The Brown-Buerger cystoscope consists of several components which are placed and were delivered in a wooden box
The next major step was Harold Hopkins patent of his rod-lens system in 1959. The system essentially reversed the roles of the glass and the air in the conventional lensing system . Most of the space in the shaft of the telescope was taken up by glass rods. The short spaces between the rods served as the lensing. This provided for greater light transmission, better resolution, and less loss of lens alignment. Karl Storz, setting up a new manufacturing company, obtained the patent and began producing endoscopes with clearly superior visualization. Others soon followed. [9]
Fiber optics played a major role both in rigid and flexible endoscopes. In rigid instruments, fiber-optic bundles could provide the light for illumination in a small package directed exactly at the area of interest. In flexible endoscopes, they would be responsible for both illumination and visualization.
In a parallel fashion, fiber optics were first developed and then later applied to imaging. Coladon in the 1840s demonstrated the concept of internal reflection in “light guiding“ of fiber optics [10]. An important concept, the transmission of light through bent or angled glass fibers, was shown by Babinet. Still at that point, the fibers were carrying only diffuse light which could be useful for illumination but not for imaging. That step was taken in patents from Baird and Hansell in 1927 and 1930, respectively. Their fiber design provided image transmission. By 1957, Curtiss demonstrated that fibers with another layer of glass, or a cladding, offered better internal reflectivity and resultant light transmission. Also in 1957, Hirschowitz developed a flexible gastroscope using glass fibers with cladding which was clinically usable as he demonstrated on himself [11, 12].
These endoscopes found interested users throughout medical fields. Both rigid endoscopes and flexible fiber-optic imaging devices were being used anecdotally by urologists for examination of the ureter . Hugh Hampton Young performed the first ureteroscopy in a pediatric patient with posterior urethral valves and a severely dilated ureter which easily accepted a rigid pediatric cystoscope in 1912. It was reported in 1929 in a review of congenital urethral valves [13].
The next phase of ureteroscopy, still tentative, occurred in 1961. Marshall placed a 9F flexible fiber-optic scope through a ureterotomy made during an open operation to inspect for calculi . The scope had neither channel nor deflection. Two years later, Marshall reported the first transurethral flexible ureteroscopy performed by MacGovern and Walzak. A 9F flexible endoscope was passed through a 26F McCarthy sheath into a ureter to visualize a calculus [14].
Efforts to develop a functional flexible ureteroscope became serious in 1968 when Takagi et al. initiated their studies of transurethral ureteroscopy with flexible endoscopes . The hurdles quickly became evident. The instrument they used was a 70 cm 8F fiber optic passively deflectable flexible endoscope. In both cadavers and patients, they could visualize the renal pelvis and papillae but could not manipulate the tip. They also found it difficult to insert the scope from the bladder into the ureter even with cystoscope sheaths and flexible introducer sheaths, each with irrigation. In these initial studies, they recognized the need for active deflection, for an irrigation channel and the limitations of instrument size [15].
The next phase started a decade later with efforts at rigid ureteroscopy. Two urologists working independently, Goodman [16] and Lyon [17], used pediatric cystoscopes for distal ureteroscopy in women . Lyon subsequently used longer, juvenile cystoscopes in men [18]. These instruments are as large as 13F and required dilation of the intramural ureter. This step alone required considerable development of techniques and instruments. Urethral dilators were first used and were followed by unguided interchangeable bougies, wire-guided bougies, and subsequently balloons. The latter proved to be the most effective device in its final form. It required a nonelastic balloon which could achieve a high pressure in the range of 20 bar.
The next version was an even longer, 41 cm, specifically designed rigid ureteroscope. This instrument could reach the renal pelvis if it could be passed through the curvature of the ureter as it courses over the iliac vessels and lumbar muscles. The scope had a removable rod-lens telescope and a working channel [19].
To be useful, ureteroscopes had to have the capability to diagnose and treat lesions, not just to visualize them. This capability matured with the addition of working channels and suitable working instruments. Simple stone retrieval was the first therapeutic procedure. Das performed the first transurethral ureteroscopic basket retrieval of a stone in 1981 [20]. The following year Huffman used the 23 cm ureteroscope to treat 16 distal ureteral calculi . Procedures were limited to the distal ureter because of the length of the endoscope and larger stones could not be treated. The success rate was 69% [21].
The next major step in stone treatment was reported by Huffman et al. in 1983 [22]. This was the first ureteroscopic ultrasonic lithotripsy of larger stones throughout the ureter and the renal pelvis. Both of these steps in stone treatment were also dependent upon the development of new working instruments. Small baskets compatible with the working channel in the ureteroscope end and an ultrasonic lithotripter probe 2.5 mm in diameter, long enough to fit through the sheath of the long ureteroscope, were essential.

(a) The stone is visualized in the ureter with the rod lens ureteroscope. (b) The stone is trapped with a basket. (c) After applying the ultrasound probe, there is a groove in the stone. (d) The basket is held in one hand and can feel pressure of the ultrasound probe
The next logical step required a change in the endoscope and the lithotripter. A long ureteroscope was designed with a straight channel which could accept a rigid instrument and an offset eyepiece. At the same time, a smaller, 4F, ultrasonic lithotripter was developed. Therefore the probe could be passed through the ureteroscope as the stone was visualized. Although the ultrasonic lithotripter was not nearly as powerful as the other designs, it was effective in reducing the size of stones and removing fragments.

The offset ureteroscope is assembled with a handle to hold the ultrasound probe and allow it to pass directly through the straight channel
The success of rigid ureteroscopy also emphasized its limitations. Often it was not possible to access the ureter proximal to the iliac vessels or the lumbar segment. These limitations were emphasized in male patients. Flexible endoscopes could overcome these hurdles but needed the capability of irrigation and deflection to be effective. The early attempts at flexible ureteroscopy are noted above. In the 1980s, Olympus developed a deflectable flexible ureteroscope based on its pediatric bronchoscope. It was a fiber-optic instrument with a working channel. Maximal deflection was in the up direction with distal movement of the thumb lever, appropriate for a bronchoscope but possibly not a ureteroscope. Initially in the USA, there was one instrument available, used by Rob Kahn in San Francisco and D Bagley in Philadelphia, each doing 1–2 days each week with the instrument traveling by overnight carrier between the locations.
Production models of deflectable flexible ureteroscopes were introduced in the USA by ACMI. The AUR series initially included two different sized endoscopes. The larger at 9.8F had a 3.6F channel while the smaller at 8.5F had a 2.5F channel. They had 180° of deflection in one direction. This design was used to minimize the outer dimension. The one-way deflection was adequate for inspection throughout the collecting system since the endoscope could be rotated easily. The shaft was constructed of an extrusion with multiple channels for fiber optics , illuminating fibers, pull wires, and irrigation. Other flexible endoscopes at that time and even now were constructed of separate lumens for each function which were then grouped within the outer body. The size and cost savings were the basis for the extrusion design. It also eliminated the need for a separate, manually controlled vent valve. This concept arose again for the single-use endoscopes several years later.
The next in this series was the AUR7. It had two-way deflection but was remarkable for its size – 7.4F along the distal 24 cm with a 3.6F channel. The original design consisted of a shaft which tapered from the base of the handle to the tip. It proved to be resilient in clinical testing but was too difficult and expensive to manufacture. Therefore, it was changed to a step-down design at the 24 cm point. This rendered it very delicate with twisting of the shaft at that point whenever it was advanced and rotated against some resistance in the ureter. It was discontinued and subsequent models from all manufacturers were larger. The AUR7 remains the smallest fully deflectable flexible ureteroscope that became a full production model.

The tip is deflected to approximately 220°. This extent helps to correct for loss of deflection with an instrument in the channel

The VanTec single-use scope was a fiber-optic device with interchangeable rigid and flexible tips
Flexible ureteroscopes have not totally replaced rigid models. Rigid endoscopes are less expensive and more durable than flexibles. It is also easier to pass them into the distal ureter for active procedures. One of the major efforts in this development has been downsizing the total outer dimension of the endoscope. The visualization system, the rod-lens telescope, was a major space-occupying factor in the shaft, and the working channel was the second major factor.
The first step taken was to change from a rod-lens system to fiber-optic imaging which had proven its value in many endoscopes in different specialties. It was used in the ACMI RigiFlex , or HTO-5 , rigid ureteroscope to provide a channel which could accept a 5F ultrasound probe along with adequate irrigation. The eyepiece was offset to offer a straight channel through the shaft and was carried within a gooseneck form to allow movement and positioning. Overall the endoscope maintained an outer dimension of nearly 12F. It had a relatively short production duration since other smaller lithotripters became available allowing smaller endoscope design.
The concept of fiber-optic imaging in a rigid metal endoscope advanced rapidly to the next enduring plateau with the introduction of laser lithotripters. The pulsed dye laser was an effective lithotripter despite being a single-purpose laser which was relatively difficult to maintain and was expensive. The small fiber (<400 μm) could be passed through a channel <2F. Watson and Dretler developed a design with the laser manufacturer of a rigid 7F endoscope with two channels, each 2F [25]. The original concept was for continuous irrigation with fluid passing through one channel and draining out the other. That feature was not very effective. However, the design of a small rigid endoscope was a winner with variations existing to the present time.
The endoscope itself was not widely accepted because the laser manufacturer permitted sales only to laser owners and the channels could not accept any stone retrieval device then in existence.
A more successful version of the small rigid ureteroscope was the MR6 which had two channels, a 3.4F and a 2.3F [26]. These could be packaged along with the fiber-optic imaging and illumination system in an instrument with a 7F outer dimension at the tip by using a triangular cross section. 3F retrieval devices were available at that time and could be passed through the larger channel. Since stones tended to move during fragmentation with the pulsed dye laser, it was helpful to stabilize them in a basket. The fiber could be passed easily through the smaller channel. Like other rigid ureteroscopes, it was available in shorter versions of 33 cm for use in the distal ureter alone or 41 cm to reach the proximal ureter or renal pelvis. . This group of endoscopes has been termed “semirigid,” but there is no question that they are made of metal and are rigid. They can tolerate some bending but can be pushed through tissue. The successful use of these endoscopes, which have a flat tip, demonstrates that there is no need for a beak on a ureteroscope.
Another great advance has been the change in the imaging mechanism for flexible ureteroscopes . For many years, the standard was fiber optics. The introduction of small digital chips for imaging provided an image of clarity and resolution never seen before in flexible devices. Initially referred to as “chip on a stick,” digital imaging flexible ureteroscopes are available from all the major manufacturers. Both CMOS (complementary metal-oxide-semiconductor) and CCD (charge-coupled devices) chips have been used. Initially the digital instruments were approximately 2F sizes larger than fiber-optic scopes, but with a reduction in size of the chips, the tips and the shafts have reached the same size range of 8.4F. The image is rather uniformly considered superior to a fiber-optic image but may be seen to have its own deficits. There can be variations in color, highlights or burnout, and contrast. There have been concerns with scatter when there is blood in the visual field. Usually when there is failure in the system, it is total. Either there is an image or there is not. It does not have the image degradation seen with fiber optics as individual fibers break. A major barrier to the acceptance of digital imaging systems is the cost. In addition to the endoscope itself, other devices are needed to complete the imaging chain.
Driven by the high cost of flexible ureteral endoscopy, there has been increasing interest in single-use endoscopes. This is not really new but was seen in 1985 with the VanTec disposable flexible fiber-optic ureteroscope. Single-use shafts were connected to a reusable handle containing the illuminating and the optical imaging system. There was a channel with size related to the outer dimension of the shaft, adequate for irrigation and usually a working instrument. They did not have deflection, but several different versions of rigid shafts were also available. Production was discontinued when the company was acquired (Fig. 1.5).

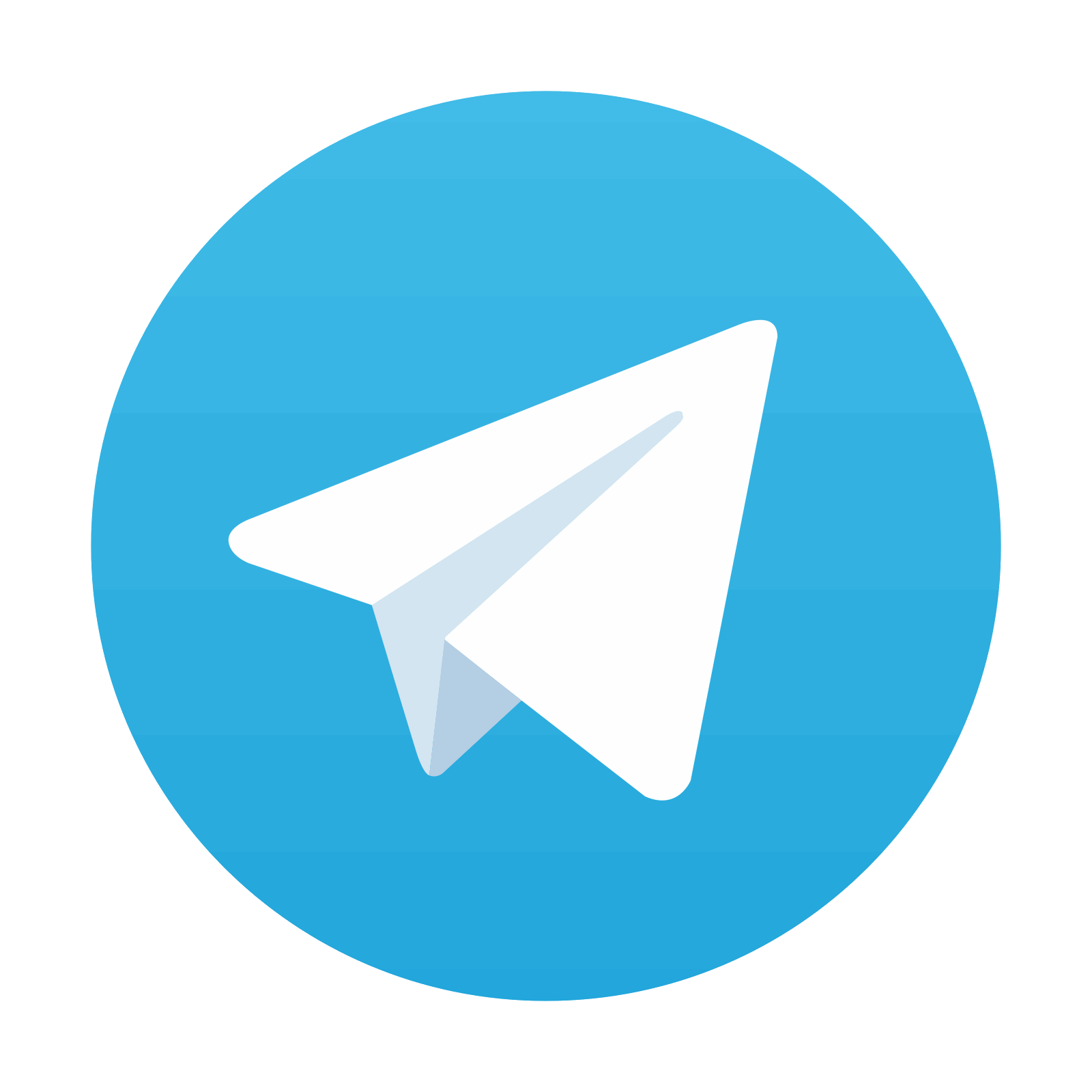
Stay updated, free articles. Join our Telegram channel

Full access? Get Clinical Tree
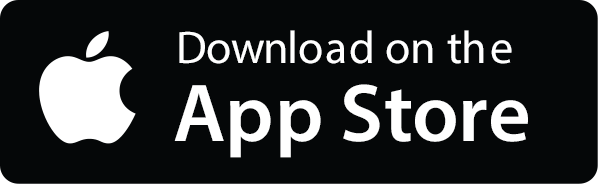
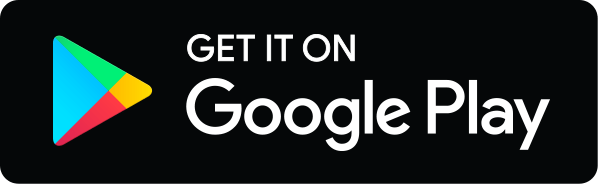