 3 Fr baskets) which cause less of a change in deflection ability. Deflection occurs via movement of a lever on the ureteroscope handle. This movement can be intuitive (the tip deflects in the direction the lever is moved) or counterintuitive. While intuitive scopes are more common, there has been no consensus, and thus both options are available in current ureteroscopes. Passive or secondary deflection is available in all flexible ureteroscopes due to a flexible segment several centimeters proximal to the active deflectable segment. This allows for further maneuverability of the ureteroscope, particularly when attempting to reach difficult regions such as the lower pole of the kidney. Despite the improvements in deflection, there continues to be incomplete accessibility to the entirety of the intrarenal collecting system, often due to insufficient deflecting ability of the flexible ureteroscope with an associated working instrument.
Another limitation of current flexible ureteroscopes is the irrigant flow rate . Most current flexible ureteroscopes have a single working channel which must consequently be used for both passage of instruments and irrigation. Thus, an instrument in the channel will reduce the irrigant flow rate. The decreased flow may be offset somewhat by pressurizing the irrigant fluid and the use of smaller, less than 1.9-Fr-caliber instruments. However, maneuvering the ureter and visualizing the upper tract particularly in the event of bleeding continue to be challenging for ureteroscopists.
To this day, urologists continue to intermittently have difficulty entering the ureter directly with a flexible ureteroscope. Despite advancements by several device manufacturers, ureteroscope size continues to be a challenge; in narrow ureters advancement of the ureter may be challenging, with the urologist forced either to dilate the ureter or to place a stent and return for a subsequent procedure.
Comparison of ergonomics of robot-assisted and traditional ureteroscopy
Roboflex™ Avicenna | Traditional ureteroscopy | |
---|---|---|
Operative maneuver | ||
Insertion of ureteroscope | Fine control with joystick and numeric display of horizontal movement | Surgeon uses fingers of both hands (at glans and instrument) |
Deflection of ureteroscope | Deflection via wheel for right hand with display of grade and direction of deflection | Surgeon holds the handpiece of the scope and uses his finger to control deflection |
Rotation of ureteroscope | Fine-tunable by sophisticated left joystick | Surgeon holds the handpiece of the scope with the fingers of his other hand at the meatus |
Irrigation | Integrated irrigation pump activated by touchscreen | Assistant controls irrigation using hand, foot, or fingers, depending on mode of irrigation |
Laser lithotripsy | Integrated control of laser fiber by touchscreen; activation by foot pedal | Assistant inserts the fiber into the scope and controls the settings from the control panel; surgeon uses foot pedal to activate laser |
Use of basket/grasper | No function for basket or grasper integrated | Assistant inserts the basket or grasper and is in charge of closing the basket or grasper, while the surgeon manually manipulates the implement within the patient |
An ideal ureteroscope would have a smaller diameter while still maintaining a large working channel(s) so that instruments and irrigation can both be accommodated. Irrigation will remain necessary for the intubation and distension of the ureter as well as for access to and full inspection of the intrarenal collecting system.
Digital Ureteroscopes
Comparison of technology behind digital and fiberoptic ureteroscopes
CCD and CMOS chip (digital) | Fiberoptic bundle | |
---|---|---|
Parameters | ||
Initial reception of field of vision | “Chip on the tip”: CCD or CMOS chip positioned on distal tip of the ureteroscope with LED | Objective lens at distal end of the ureteroscope with a light diode receives the reflected light rays and focuses them onto a fiberoptic bundle |
Reception of initial image | Convert photons of light to a series of electrons | Reflected light rays focused onto a fiberoptic bundle |
Transmission of image through the scope | Wires within ureteroscope transmit the flow of electrons to the image processor | Light received from the objective lens and transmitted through the fiberoptic bundle |
Reception of final image and display | Image processor receives the electric signal and converts it into an image for real-time display | Camera located at the proximal end of the scope receives the light rays from the fiberoptic bundle and displays the image on-screen |
Comparison of digital and fiberoptic ureteroscopes
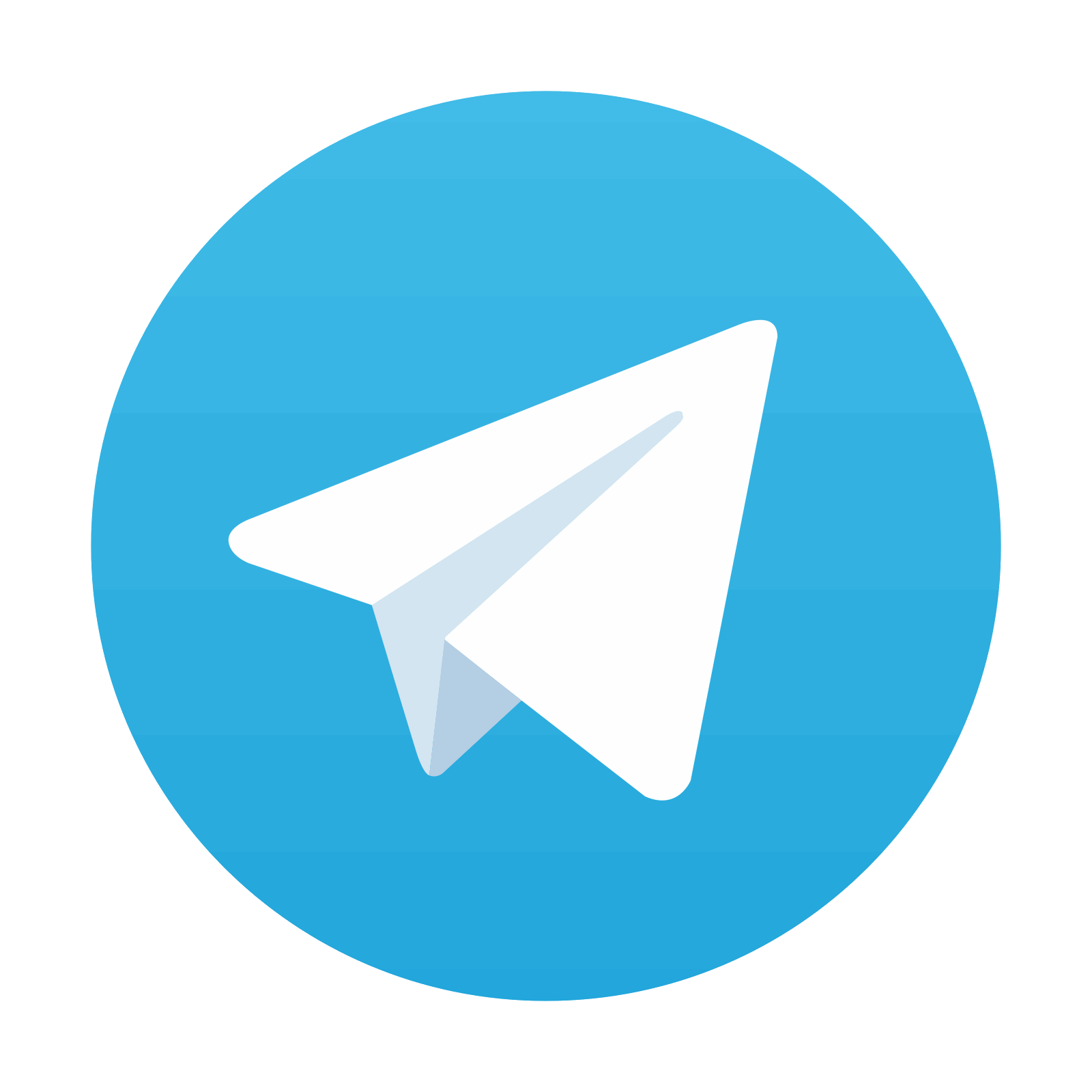
Stay updated, free articles. Join our Telegram channel

Full access? Get Clinical Tree
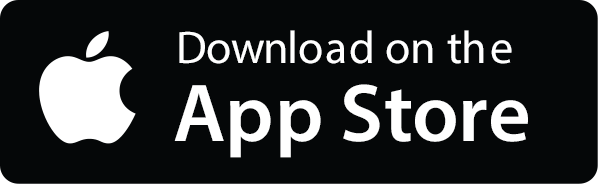
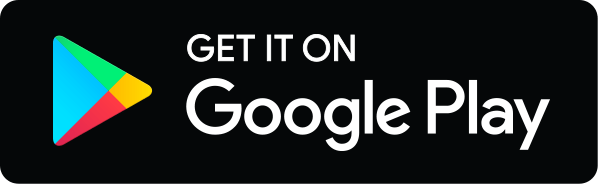