CHAPTER 69 Tumors of the Bile Ducts, Gallbladder, and Ampulla
Biliary malignancies comprise the vast majority of biliary neoplasms and are divided into the following three categories: (1) carcinomas of the intra- and extrahepatic bile ducts; (2) carcinoma of the gallbladder; and (3) carcinoma of the ampulla of Vater.1 In the United States and other Western nations, biliary malignancies are rare. In certain parts of the world, however, their prevalence rates are high, making them leading causes of cancer death. Biliary cancers are highly aggressive with dismal prognoses. Often these cancers are diagnosed at an advanced stage. In general, they are resistant to chemotherapy. Advances in the understanding of the molecular pathogenesis of these tumors has allowed the development of new experimental models and targeted therapies, and advances in diagnosis and surgical treatment have resulted in improved outcomes for subsets of patients.
CHOLANGIOCARCINOMA
Cholangiocarcinoma is an epithelial carcinoma with differentiated features of biliary epithelium that arises from the intra- and extrahepatic biliary tree.2 It is the most common bile duct tumor and second most common primary hepatic malignancy (after hepatocellular carcinoma, see Chapter 94). Since the 1970s, the incidence has increased substantially in Western societies.3
The classification of cholangiocarcinomas into intra- and extrahepatic cancers is based on differences in anatomy, etiology, pathogenesis, molecular signature, and treatment. The second-order bile ducts are the anatomic margin for the distinction between these two subsets (see Chapter 62). Extrahepatic cholangiocarcinomas account for 80% to 90% of these cancers and can be further subclassified into hilar or distal bile duct cancers. Hilar cholangiocarcinoma, also referred to as Klatskin tumors, are described clinically according to the Bismuth-Corlette classification as types I to IV (Fig. 69-1). Type I cholangiocarcinomas involve the common hepatic duct distal to the union of the right and left hepatic ducts; type II tumors involve the union of the right and left hepatic ducts; type IIIa tumors involve the union of the right and left hepatic ducts and extend up the right hepatic duct; type IIIb tumors involve the union of the right and left hepatic ducts and extend up the left hepatic duct; and type IV tumors are multifocal or involve the biliary confluence and extend up the right and left hepatic ducts. The natural course of cholangiocarcinoma is aggressive, with a median survival of less than 24 months following diagnosis.4 The only potentially curative treatment is surgical. Unfortunately, the majority of patients are diagnosed at an advanced stage that precludes curative surgery.
EPIDEMIOLOGY
Global incidence rates for cholangiocarcinoma are heterogeneous. The highest incidence is observed in Southeast Asia, with rates up to 96 per 100,000 population, and the lowest incidence is observed in Australia, with rates as low as 0.1 per 100,000 population.5 In the United States, incidence rates are 0.95 per 100,000 population for intrahepatic cholangiocarcinoma and 0.82 per 100,000 for extrahepatic cholangiocarcinoma. Ethnic differences in the incidence of cholangiocarcinoma have been observed in the United States, with the highest rates observed in patients of Hispanic descent and the lowest in African Americans. Since the 1980s, age-adjusted incidence rates for intrahepatic cholangiocarcinoma have increased, whereas those for extrahepatic cholangiocarcinoma have remained stable; the cause of the overall increase in incidence is unknown.3 Fifty-two percent to 54% of patients with cholangiocarcinoma are male. Globally, the average age at diagnosis is older than 50 years. In Western industrialized nations, most cases are diagnosed around age 65, and cholangiocarcinoma is uncommon before age 40 except in patients with primary sclerosing cholangitis (see Chapter 68).
ETIOLOGY
In the majority of cases, the etiology of cholangiocarcinoma is unknown. Several risk factors have been identified (Table 69-1). These risk factors are characterized by their association with inflammation and cholestasis. Primary sclerosing cholangitis (PSC) is one of the most common risk factors (see Chapter 68). In patients with PSC, the annual frequency rate of cholangiocarcinoma is 0.6% to 1.5%, and the overall prevalence rate of cholangiocarcinoma is 5% to 15%. In the majority of patients with PSC in whom cholangiocarcinoma develops, the diagnosis of cholangiocarcinoma is made within two-and-a-half years of the diagnosis of PSC.6 Other risk factors for cholangiocarcinoma include biliary infections with Opisthorchis viverrini and Clonorchis sinensis, which are endemic in East Asia (see Chapter 82).7 Biliary malformations such as Caroli’s disease and choledochal cysts are associated with a 10% to 15% risk for the development of cholangiocarcinoma (see Chapter 62),8 whereas hepatolithiasis (“recurrent pyogenic cholangitis”) carries a 10% risk for the development of cholangiocarcinoma (see Chapter 68).9 Recurrent bacterial cholangitis in the setting of biliary-enteric drainage procedures has also been associated with the development of cholangiocarcinoma.10 Carcinogens such as thorotrast (used as a radiologic contrast agent in the past) and dioxins have been associated with an increased risk of cholangiocarcinoma.11 Hepatitis C and cirrhosis are considered possible risk factors for cholangiocarcinoma (see Chapter 79).5
Table 69-1 Risk Factors for Cholangiocarcinoma
PATHOLOGY
Cholangiocarcinoma is a paucicellular, highly desmoplastic tumor. Macroscopically, it can be described according to its growth characteristics as mass forming, periductal-infiltrating, or intraductal-papillary. Intrahepatic cholangiocarcinomas are typically mass forming, whereas ductal carcinomas can present in any of the three growth forms. Histologically, 90% of cholangiocarcinomas are adenocarcinomas. Other histologic types include intestinal-type adenocarcinoma, clear cell adenocarcinoma, signet-ring cell carcinoma, adenosquamous carcinoma, squamous cell carcinoma, and small cell carcinoma.12 Intraductal-papillary adenocarcinomas spread superficially along the biliary mucosa without deep invasion of the fibromuscular wall layers and have a better prognosis than nonpapillary cancers.13 Metastases to regional and peripancreatic lymph nodes are frequently observed with this growth type.
PATHOGENESIS
As noted earlier, etiologic associations as well as experimental data provide evidence for inflammation and bile acids as key factors in the molecular pathogenesis of cholangiocarcinoma. Inflammation provides a microenvironment that promotes malignant transformation of bile duct–associated cells such as cholangiocytes, biliary stem cells, or epithelial cells within peribiliary glands (see Chapter 62). In subsets of cholangiocarcinoma and mixed hepatocellular-cholangiocarcinoma, hepatic stem/progenitor cells have been suggested to be the cells of origin.14 On a molecular level, increased tissue concentrations of cytokines, bile acids, and growth factors can lead to functional inactivation of mismatch DNA repair genes and tumor suppressor genes and promote the expression of proto-oncogenes. Cytokines stimulate expression of inducible nitric oxide synthase (iNOS) in epithelial cells, thereby resulting in increased intracellular levels of nitric oxide (NO) and reactive nitrogen oxide species (RNOS). NO and RNOS interact with cellular DNA and proteins and thus result in DNA mutations and strand breaks, with inactivation of DNA repair proteins.15 A variety of different oncogenic mutations have been described in cholangiocarcinoma (Table 69-2). Their frequencies differ depending on the anatomic location, stage, type, and etiology of the tumor and the ethnicity of the patient.
Table 69-2 Molecular Changes in Cholangiocarcinoma
MALIGNANT PHENOTYPE | DYSREGULATED GENE PRODUCTS AND PATHWAYS |
---|---|
Proliferation | |
Apoptosis evasion | |
Senescence evasion | |
Cell cycle dysregulation | |
Invasion/Metastases | |
Angiogenesis |
Growth factors, dysregulated signaling pathways, and tyrosine kinases promote further tumor cell proliferation, as well as inhibition of senescence and apoptosis. Key signaling pathways in cholangiocarcinoma involve interleukin (IL)-6, cyclooxygenase-2 (COX-2), epidermal growth factor receptor (EGFR), ErbB2 (also known as human epidermal growth factor receptor 2 [HER 2]), hepatocyte growth factor (HGF), and vascular endothelial cell growth factor receptor (VEGFR). The IL-6 signaling axis in particular has been shown to be critical to the pathogenesis of cholangiocarcinoma.16,17 Increased IL-6 signaling is sustained in cholangiocarcinoma cells by the following mechanisms: (1) autocrine secretion of high levels of IL-6; (2) COX-2-mediated up-regulation of the IL-6 receptor subunit gp130; and (3) inactivation of the negative feedback loop through epigenetic silencing of suppressor of cytokine signaling 3 (SOCS3). Increased IL-6 signaling constitutively activates the PI3K pathway, Janus Kinase (JAK) signal transducers and activators of transcription (STAT) pathway, and p38- and p42/44-mitogen-activated protein kinase (MAPK) pathways, which in turn mediate cell survival, cell proliferation, and evasion of cell senescence. The EGFR pathway is also constitutively activated, resulting in further activation of p38- and p42/44-MAPK.18 The EGFR homolog ErbB2 is overexpressed in many cholangiocarcinomas, resulting in downstream induction of Raf/MAPK pathways. In addition to its oncogenic effects, MAPK induces overexpression of COX-2, which is further stimulated by increased concentrations of bile acids, oxysterols, and iNOS.19,20 COX-2 also mediates cell survival and proliferation. Sustained cell proliferation is further mediated by increased HGF secretion and overexpression of its receptor, c-Met.21 The multiple pathways form a complex interacting network.
CLINICAL FEATURES AND DIAGNOSIS
The diagnosis of cholangiocarcinoma is challenging because of the paucicellular character of the malignancy and requires a multidisciplinary approach that includes clinical evaluation and laboratory, endoscopic, and radiologic studies (Fig. 69-2 and Table 69-3). Intrahepatic cholangiocarcinoma manifests predominantly with abdominal pain and systemic symptoms such as cachexia, malaise, and fatigue. Extrahepatic cholangiocarcinoma manifests in most cases with painless jaundice secondary to malignant biliary obstruction. In 10% of patients, bacterial cholangitis is the initial presenting symptom. An “atrophy-hypertrophy” complex can be documented by physical examination as palpable hypertrophy of the contralateral, unaffected lobe of the liver, with atrophy of the affected lobe as a result of vascular encasement and bile ductal obstruction.
Table 69-4 Sloan-Kettering Cancer Staging System for Cholangiocarcinoma
STAGE | CRITERIA |
---|---|
T1 | |
T2 | |
T3 |
Laboratory analysis may reveal evidence of obstructive cholestasis (e.g., elevation of serum alkaline phosphatase and bilirubin levels). Serum levels of several serum tumor markers (CA 19-9, carcinoembryonic antigen [CEA], and Ca-125) may be elevated in patients with cholangiocarcinoma; however, none of these serum markers is specific, and they each can be elevated in other gastroenterologic or gynecologic malignancies and in the setting of biliary inflammation or infection.22 The most commonly used marker is CA 19-9. In patients with PSC, the sensitivity and specificity of CA 19-9 for the diagnosis of cholangiocarcinoma is 79% and 98%, respectively, when the cutoff value is 129 U/mL. In patients without PSC, the sensitivity is 53% with a cutoff value of 100 U/mL.23,24 Importantly, patients with a negative Lewis antigen status do not express CA 19-9, and significant CA 19-9 elevations can occasionally be observed in patients with bacterial cholangitis and choledocholithiasis.
An essential diagnostic tool for cholangiocarcinoma is cholangiography, which provides both anatomic information and material for a tissue diagnosis. Several approaches can be used, including endoscopic retrograde cholangiopancreatography (ERCP), percutaneous transhepatic cholangiography (THC), and magnetic resonance cholangiography (MRCP) (Figs. 69-3 and 69-4). The choice of ERCP versus percutaneous THC depends on the location of a suspicious biliary stricture, local expertise, and accessibility of the stricture to the technique. Either technique provides information on intrabiliary tumor extension and allows cytologic sampling and therapeutic intervention for malignant biliary obstruction. Although such interventions are not possible with MRCP, this technique is noninvasive and provides additional information on the extent of extrabiliary tumor, vascular encasement, the relation of the primary tumor to surrounding structures, and intra- as well as extrahepatic metastases.2
Computed tomography (CT) is used primarily for preoperative planning; it provides information on vascular and other anatomic structures that affect surgical decision making. The sensitivity of CT for detection of nodal N2 metastases (see later), however, is only 50%, and its accuracy in the evaluation of resectability is only 60% to 75%. Magnetic resonance imaging (MRI) is currently the best imaging technique for cholangiocarcinoma; its sensitivity and tumor tissue depiction are improved by the use of ferumoxide as a contrast agent.25 Cross-sectional imaging should be performed prior to cholangiography to help guide decisions regarding biliary drainage (see later). The use of positron emission tomography (PET)/CT should be limited to cases that are indeterminate with regard to extent and other features (including malignancy) after the other studies are performed. Its sensitivity for regional lymph node metastases is only 12%. Also, the sensitivity of CT and PET for the detection of cholangiocarcinoma do not differ. PET/CT achieves sensitivity and specificity rates of 93% and 80% for the detection of intrahepatic cholangiocarcinoma but only 55% and 33% for the detection of extrahepatic cholangiocarcinoma. False-positive PET results can be observed in the setting of inflammation.26
Endoscopic ultrasound (EUS) can contribute additional information about tumor dimension and anatomic location in relation to surrounding structures. In addition, it provides information about regional lymphadenopathy and allows sampling of lymph nodes by fine-needle aspiration. Biopsy of the primary lesion via EUS is highly discouraged, however, because of the potential for tumor cell spread.2
As for most malignancies, a tissue diagnosis of cholangiocarcinoma is desirable; however, a pathologic diagnosis is challenging because of the tumor’s paucicellular character. Tissue is difficult to obtain, and cellular reactive changes resulting from inflammation often complicate the diagnosis. The most common technique for collecting tumor tissue is brush cytology during ERCP, but conventional cytology is limited because of low specificity. Fluorescence in situ hybridization (FISH) has been demonstrated to increase the sensitivity and specificity of cytology for diagnosing cholangiocarcinoma in patients with and without PSC.27 Typical findings include (1) trisomy 7; (2) tetrasomy or duplication of all labeled chromosomes; or (3) polysomy or amplification of at least three chromosomes (Fig. 69-5). Polysomy is diagnostic of cholangiocarcinoma in the appropriate clinical setting, whereas trisomy 7 is not diagnostic but increases a patient’s risk for the development of cholangiocarcinoma. Tetrasomy has to be interpreted with caution because it can be observed during the M phase of the cell cycle during mitosis (see Chapter 3).2
STAGING
Cholangiocarcinoma has been classified according to the American Joint Committee on Cancer (AJCC)/ International Union Against Cancer (UICC) TNM (tumor node metastasis) system.28 This system is based on hepatocellular carcinoma and is therefore only of limited use in cholangiocarcinoma. It is especially suboptimal for hilar cholangiocarcinoma. An improved, preoperative staging system for extrahepatic cholangiocarcinoma is needed. In oncologic surgery, negative surgical margins (R0) correlate with lack of postoperative recurrence and with prognosis. A staging system for hilar cholangiocarcinoma that incorporates resectability requires that the extent of biliary disease, vascular encasement, and hepatic lobar atrophy be assessed, in addition to the information provided by a clinical TNM system. Unilobar atrophy is clinically important, because it indicates unilateral biliary obstruction with ipsilateral vascular encasement (atrophy-hypertrophy complex). This finding does not indicate unresectability unless it occurs in combination with contralateral vascular or biliary tumor involvement.29 Such a staging system has been proposed at Memorial Sloan-Kettering Cancer Center (MSKCC) (Table 69-4). Resectability, likelihood of curative (R0) resection, metastatic spread to N2-level lymph nodes (where N1 signifies metastasis to lymph nodes within the hepatoduodenal ligament and N2 signifies metastasis to peripancreatic, periduodenal, celiac, superior mesenteric, or posterior pancreaticoduodenal lymph nodes), and survival correlate with the tumor stage in this classification system.29
Table 69-5 Exclusion Criteria for Resection of Hilar Cholangiocarcinoma
TREATMENT
The only curative treatment option for cholangiocarcinoma is surgical extirpation. Surgical outcomes have improved substantially in the 2000s because of careful patient selection with lower surgical mortality rates and higher rates of R0 (negative surgical margin) resection.30 Surgical treatment options include resection and liver transplantation (see Chapter 95).
Solitary intrahepatic cholangiocarcinoma can be resected by hepatic segmentectomy or lobectomy. Five-year survival rates after resection of intrahepatic cholangiocarcinoma range from 22% to 42%. Survival is positively correlated with early tumor stage (localized tumor), younger age, and better performance status.30 Liver transplantation is not an option for intrahepatic cholangiocarcinoma because of disappointing five-year survival rates of 0% to 18%.31
Resection is also the treatment of choice for extrahepatic cholangiocarcinoma in the absence of PSC (Table 69-5). Five-year survival rates for extrahepatic CCA after R0 resection are 11% to 41% for hilar and 27% to 37% for distal cholangiocarcinoma. Unfortunately, R0 resectability rates are less than 50%.32 Occasionally, increased resectability can be achieved by preoperative portal vein embolization, resulting in compensatory hyperplasia of the contralateral hepatic lobe. This technique allows extended partial hepatectomy because of the increased volume of the remnant liver.33 Neither adjuvant nor neoadjuvant chemotherapy or radiation therapy has been shown to be effective and is therefore not recommended in patients with cholangiocarcinoma who undergo resection.
Table 69-3 Diagnostic Criteria for Cholangiocarcinoma
* Serum CA 19-9 >100 U/mL in patients with primary sclerosing cholangitis.
In the past, liver transplantation was not an option for patients with extrahepatic cholangiocarcinoma because five-year survival rates were only 23% to 26%.34 Subsequently, new liver transplantation protocols were developed and led to 5-year survival rates of 76% in carefully selected patients with hilar cholangiocarcinoma.35 The selection criteria are strict (see Table 69-3 and Chapter 95). The treatment protocol consists of neoadjuvant external beam radiation therapy with concurrent systemic 5-fluorouracil chemotherapy, followed by brachytherapy and oral maintenance chemotherapy with capecitabine until transplantation.
At the current time, no curative medical therapies for cholangiocarcinoma are available. A variety of chemotherapeutic agents such as gemcitabine, other antimetabolites, taxanes, platinum analogs, anthracyclines, and mitomycin have been evaluated as single or combination therapies. The only chemotherapeutic drug approved by the U.S. Food and Drug Administration (FDA) for cholangiocarcinoma is gemcitabine; however, no randomized, controlled phase III trials have shown a significant survival benefit with chemotherapy in patients with cholangiocarcinoma. Available studies have been statistically flawed or underpowered or have shown poor response rates. Therefore, chemotherapy is not a treatment option for these malignancies unless used in the palliative (see below) or neoadjuvant setting. Similarly, the use of radiation therapy remains controversial.2
Palliative Treatments
Cholangiocarcinoma is associated with substantial morbidity. Commonly, patients experience cholestasis, abdominal pain, and cachexia, which limit the quality of life. Therefore, palliative treatments are essential in the management of patients with cholangiocarcinoma. Options for restoration of biliary drainage include endoscopic, percutaneous, and surgical techniques. Endoscopic and percutaneous methods are based on placement of biliary stents (see Chapter 70), whereas surgical approaches create a bypass via a choledocho- or hepaticojejunostomy. The efficacies of similar endoscopic and surgical approaches are similar, but the mortality rate, frequency of procedure-related complications, and duration of hospital stay are higher for surgical palliation.36
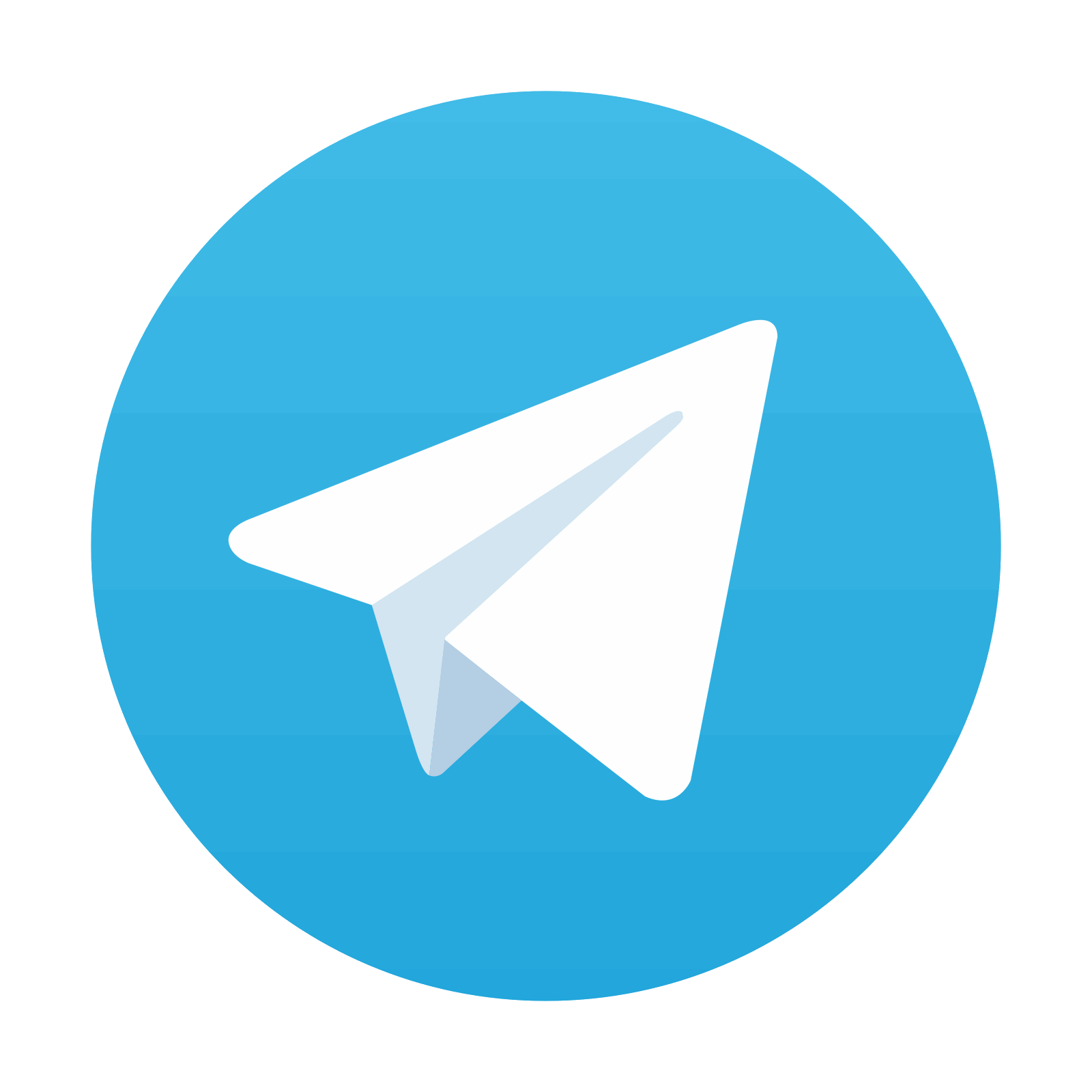
Stay updated, free articles. Join our Telegram channel

Full access? Get Clinical Tree
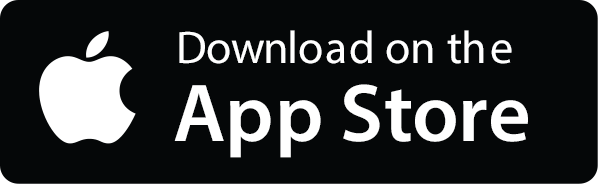
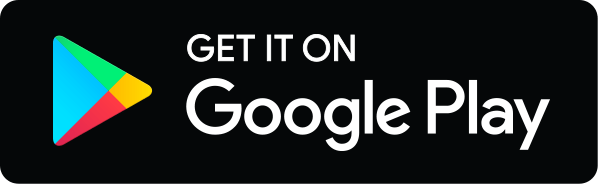