Hepatic metastases to the liver are significantly more common than primary liver malignancy and comprise approximately 90% of hepatic malignancies. Each year, approximately 140,000 new cases of colorectal cancer (CRC) are diagnosed in the United States, with hepatic metastases complicating 10% to 25% of cases.1,2 Two-thirds of these patients die from their disease as a result of liver involvement.2 In addition, numerous other malignancies metastasize to the liver. For instance, approximately 50% of patients with uveal melanoma develop liver metastases within 2 to 5 years after initial diagnosis, and 90% of metastatic uveal melanoma patients die with disease burden in the liver due to inefficacious systemic chemotherapy.3 Neuroendocrine tumors (NETs) also have propensity to metastasize to the liver as well as breast cancer, esophageal cancer, gastric cancer, gastrointestinal stromal tumors (GISTs), sarcoma, gynecologic and urologic malignancies, and melanoma.4 Therefore, because of the significant number of patients impacted by either primary or secondary liver malignancy, there is an increasing need for effective locoregional therapy. Surgical resection as a part of multimodal therapy is generally considered the gold standard.5-8 However, resection is often not a reasonable option secondary to disease burden, tumor location, insufficient estimated future liver remnant, extrahepatic disease, and medical comorbidities. In fact, surgical resection for metastatic disease to the liver is only feasible in approximately 20% of patients.9 Therefore, alternative options are necessary in the 60% to 80% of patients with metastatic disease to the liver in whom surgery is not possible.9 These nonresective techniques are the focus of this chapter.
Malignant tumors confined to the liver that cannot be addressed with curative intent are best treated with locoregional therapy. Primary liver lesions such as hepatocellular carcinoma (HCC) and intrahepatic cholangiocarcionma as well as metastatic liver lesions such as colorectal, neuroendocrine, breast, melanoma, and renal can be palliated or down-staged with arterial-directed therapy. Transarterial directed therapy includes bland embolization, chemoembolization, drug-eluting beads (DEBs), and radioembolization. Bland embolization induces tumor ischemia by arterial disruption but does not use chemotherapeutic agents; therefore, tumor necrosis is simply accomplished by restricted blood supply. Chemoembolization, DEB, and radioembolization deliver toxic agents via the arterial system which preferentially supplies the tumor, in contrast to normal hepatocytes which derive much of their blood supply from the portal venous system.10 The latter three techniques are the focus of the arterial-directed therapy section.
Transcatheter arterial chemoembolization also known as transarterial chemoembolization (TACE) was first introduced in 1980 for treatment of unresectable liver tumors, and since that time has expanded to include treatment of numerous malignant processes (HCC, neuroendocrine metastases, cholangiocarcinoma, melanoma, colorectal metastases).11 Conceptually, liver tumors preferentially utilize hepatic arterial supply, and therefore chemotherapeutic agents with subsequent embolization can selectively target malignant cells while preserving surrounding liver parenchyma. Specific delivery of chemotherapeutic agents reduces systemic toxicity as the agents remain in the tumor bed; in addition, ischemia induced by arterial embolization can increase tumor cell lethality. However, preservation of proximal hepatic arterial inflow is essential to allow repeated treatments and the optimal number of treatments is based upon tumor response and hepatic reserve. Tumor response is characterized by reductions in size or lack of enhancement on CT/MRI indicating tumor necrosis.11
After meticulous patient evaluation and characterization of tumor extent, femoral catheter insertion and subsequent angiography is utilized to delineate the vascular anatomy in the region of the tumor (Fig. 59-1A,B). The chemotherapeutic agent is then introduced into segmental or subsegmental vessels feeding the tumor(s). Variability in technique is common, including altering chemotherapeutic agents and embolic material, as well as inclusion of lipiodol, and also the technical selectivity of catheter positioning. Lipiodol is an iodinated ester from poppy seed oil that is combined with the chemotherapeutic mixture which allows emulsification of the drugs as well as directed targeting of liver tumors because the compound is selectively retained by liver tumors (Fig. 59-1C).11 The most common cytotoxic agents used include doxorubicin, gemcitabine, cisplatin, and mytomycin C (MMC). After delivery of chemotherapy agents, embolization is performed. Commonly used embolic agents include polyvinyl alcohol particles, starch microspheres, or gelfoam.11,12 Following embolization, angiography is performed, and subsequently cross-sectional imaging is obtained to confirm the interruption of blood supply.12 Patients generally require a short hospital stay post-procedure for intravenous hydration and pain control, as well as to monitor for post-embolization syndrome (discussed below). Additional TACE procedures can be performed at 4-week intervals until the specified tumor volume has been treated. Follow-up cross-sectional imaging is generally obtained at 1 month after completion of treatment to assess response; imaging is then obtained at 3-month intervals thereafter.13
Figure 59-1
Conventional transcatheter arterial chemoembolization (TACE). (A) Contrast-enhanced CT images demonstrate a 9.1 × 8.8 cm heterogeneously enhancing mass in the right lobe of the liver in a female patient with metastatic leiomyosarcoma. (B) Digital subtraction angiography (DSA) anteroposterior view of a segmental right branch off the replaced right hepatic artery showing the hypervascular lesion. (C) Spot fluoroscopic image following conventional TACE demonstrates lipiodol deposition in the treated lesion.



Early randomized studies focused on TACE for unresectable HCC. Many did not demonstrate survival benefit of hepatic arterial chemoembolization versus more conservative treatment.14-19 However, more recent studies have identified a benefit in patients with unresectable HCC confined to the liver, and therefore TACE has emerged as a safe therapeutic option.20-22 Subsequently, metastatic disease to the liver has also been evaluated. Vogl and associates identified 463 patients with unresectable colorectal liver metastases (CRLM) that demonstrated no response or disease progression on systemic chemotherapy (two or more lines of chemotherapy), or developed toxicity precluding further systemic treatment. Therefore, all of the patients in the study received no further systemic chemotherapy. Patients received TACE comprised of MMC, MMC with gemcitabine, or MMC with irinotecan followed by embolization. The mean number of sessions was 5.3 per patient, and the TACE was tolerated quite well with minimal toxicity. Partial response (PR) was demonstrated in 14.7%, stable disease in 48.2%, and disease progression in 37.1% of patients according to the Response Evaluation Criteria in Solid Tumors (RECIST). Approximately 13% of patients achieved downstaging which allowed percutaneous ablative procedures to be performed. Median overall survival (OS) duration was 38 months from diagnosis of liver metastases and 14 months from initiation of chemoembolization; these survival times did not differ among the three TACE groups.23
Albert and colleagues investigated TACE in 121 patients with metastatic CRC, most of whom had failed systemic chemotherapy for nonresectable CRLM. Chemoembolization consisted of MMC, doxorubicin, and cisplatin and was provided in 4-week intervals until the entirety of tumor volume had been treated (one cycle). Most procedure-related toxicity consisted of pain, fever, nausea, and emesis; serious complications occurred in 11% and included hepatic infarction, hematoma, pulmonary edema, myocardial infarction (MI), and venous thromboembolism (VTE). Overall, 2% had PR, 41% had disease stability, and 57% had disease progression (RECIST). Median time to progression was 5 months and median OS was 9 months after initiation of TACE. Median OS after diagnosis of liver metastases was 27 months, and the authors suggested that given the median survival was greater than historical survival of patients receiving systemic chemotherapy alone (ie, 20 months), chemoembolization provided an additive benefit to systemic chemotherapy.13
Vogl and colleagues evaluated TACE followed by MR-guided laser-induced thermotherapy (LITT) for patients with CRLM (unresectable, inoperable, contraindications for surgery, or refusal of surgery). The mean number of TACE treatments was 3.7 and patients received MMC, MMC with gemcitabine, or MMC with irinotecan. PR was seen in 31% of patients with stable disease in 69%; overall mean reduction in tumor size was 21.4%. Reduction in tumor size was sufficient to allow LITT for all of the patients. Median time to progression was 8 months and median OS was 23 months. There was no difference between the treatment groups with respect to reduction in tumor size, response rate, time to progression, and OS. In addition, the treatments were well tolerated, with no mortalities or major complications.12 The authors concluded that the OS was favorable compared to previous studies demonstrating median survival between 10 and 20 months in patients with nonresectable CRLM receiving systemic or regional chemotherapy.24,25
Use in liver metastases from noncolorectal primaries has also been demonstrated. Dong and Carr performed a retrospective study of TACE (doxorubicin or streptozotocin) in the treatment of unresectable metastatic NETs to the liver. An average of seven cycles was provided and PR was found in 62%, while 24% had minor response or disease stability. Median survival was about 3½ years, and the treatment was well tolerated.26 A recent analysis of therapeutic options for patients with gastro-enteric-pancreatic (GEP) NET identified TACE as a treatment modality for those with unresectable disease. However, the level of evidence presented was low and studies have failed to demonstrate an advantage of TACE over bland embolization.27
Taken together, these investigations demonstrate marginal benefit at best for patients with CLRM undergoing TACE versus systemic chemotherapy. In addition, there have not been any robust studies demonstrating improvement in outcome utilizing TACE for CRLM.28 For these reasons, TACE is not currently recommended for treatment of colorectal liver metastases except as part of a clinical trial.29 Coincident with this, the European Neuroendocrine Tumor Society (ENETS) consensus guidelines for patients with GEP NET liver metastases stated that although symptom improvement occurs in 73% to 100% (duration 14-22 months) and CR/PR occur in approximately 33% to 50% of patients undergoing TACE for liver metastases from GEP NET, there remains uncertainty whether TACE provides a survival advantage over systemic therapy.30
Patients with good performance status and tumor suitable for resection, ablation, or transplantation should pursue curative measures. In patients with unresectable liver malignancy (primary or metastatic) and disease primarily confined to the liver, TACE can be utilized as salvage monotherapy when patients are unresponsive to systemic chemotherapy.12,13 TACE can also be used concordantly (neoadjuvant) with other treatment modalities such as LITT, radiofrequency ablation (RFA), microwave ablation (MWA), and resection. However, currently TACE appears to be best suited for treatment in patients with HCC (intermediate stage) as opposed to patients with liver metastases.31
Congruent with the exclusion criteria for many of the above studies, contraindications to chemoembolization are absence of hepato-pedal flow and lack of compensatory collaterals, poor hepatic function (encephalopathy or ascites not controlled with diuretics), and biliary obstruction. Relative contraindications include poor hepatic synthesis (serum albumin <2 mg/dL), hepatic compromise (serum bilirubin >2 mg/dL, lactate dehydrogenase >425 U/L, aspartate aminotransferase >100 U/L), bleeding varices, ascites, thrombocytopenia, extensive tumor burden (>50% of liver), cardiac insufficiency, or renal insufficiency.10
While TACE is generally well tolerated, complications include liver abscess (1%), tumor rupture (1%), acute liver failure, gastrointestinal bleeding (1%-4%), pulmonary embolism, renal dysfunction, cardiac toxicity, bile duct injury, bleeding from femoral puncture site (1%-2%), and post-embolization syndrome. This syndrome, which occurs in 4% to 10% of patients, is characterized by right upper quadrant pain, nausea, emesis, fever, and liver enzyme elevation.10,11 Although common, the syndrome typically resolves spontaneously within 7 to 10 days.
Transcatheter delivery of agents such as doxorubicin and cisplatin has less systemic toxicity compared to standard systemic chemotherapy.32 Despite this, adverse effects such as acute liver failure, encephalopathy, ascites, upper GI bleeding, marrow suppression, alopecia, and renal failure still occur.10,11 Although TACE has demonstrated benefit for unresectable HCC, there remains a necessity for improved response rates and decreased complications which may allow expansion of indications. Localization of drugs specifically to the tumor with subsequent sustained release of the specific drug may result in decreased systemic effects and longer tumor exposure to the chemotherapeutic agent. By loading various chemotherapy agents onto polyvinyl alcohol-based hydrogel (DC-Beads, Biocompatibles, Surrey, UK) or a sodium acrylate and vinyl alcohol copolymer (HepaSphere, BioSphere Medical, Rockland, MA) and catheter delivery similar to standard TACE, drug-eluting bead transarterial chemoembolization (DEB-TACE) achieves directed delivery to the tumor microvasculature where the small compounds (100-300 μm and 300-500 μm, respectively) obstruct the vasculature.33 The lodged DEBs allow slow elution of chemotherapeutic agent in a sustained manner with prolonged exposure compared to TACE and lower systemic plasma levels of chemotherapeutic agent, resulting in reduced adverse effects.34,35 The technique is quite similar to TACE with the exception of the deployment of DEB microspheres impregnated with chemotherapeutic agent as opposed to chemotherapeutic agent with emulsificant and embolic agent.36 Additional considerations include presence of replaced or aberrant vessels, avoidance of embolization of cystic artery, and evaluation of phrenic artery if suspected to supply the target tumor.37
In the setting of CRLM, a phase III multi-institutional prospective randomized trial evaluated DEB loaded with irinotecan (DEBIRI) compared to systemic chemotherapy including folinic acid, 5-FU and irinotecan (FOLFIRI) in patients presenting with unresectable liver metastases from CRC (<50% liver parenchyma and no extrahepatic disease) who had undergone prior chemotherapy. Complete or partial response occurred in 69% of patients receiving DEBIRI compared to 20% of the systemic chemotherapy patients. At 2 years, survival was 56% in the DEBIRI group compared to 32% in the FOLFIRI group, and median OS was significantly higher in the DEBIRI group (22 months vs 15 months, p = .03). Furthermore, neutropenia greater than or equal to grade III occurred in 4% of the DEBIRI population compared to 44% of the systemic population with fewer rates of diarrhea (6% vs 18%), alopecia (5% vs 35%), asthenia (20% vs 50%), and mucositis (1% vs 20%), indicating a superior safety profile. Supplementary data revealed improved quality of life scores and significant reduction in cost in the DEB-TACE patients compared to the patients receiving FOLFIRI.32 This study emphasized the importance of consideration of arterially directed therapy in select patients with metastatic disease to the liver.
The indications for DEB-TACE fall into the same category as for standard TACE; most studies have evaluated its use in HCC.37,38 DEB-TACE may provide survival benefit compared to systemic chemotherapy for CRLM; however, further studies are necessary to clarify this potential role.39
Prajapati and colleagues sought to evaluate the safety and efficacy profile of small (100 to 300 μm) and large (300-500 μm) DEBs. DEB TACE with doxorubicin was performed in 94 patients (mean 2.8 per patient). Each group experienced similar mild complications (approximately 30%) consisting of abdominal pain (18%-26%), nausea (13%-17%), fever (3%-4%), and groin pain (3%-4%). The larger bead group had significantly more severe complications, including femoral artery pseudoaneurysm (1.7 vs 2.9%), prolonged hospitalization due to severe abdominal pain (1.7% vs 8.6%), and encephalopathy (0% vs 8.6%). Other complications included bradycardia (1.7%) and gastric ulceration/bleed (1.7%). In addition, mortality was 0% in the small bead group and 4% in the large bead group, leading the authors to conclude that 100 to 300 μm DEB may have an improved safety profile compared to larger beads.36
Yttrium-90 (90Y) radioembolization is a transarterial treatment for locoregional disease with demonstration of a clear palliative role by achieving delayed progression of hepatic lesions.40 Selective internal radiation therapy (SIRT) exploits the preferential arterial perfusion of hepatic malignant disease in order to expose liver lesions to radioactive microspheres. There are two microsphere forms that are commonly used including nonbiodegradable glass microspheres (TheraSphere™, MDS Nordion, Ottawa, Canada) of diameter 20 to 30 μm, and biodegradable resin microspheres (SIR-Spheres™, Sirtex, Lane Cove, Australia).10 As opposed to chemoembolization that combines chemotherapy and ischemia to achieve desired antitumor effect, 90Y radioembolization relies upon intravascular brachytherapy radiation effect to the targeted segment or lobe. The goal pulmonary exposure (pulmonary shunting) is less than 30 Gy while optimizing exposure to tumor.41 Prior to radioembolization of hepatic tumor, a simulation angiographic injection of 20 to 100 μm technetium-99m labeled albumin followed by single photon emission computed tomography (SPECT) imaging allows estimate of 90Y deposition (Fig. 59-2 A-D).40,41 The pretreatment simulation allows for projected tumor delivery as well as to evaluate for gastrointestinal (aberrant vessels) or pulmonary shunting (Fig. 59-2 E-G).41,42 Consequently, a tumor delivery dose can be estimated; however, tumor dose and resultant objective response is dependent upon tumor type, vascularity of the tumor (ie, intratumoral shunting), and use of previous systemic or intra-arterial agents.42 In addition, the targeted liver volume with associated feeding vessels can greatly influence the delivery dose. For instance, localized tumors confined within a specific segment can be targeted with the entire planned infusion of 90Y resulting in a “radiation segmentectomy” in which substantial doses are achieved (>1000 Gy).42 However, indications are not limited to segmental infusions, as lobar and whole-liver treatments are also routine and can be performed safely.33
Figure 59-2
90Y radioembolization in a patient with multifocal hypervascular carcinoid metastases. (A) Early arterial and (B) delayed arterial phase digital subtraction angiography (DSA) images following selective right hepatic arterial injection. Note: The gastroduodenal artery has been prophylactically embolized to avoid non-target embolization of glass microspheres to the pancreaticoduodenal arcade. (C) Early arterial and (D) delayed-phase DSA images following selective left hepatic arterial injection in the same patient. Note arterial supply to segments II-IV as well as a small caudate artery coursing inferiorly off of the left hepatic artery (arrow). (E) 3D volumetric data is obtained from CT imaging performed during mapping angiography and used to facilitate dosimetric calculations for each lobar treatment. (F,G) Here, segmentation of the right hepatic lobe is used to calculate the volume of liver parenchyma perfused by the right hepatic artery.







Radioembolization is accomplished 1 to 2 weeks following the simulation. Femoral catheter cannulation and subsequent angiography is utilized to delineate the vascular anatomy in the region of the tumor. The selective internal radiation therapy then consists of infusion of 90Y loaded onto resin or glass microspheres. 90Y is pure β-emission radiation and has a mean tissue penetration of 2.5 mm (maximum 10 mm) with a relatively short half-life of 64.1 hours.42 For this reason, isolation for radiation precaution is not required and the majority of radiation energy (97%) is emitted within the first 2 weeks. Although the absorbed dose is heterogeneous based on hemodynamics and tumor vasculature, the majority of the 90Y is absorbed into the tumor compared to the normal liver parenchyma (3:1 to 20:1 ratio). The consequence of radiation exposure to tumor cells is irreversible cell damage. Patients are either observed in the hospital setting for a period of less than 24 hours or the procedure is performed on an outpatient basis. Follow-up imaging is then obtained at specified intervals in order to determine the response to therapy by RECIST criteria, World Health Organization (WHO) criteria, or European Association for the Study of Liver (EASL) criteria (Fig. 59-3). In the setting of downstaging or identifying tumor response based on size criteria alone, imaging can inadequately predict response as a result of discordance between residual size and cell viability. For example, in a study by Kulik et al. in patients with liver tumors treated with 90Y who had tumor response allowing subsequent liver transplant, there was radiologic-pathologic discordance in five of seven patients receiving liver transplant. Imaging suggested viable tumor in each of the seven patients, while pathologic analysis demonstrated complete pathologic necrosis in five patients.42 Therefore, additional characteristics such as enhancement on CT or MRI, or PET imaging can be utilized to estimate tumor volume as defined by the modified RECIST assessment or the EASL guidelines assessing percent change in enhancing tissue.43
Figure 59-3
90Y radioembolization treatment and follow-up imaging. (A) Contrast-enhanced CT demonstrates a 2.5 × 2.7 cm homogeneously enhancing NET metastasis in segment VIII. (B) Three-month and (C) 6-month post-contrast T1WI following 90Y radioembolization confirm significant post-90Y necrosis and volume reduction.



90Y radioembolization has been evaluated in numerous studies and is currently recognized as a treatment option for unresectable Barcelona Clinic Liver Cancer (BCLC) intermediate stage HCC in the National Comprehensive Cancer Network guidelines.40,42-44 Metastatic disease to the liver has also been demonstrated to respond to 90Y therapy and is currently a category 3 recommendation for CRLM due to limited data in a highly select patient population.29
In a single-institution analysis, Cianni et al. evaluated 110 patients with liver metastases (colorectal, breast, gastric, pancreatic, esophageal, melanoma, cholangiocarcinoma, and pharyngeal) unresponsive to systemic chemotherapy and not amenable to local therapy. Approximately 60% received whole-liver SIRT (90Y), 20% sequential, and the remainder lobar. Among CRC patients, CR or PR was seen in 46%, while in breast cancer patients CR/PR was 44%. 90Y achieved clinically relevant response rates and was well tolerated, indicating a potential therapy for patients with metastatic disease to the liver.45
The same Italian group performed a study to evaluate 90Y SIRT in 52 patients with metastatic breast cancer. The motivation arose from the fact that only 10% to 20% of patients with hepatic metastases from breast cancer can undergo attempted resection and therefore additional therapies require evaluation. The selected patients were inoperable with chemotherapy-unresponsive hepatic metastases. Patients with less than 25% liver involvement had 54% PR and median survival of 14.3 months, while patients with 26% to 50% liver involvement had 60% PR and median survival of 8.2 months. Considering these patients were receiving salvage therapy, the authors suggested studies investigating earlier use of SIRT (potentially in combination with standard therapies) in metastatic disease to the liver.46
In 2012, a group of experts in the management of patients with liver metastases from NET convened to determine appropriate treatment in patients who are not candidates for surgery or RFA. They examined 18 reports on utilization of transarterial embolization (TAE) and TACE (11 publications), or radioembolization (7 publications). In the studies evaluating 90Y treatment, there were response rates ranging from 22.5% to 71.4% and median survival ranging from 22 to 70 months, highlighting the heterogeneity of the studies and populations. In a representative study, Memon et al. investigated 90Y in 40 patients with unresectable liver metastases from NET. Median liver dose was 113 Gy and lung dose 3.81 Gy. Complete or partial response was noted in 64% with stable disease in 32.5% and median OS was 34 months. Symptom control was achieved in 84%.10 These response rates and median OS were similar to the wide array observed in TAE/TACE. The panel concluded that future studies are required for direct comparison of the intra-arterial therapies, use of intra-arterial therapy versus systemic therapy, and concomitant use of intra-arterial therapy with systemic therapy in order to optimally define the role of 90Y in NET metastases the liver.47 Unfortunately, the broad heterogeneity and rarity of this tumor will make future studies difficult to interpret, since it is unlikely an RCT will be performed.
From these studies, it is apparent that 90Y treatment for liver tumors is a useful adjunct, but its specific capacity remains to be established. Therefore, due to the necessity of additional studies evaluating SIRT in metastatic disease to the liver, investigations have been established that will help clarify its role. For instance, patients with unresectable CRLM will be randomized to FOLFOX ± bevacizumab versus single-session SIRT + FOLFOX ± bevacizumab (SIRFLOX study) to determine effect on progression-free survival (PFS) and OS.48 The premise of this study (and another similar study, FOXFIRE) is the increased PFS observed in patients with CRLM that received 5-FU/LV and SIRT (18.6 months) versus 5-FU/LV (3.6 months).49 These, along with additional ongoing studies, will better delineate the optimal patient selection for 90Y-SIRT, either as monotherapy or in combination with other modalities.
In general, patients with Eastern Cooperative Oncology Group (ECOG) performance status of 0 to 2 without extrahepatic life-limiting disease, and adequate hematologic parameters with appropriate pulmonary, renal (creatinine <2 mg/dL), and liver function (bilirubin <2.0 mg/dL) are candidates for SIRT. Multiple malignant tumors (primary and secondary) of the liver have been treated with 90Y radioembolization, allowing for broad application. In addition, patients with disease not amenable to resection or ablation, and who are poor candidates for TACE due to extensive tumor burden or portal vein invasion, or patients who had disease progression following TACE can be treated with SIRT.
When considering transarterial therapy, a noteworthy characteristic of SIRT is the microembolic nature of the 90Y-microspheres. Sato et al. evaluated 30 patients undergoing SIRT for unresectable HCC or liver metastases and found that pretreatment arteriography and post-treatment arteriography (1-3 months) revealed 100% patency of first- through third-order vessels and inability of experienced radiologists to correctly determine pre- versus post-treatment imaging based on arterial flow, emphasizing the microembolic character of 90Y.50 In addition, given the microembolic nature of SIRT (as opposed to macroembolic phenomena in TACE), use in patients with portal venous thrombosis has proven safe and effective without the risk of ischemic hepatitis and hepatic decompensation seen with chemoembolization.51 Furthermore, 90Y radioembolization is equally well tolerated in young patients compared to those greater than age 70, indicating an appropriate treatment option for the elderly patient population whose frailty may limit alternative treatment methods.52
Contraindications include excessive estimated radiation doses to the lungs (>30 Gy single dose or >50 Gy multiple doses), uncorrectable flow to the gastrointestinal tract on angiography (ie, inability to occlude aberrant vessels), poor hepatic synthetic function (albumin <3 mg/dL), signs of limited hepatic reserve (bilirubin >3 mg/dL in absence of reversible cause, ascites, encephalopathy, recent variceal hemorrhage), or significant extrahepatic disease that is life-limiting.33
Radioembolization-induced liver disease (REILD) is a syndrome manifest from sinusoidal obstruction that occurs 4 to 8 weeks after treatment. Patients developed jaundice, ascites, and moderate increase in canalicular enzymes; this occurs in 8% to 15% of patients undergoing partial liver radioembolization and is mostly transient but can be severe in approximately 3.1%.53 Other adverse effects from SIRT include fatigue (50%), nausea or emesis (30%), abdominal pain (20%-30%), fever (10%-15%), pneumonitis (rare), and gastrointestinal ulcers (1%-3%)—the overwhelming majority of which are grade I or II adverse events.52
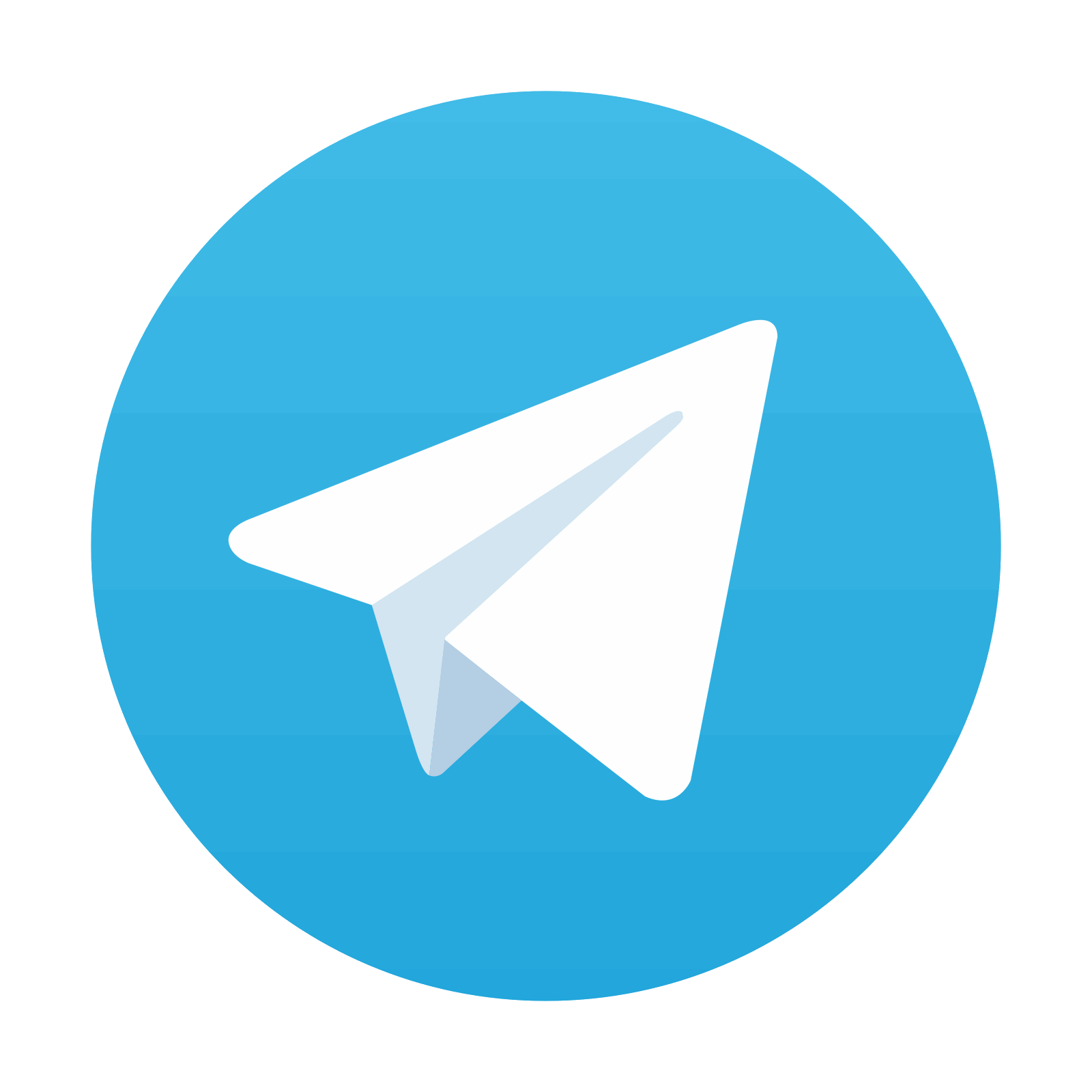
Stay updated, free articles. Join our Telegram channel

Full access? Get Clinical Tree
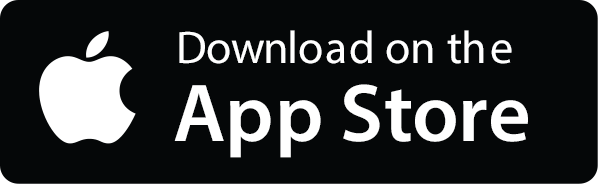
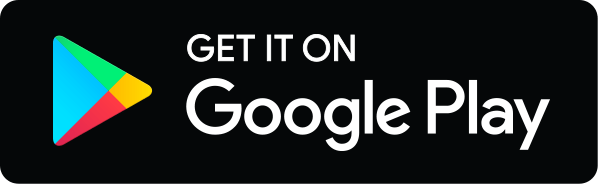