Traumatic brain injury (TBI) remains frustratingly resistant to the advances that have improved outcome from so many other types of injury. Recent progress in resuscitation, hemostasis, imaging, noninvasive management, critical care, rehabilitation, and emergency medical system organization has not yet had a significant impact on reducing the toll of this disease. New and developing insights into our classification and approach to TBI may soon pave the way for meaningful advances. Until then, the clinicians who care for these patients must keep themselves as informed as possible about the limitations and appropriate uses of existing therapies.
TBI has been defined in many different ways. A good working definition is that it is a disruption or alteration of brain structure or function caused by external mechanical forces. The disruption may be variable in severity and may be transient or permanent. The causative external forces are diverse and include rapid acceleration or deceleration, direct compression, penetration and physical disruption of brain tissue, blast and other complex mechanisms, and various combinations of these and other etiologies. Mild TBI may be present with no abnormalities on imaging studies. At the other end of the spectrum, more severe injuries may be associated with large contusions, traumatic hematomas, or other immediately life-threatening structural lesions.
The exact number of people who sustain TBI is unknown since some patients with severe systemic injuries do not survive long enough to undergo a thorough evaluation that would uncover the presence of brain injury. At the other end of the spectrum, many individuals suffering mild or moderate TBI choose not to seek medical care.
Many epidemiologic studies include only those TBI patients who receive medical care in an emergency department. By this criterion, approximately 1.4 million people per year suffer TBI in the United States. Approximately 1.1 million are treated and released, 240,000 are hospitalized, and 50,000 die.1
Common causes of TBI include falls, motor vehicle collisions (MVCs), pedestrian impact, and assault. TBI has a bimodal age distribution, with the greatest risk in 0–4 and 15- to 19-year-olds. Falls predominate at the extremes of age, whereas MVCs are most common in teenagers and young adults. The incidence in males is 1.5-fold higher than in females. The youngest patients are often the victim of abuse and cannot protect themselves. Military personnel comprise a statistically small number of the overall volume of TBI patients, but combat operations cause them to have a higher incidence of penetrating and blast injuries.
TBI is a dynamic process. Management priorities for a given patient may change rapidly as his or her underlying pathophysiology changes. Primary injuries result directly from the forces imparted at the time of the accident. These may include both focal disruption of tissue, such as contusions and hematomas, and diffusely distributed damage.
Secondary insults are defined as those occurring subsequent to the initial impact. These injuries may include hypoxemia, ischemia, seizures, fever, hypoglycemia, and other systemic (non-neurologic) events that can directly impact the brain’s ability to respond to a primary insult. A brief period of mild hypotension or hypoxia may be tolerated quite well by an uninjured brain, but the same minimal secondary insult can be devastating if it occurs soon after a brain injury. A neurosurgeon may spend several hours operating on an acutely injured patient, but the biggest determinant of outcome may be the management that the patient receives in the intensive care unit (ICU) in the days and weeks after admission.
Closed injuries are those in which the overlying scalp remains intact.
Penetrating injuries to the central nervous system are generally considered to be of low velocity when caused by such objects as knives, arrows, lawn darts, and ice picks. High-velocity injuries are caused by missile-type projectiles. If an object is still embedded and protruding, great care should be taken to stabilize it during transport and evaluation. Physical examination should describe any visible wounds, but labeling these as “entry” or “exit” wounds is best avoided until all information is available, including results of detailed imaging studies. Clipping or shaving hair may be required for a complete assessment. Control of vigorous scalp hemorrhage may require suturing or tight wrapping of scalp wounds, which can bleed profusely.
Assessment and resuscitation proceed as in other types of TBI. Computed tomography (CT) scanning should be performed to localize any intracranial projectiles or indriven bone fragments and to characterize the extent of injury. If a foreign body has passed near the location of any major vessels, catheter angiography should be considered once more urgent evaluation and stabilization have been performed.
Evaluation, planning, and operative setup should be undertaken with the foreign body still embedded, and removal should proceed only in the operating room (Fig. 19-1). To help plan the removal, a similar or identical object may be useful to have on hand as a reference. Antibiotics and prophylactic anticonvulsants are usually administered.
Gunshot wounds to the head (GSWH) make up the majority of penetrating cranial injuries. In some parts of the United States, GSWHs are the most common form of TBI. Some studies report an overall mortality rate greater than 90% if on-scene deaths are included, with a mortality rate exceeding 50% among those who are alive upon presentation to a hospital.
Traditionally, GSWH have been divided into those from high-muzzle-velocity rounds (approximately 750–1000 m/s, such as hunting rifles and military weapons) and those from low-muzzle-velocity rounds (approximately 200–500 m/s, as seen in most handguns). With escalating civilian firepower, however, these distinctions are becoming blurred. The above velocities reflect measurements taken as the projectile leaves the barrel of the weapon. As a bullet covers aerial distance, ricochets off objects, or passes through objects, it loses velocity and kinetic energy.
Primary injuries from gunshot wounds include direct injuries to face and scalp, pressure waves of gaseous combustion from the weapon if it is touching the target or fired at close range, coup and contrecoup injuries from missile impact, and destruction of brain or bone along the primary path of the original bullet or secondary paths created by fragments of bullets and bone. High-velocity projectiles also create secondary cavitation that pushes tissue away from the bullet in a cone of injury many times wider than the projectile itself. The vacuum that follows the cavitation may pull surface debris into the wound, potentially creating a nidus for infection. Some specially designed bullets mushroom, fragment, or tumble in order to increase the width of the destructive path. Projectiles can also ricochet off the inner table of the skull.
Secondary injuries from GSWHs mimic those seen in other types of head trauma and include edema, growth of contusions, disseminated intravascular coagulation (DIC), hemorrhage from vessel disruption, ischemia, infarction, and herniation. Late complications can include abscess or traumatic aneurysm formation, seizures, and migration of fragments of bone, bullet, or other debris.
The decision about whether to take a GSWH patient to the operating room may be difficult since many of these patients have devastating and irrecoverable injuries that will not benefit from surgery. The Glasgow Coma Scale (GCS) score is the most useful piece of information for triaging a GSWH patient.2 Patients with GCS scores of 3–4 generally have a poor outcome regardless of CT findings, and for that reason some clinicians do not even obtain CT scans on these patients. Limited treatment is a reasonable course in such cases. Those with a GCS score of 5 are in an indeterminate category. If the GCS score is 6 or higher, most experts would opt for aggressive treatment in the absence of additional significant findings to suggest that a less aggressive course would be preferable. Poor prognostic factors include self-infliction of injuries, the presence of bilaterally fixed and dilated pupils, and the development of coagulopathy. Poor prognosis and high mortality have also been associated with bullet passage across the midline, through the geographic center of the brain, through the ventricles, or across more than one lobe of the brain.3
Basic principles of surgery for GSWH include evacuation of hematomas causing mass effect, meticulous hemostasis, thorough debridement of devitalized tissue and foreign debris, and watertight layered closure to prevent cerebrospinal fluid (CSF) leaks. A “chain of evidence” for forensic purpose should be preserved when bullet fragments are removed.
Diffuse axonal injury (DAI) refers to axonal damage that is caused largely by rotational and other mechanical forces. Mild cases result in axonal stretching and transient neuronal dysfunction. More severe cases may set into motion a complex series of cellular events that cause focal impairment of axoplasmic transport, culminating in axonal disconnection at the site of impairment. In humans, this sequence of events may occur over the course of roughly 6–12 hours, suggesting that a yet-to-be-developed therapeutic intervention might be administered within this time frame. More severe traumatic forces may cause direct mechanical disruption of tissue and immediate disconnection of axons. Subsequent axonal sprouting and attempts to re-establish connectivity may go awry when aberrant neural pathways are created, potentially contributing to the morbidity of severe brain injury. The development of DAI does not require direct impact to the head. It may result from rapid noncontact acceleration or deceleration in a linear or rotational fashion.4
Histologically, DAI-associated lesions are usually microscopic in size and nonhemorrhagic in composition. CT scans may be normal or may show hyperdense petechial hemorrhages. T2-weighted magnetic resonance imaging (MRI) scans may show multifocal hyperintense lesions at gray matter/white matter interfaces, especially in the frontal lobes, corpus callosum, and brainstem. When hemorrhagic DAI is visible on CT or MRI scanning, one must assume that the radiologically visible lesions are just the tip of the iceberg and that more widespread DAI exists.
Injury severity parallels the amount of force required to cause DAI. Prognosis worsens with increasing number of lesions or as lesion depth progresses from the cortex to corpus callosum to brainstem.
Military personnel are at much higher risk than civilians for blast traumatic brain injury (bTBI). Brain injury accounts for approximately 20% of all combat-related injuries in modern wars,5,6 including Operation Enduring Freedom in Afghanistan and Operation Iraqi Freedom. In these two conflicts, bTBI was frequently caused by improvised explosive devices (IEDs). Modern helmets, body armor, rapid transport of injured personnel, and forward-based field hospitals have provided unprecedented rates of warfighter survival and have allowed for a better understanding of the effects of bTBI.
bTBI may be composed of four distinct types of injuries that often overlap. Primary blast injury occurs from overpressure. This has long been known to contribute to injuries in air-filled organs, such as the lungs, bowel, and middle ear. The potential contribution of overpressure to brain injury is currently the focus of much study. Secondary blast injury results from penetration of objects that are set into motion by the explosion. Tertiary blast injury results from a patient being thrown and striking the ground or other object. Quaternary blast injury refers to additional factors not included above, such as heat, toxic fumes and inhalational injury, or hypoxia. Blast injuries in an enclosed space produce an enhanced and complex pattern of forces as energy reflects off walls and objects to impact the head and body at multiple angles and to multiple degrees.
bTBI often includes closed and penetrating TBI components, and many of these patients also have additional serious injuries, such as traumatic limb amputations or hemorrhagic shock. This combination of factors makes it difficult to assess the true contribution of primary or quaternary blast effects on patients who present with TBI after an explosion. In milder cases, combatants may not recognize that they suffer delayed effects of bTBI, or they may knowingly hide their deficits in hopes of remaining with their combat unit.
bTBI can cause headache, confusion, amnesia, altered mental status, and other symptoms associated with concussions. Postconcussive symptoms may be difficult to differentiate from post-traumatic stress disorder, which often coexists with TBI.
Severe bTBI may cause hyperemia and severe cerebral edema early after injury, often requiring decompressive craniotomy. Higher rates of traumatic pseudoaneurysm formation and vasospasm are seen in comparison to civilian closed and penetrating TBI. These vascular pathologies may require more frequent endovascular or open vascular repair.7
Concussion is the mildest form of mild traumatic brain injury (mTBI). It may be defined as a usually transient alteration of neurological function caused by nonpenetrating injury to the brain and characterized by normal imaging studies. Classic symptoms of concussion include headache, irritability, confusion, amnesia, nausea, vomiting, memory problems, difficulty concentrating, vertigo, alterations of balance, anorexia, insomnia, hypersomnolence, impaired coordination, anxiety, and depression. As many as 90% of concussed patients exhibit no loss of consciousness, and when this does occur, it is usually brief. Grading the severity of concussion is currently not recommended because the grading systems that have been described to date have not been shown to correlate with outcome, treatment recommendations, or duration of symptoms.
By definition, CT scanning is unremarkable in these patients. MRI may reveal abnormalities in up to 25% of cases in which CT scans are normal.8 Pathologic specimens show no gross or microscopic parenchymal abnormalities in patients who have suffered a single concussion.
“Second impact syndrome” is a rare but potentially catastrophic sequela of concussion. It is seen most commonly in children and teenagers. These patients are still recovering from the effects of a concussion when they suffer a second one. Within minutes, the patient develops cerebral vascular engorgement, possibly from impairment of cerebral autoregulation caused by the first concussion. Neurological deterioration quickly follows, usually culminating in cerebral herniation and death.
Most important for the treatment of concussion is the recognition of injury. Signs of concussion might be so subtle that they do not prompt the patient or family to seek medical attention. However, returning while still symptomatic to an activity that carries a risk of another concussion, such as contact sports, can be disastrous. All experts agree that a symptomatic player should not return to play until he or she has fully recovered.
Nonaccidental trauma (NAT) is traumatic injury that is deliberately inflicted on infants and children. The concept was first described in infants as an injury triad consisting of long bone metaphyseal fractures, subdural hematomas (SDHs), and retinal hemorrhages9 and has become known in common parlance as “whiplash shaken infant syndrome” or “shaken baby syndrome.”10 Even though it is almost certainly underreported, it is nevertheless recognized as the primary etiology of brain injury death in children less than 2 years of age.
Some medical professionals find management of these cases to be difficult due to awkward discussions with the child’s parents, emotional attachment to the children, frequent lack of accurate information, rarity of admissions of guilt from perpetrators, and the medicolegal implications of child abuse accusations. However, a heightened level of suspicion must be maintained since missed recognition of NAT returns the child to a harmful environment, almost always results in continuation or escalation of the abuse, and may result in the patient’s death.
The two most commonly provided histories are trivial blunt trauma such as a short-height fall from bed or low surface, or complete denial of any history of trauma. Except for the rare epidural hematoma (EDH) with middle meningeal arterial bleeding, low-height falls (head-to-impact distance less than three feet) do not result in life-threatening brain injuries.11,12 In the absence of a history of trauma, the existence of NAT may be indicated by feeding difficulty, emesis, lethargy, irritability, abnormal movements, seizures, unresponsiveness, or apnea.
On imaging studies, NAT may appear as multiple brain injuries that are more severe than expected given the reported history. Injuries may appear to vary in age (some recent, some remote). Impact injuries include skull fractures and superficial scalp lacerations or swelling, in addition to injuries to the underlying brain. There is a high association with other organ injuries. Historically, some fracture patterns have been incorrectly considered suspicious for child abuse. Fractures that are multiple, compound, diastatic, midline, or nonparietal, or that cross suture lines, may denote a greater degree of imparted force, but they are not pathognomonic for NAT.
A shaking mechanism can result in diffusely distributed SDH. The most frequent hemorrhagic finding is a combination of convexity and interhemispheric SDH, often located posteriorly. Some experts believe interhemispheric SDH has the highest degree of specificity for abuse of any intracranial injury. SDH, subarachnoid hemorrhage (SAH), and retinal hemorrhages are far more commonly seen in abused children than in nonabused children. EDHs can occur, but they are much more commonly associated with accidental injury. Retinal hemorrhages are seen in 65–95% of children with inflicted head injuries and may be unilateral or bilateral. However, severe bilateral retinal hemorrhages are occasionally seen in accidental trauma, usually after major application of force via a well-defined mechanism, such as MVC.
In the appropriate circumstances, practically any pattern of hemorrhage or fracture can result from either accidental or inflicted trauma. However, inflicted injury is the only known illness or condition associated with the combination of acute SDH, skeletal fractures, and severe bilateral retinal hemorrhages. The burden of injury is staggering, with 15–38% overall mortality and 60% mortality if the patient is comatose on presentation. Survivors face a 60–70% likelihood of significant neurological handicap.
TBI varies widely in both severity and specific subtype. During a single traumatic event, a patient may be subjected to multiple forces that differ in magnitude, direction, and duration. Although the following injuries are discussed individually, it must be remembered that multiple types of injury may, and often do, occur in the same patient. Furthermore, focal and diffuse injuries often coexist. Thus, initial treatment efforts may focus on immediate evacuation of a patient’s large SDH, but associated DAI may have an even greater influence on ultimate neurological outcome.
Skull fractures can be categorized by the state of the overlying scalp (closed or open), the number of bone fragments (simple or compound), the relationship of bone fragments to each other (depressed or nondepressed), the widening or entry of a fracture into an existing cranial suture (diastatic), and involvement of the cranial vault or skull base. In general, lower-force impacts like falls from standing will create fractures that tend to be linear, closed, and without dural laceration. Higher-force impacts like motor vehicle accidents, falls from heights, or penetrating trauma are more likely to produce comminuted, open fractures with a greater likelihood of underlying dural or cerebral injury. “Ping-pong” fractures are greenstick-type fractures usually seen in newborns due to the plasticity of the skull (Fig. 19-2). They show a local concavity of the skull, without sharp edges, and usually do not require intervention because the skull remodels during growth, with gradual resolution of the cosmetic deformity.13
Clinical signs suggestive of the presence of calvarial fractures include gross deformity and palpable step-offs. Basilar skull fractures may show postauricular or periorbital ecchymosis, hemotympanum or laceration of the external auditory canal, and CSF rhinorrhea or otorrhea. Cranial nerve injuries may be seen with fractures of the cribriform plate (CN I, anosmia), optic canal (CN II, visual deficit), and temporal bone (CN VII, facial weakness; or CN VIII, hearing loss). Severe basilar skull fractures may result in pituitary gland or stalk injury and resultant endocrinopathies. Direct injury to vasculature that penetrates the skull base may result in arterial dissection, traumatic aneurysm formation, or traumatic carotid-cavernous sinus fistula formation and resulting cranial neuropathies, chemosis, bruit, and stroke. Fractures of air sinuses or mastoid air cells may result in meningitis, even years after the initial event.
Most skull fractures can be seen on CT scans. Plain films may be superior to CT for discovering linear calvarial fractures parallel to the skull base (in the plane of CT slice acquisition). They can be differentiated from vascular grooves or normal cranial sutures by the characteristics listed in Table 19-1.14 CT scans provide better visualization of facial and orbital fractures, temporal bone fractures, and pneumocephalus, as well as better detection of air-fluid levels and varying degrees of opacification that may indicate injury to air sinuses and mastoid air cells. Thin-cut bone windows can be reconstructed in coronal and sagittal planes and via three-dimensional surface modeling to aid in fracture characterization and surgical planning. CT angiograms and venograms are useful for assessing fractures involving skull base foramina containing important vasculature, such as the carotid canal and foramen magnum, or fractures that cross major venous sinuses.
Linear skull fracture | Vascular groove | Suture line | |
---|---|---|---|
Density Course Branches Width | Black Straight Usually none Very thin | Gray Curving Often branching Thicker than fracture | Gray Follows known suture course Joins other suture lines Jagged, wide |
Fractures must be assessed and treated in concert with management of any underlying brain injury. The following discussion of skull fracture treatment assumes that evaluation has been conducted and appropriate treatment instituted for subdural or epidural hematomas, parenchymal hemorrhages, contusions, and/or cerebral edema, and that clinical criteria do not separately mandate operative intervention for these lesions.
Closed, nondisplaced fractures do not normally require intervention. Open skull fractures may require debridement and careful inspection, along with prophylactic antibiotics. Those with obvious underlying dural laceration or CSF leakage require layered surgical repair to reduce the risks of meningitis or brain herniation through a dural defect. In the pediatric population, laceration of the underlying dura may rarely lead to development of a growing skull fracture (or leptomeningeal cyst) in 0.05–0.6% of skull fractures.15 Over time, pulsations of the underlying rapidly growing brain widen the dural laceration and fracture line (Fig. 19-3). These are most common in children under 1 year of age, and over 90% occur in children less than 3 years old. Surgical repair includes wide bony exposure to repair the dural edges that often retract beyond the limits of the visible fracture.
Relative indications for surgical elevation of a depressed skull fracture include depression of more than 8–10 mm or more than the thickness of the adjacent skull (Fig. 19-4), a focal neurological deficit clearly attributable to compression of underlying brain, significant inward intrusion of bone fragments (implying possible dural laceration), and persistence of cosmetic deformity after overlying scalp swelling has subsided. For simple depressed fractures without dural violation, there is no evidence that post-traumatic seizure (PTS) risk16 or neurological outcome17 is improved by surgical elevation of the fracture. At least in very young patients, cosmesis may not be improved by surgery because, over time, the growth of the child’s brain induces remodeling of the overlying skull.17 Fractures that cross a major dural venous sinus may warrant a more conservative approach given the increased risks of bleeding and air embolism that may occur during surgical repair.
Current recommendations support surgical repair of open fractures depressed greater than the thickness of the cranium. Surgery is also supported in the presence of significant intracranial hematoma, depression greater than 1 cm, significant frontal sinus or nasofrontal duct involvement, gross cosmetic deformity, wound infection, pneumocephalus, or gross wound contamination. In the absence of gross contamination, primary bone fragments may be replaced without excessive risk of infection.18 Nonoperative management may be preferred for management of open depressed cranial fractures if there is no evidence of dural penetration.
EDH occurs when blood collects in the potential space between the dura and inner table of the skull. Although classically described EDHs arise from arterial bleeding caused by a temporal bone fracture that lacerates the middle meningeal artery, the advent of CT scanning has revealed that many EDHs originate from bleeding of the bony edges of skull fractures. Another common cause is injury to a venous sinus.
On CT scanning, an EDH usually appears as a hyperdense, biconvex (lenticular) mass adjacent to the inner table of the skull (Fig. 19-5). Unless sutural diastasis is present, the EDH is often externally bounded by cranial sutures and may cross the falx or tentorium. Additional associated findings may include SDHs and cerebral contusions. A significant percentage of EDHs managed nonsurgically will demonstrate an increase in size, usually in the first few hours after injury. Some of these lesions may appear in a delayed manner after an initial CT scan failed to reveal their presence.
The classic clinical presentation of an EDH describes a brief post-traumatic loss of consciousness (LOC) followed by a lucid interval of varying duration, proceeding to obtundation, contralateral hemiparesis, and ipsilateral pupillary dilatation. In reality, however, this sequence of events occurs only rarely. LOC is seen in a small minority of cases. Overall mortality from a unilateral EDH has been reported as 5–12%,19 with rates increasing in cases of bilateral EDHs, posterior fossa location (25% mortality), and concurrent acute SDH.
Rapid diagnosis and timely intervention are paramount to optimize outcome. Surgical guidelines suggest that an EDH >30 cm3 in volume should be evacuated regardless of GCS score. EDH <30 cm3 in volume and <15 mm of thickness and <5 mm midline shift may be treated conservatively at a neurosurgical center with frequent neurological examinations and serial CT scanning.18 Relative indications for evacuation of EDHs include the presence of neurological symptoms or maximal hematoma thickness >1 cm. Acute EDH patients in coma (GCS score ≤8) and with anisocoria should undergo surgical evacuation as soon as possible.18
Posterior fossa injury is rare, comprising <3% of head injuries. However, a disproportionately large proportion of these lesions are EDHs. The limited volume of the posterior fossa and the potential compromise of the brainstem and CSF pathways underscore the importance of rapid evacuation via suboccipital craniectomy.18 Patients without signs of mass effect or neurological deterioration may be watched conservatively, with serial neurological examinations, CT scans, and a low threshold for surgical intervention.
SDH occurs when blood collects between the arachnoid and inner dural layers of the meninges. Thus, from a strict anatomic point of view, these are really intradural hematomas. A common cause is traumatic stretching and tearing of cortical bridging veins that cross the subdural space and drain into the dura or into a dural sinus. The force may be applied by direct impact or by indirect linear or rotational motion. Less common etiologies include coagulopathy, subdural dissection of parenchymal hematomas, and rupture of a vascular anomaly into the subdural space. Patients with cerebral atrophy, cranial CSF shunts, and large middle fossa arachnoid cysts are predisposed to SDH because of increased traction on cortical veins. Many patients with acute SDHs also have other significant intracranial lesions.
SDHs are commonly located over the hemispheric convexities and may cover part or all of a hemisphere. Classically, they are crescent-shaped, may cross calvarial suture lines, and layer along the falx or tentorium (Fig. 19-6). Patients may present with symptoms of mass effect or of more diffuse underlying brain injury. Chronic SDH may present more insidiously, with progressively worsening headaches and/or focal deficits.
The appearance of SDHs evolves over time. On CT scanning, acute SDHs are usually hyperdense, but some may show areas of mixed density if ongoing active bleeding causes hyperacute (and not-yet-clotted blood) to continue to accumulate in the subdural space. Some acute SDHs may be isodense in the presence of coagulopathy, significant anemia, or admixing of blood and CSF. Subacute SDHs are isodense to brain, and chronic SDHs become hypodense. Inward displacement of the gray/white cortical ribbon and cortical vessels may be evident on contrast-enhanced CT scanning. The subdural membranes that often exist with chronic SDHs may appear within 4 days and enhance with contrast administration.20 MRI may show evolving signal intensities that vary with the age of the SDH as blood breakdown products are converted to oxyhemoglobin, deoxyhemoglobin, methemoglobin, and hemosiderin (Table 19-2).
SDHs often have worse outcomes when compared to EDHs of similar size. EDHs are frequently of arterial origin and thus present with symptoms quickly, facilitating prompt diagnosis and treatment. In contrast to EDHs, it has been postulated that many SDHs result from higher-magnitude forces, which cause greater damage to the brain and cortical vessels. Because of the venous origins of the bleeding, a SDH may require more time to grow and become symptomatic. The attribution of symptoms to diffuse brain injury has also been postulated to delay diagnosis in some cases until the signs of midline shift and brainstem compression become evident. Mortality associated with SDH can be high and may be related more to the underlying brain injury than to the SDH itself. Mortality rates increase in the elderly and in patients on anticoagulants.21
Guidelines propose that an acute SDH with thickness >1 cm or a midline shift >5 mm should be evacuated regardless of GCS score. Comatose patients (GCS score ≤8) with an acute SDH <1 cm in thickness and midline shift <5 mm should undergo SDH evacuation if the GCS decreases by 2 points between the time of injury and hospital admission, if they present with pupils that are asymmetric or fixed and dilated, or if intracranial pressure (ICP) reaches or exceeds 20 mm Hg.18 A craniotomy to evacuate an acute clot should be performed as soon as possible. Over time, as solid blood clots pass through subacute to chronic stages, they liquefy and may be amenable to drainage via burr holes.
Traumatic intracerebral hematomas (TICHs) and contusions may expand rapidly. Worsening perilesional edema and rapid hematoma growth may produce increasing mass effect and neurological deterioration. If patients develop neurological decline referable to an expanding TICH, surgical intervention may be warranted. Surgical decompression is often necessary in patients when TICH volume exceeds 50 cm3 or when patients present with GCS scores of 6–8 with frontal or temporal contusions >20 cm3 in volume with midline shift ≥5 mm and/or cisternal compression on CT scan.18 Surgical procedures range from localized frontal or temporal craniotomy with resection of underlying focal clot to more extensive craniectomy with duraplasty, evacuation of severely contused brain, and temporal lobectomy.
Subarachnoid blood occupies the space between the pial and arachnoid membranes. Traumatic subarachnoid hemorrhage (tSAH) results from venous tears in the subarachnoid space. The injured vessels are often quite small, and the resulting hemorrhage frequently appears as a thin, diffuse hyperdensity over the sulci and gyri of the cerebral convexity (Fig. 19-7) and as a thin hyperintensity on fluid-attenuated inversion recovery (FLAIR) MRI. It may be confused with FLAIR hyperintensities seen in patients with inflammatory conditions or those breathing 100% oxygen or receiving propofol. The usual convexity location of tSAH distinguishes it from SAH caused by spontaneous rupture of a cerebral aneurysm, in which the blood is usually most prominent in the basilar cisterns and appears thicker on CT scanning. Occasionally, tSAH can have a CT appearance similar to that of aneurysmal SAH, and if a detailed history cannot help distinguish between spontaneous and traumatic SAH, imaging of the vasculature via CT, MR, or catheter angiography may be required. In general, no specific treatment is needed for tSAH, and patient management is dictated by associated injuries.
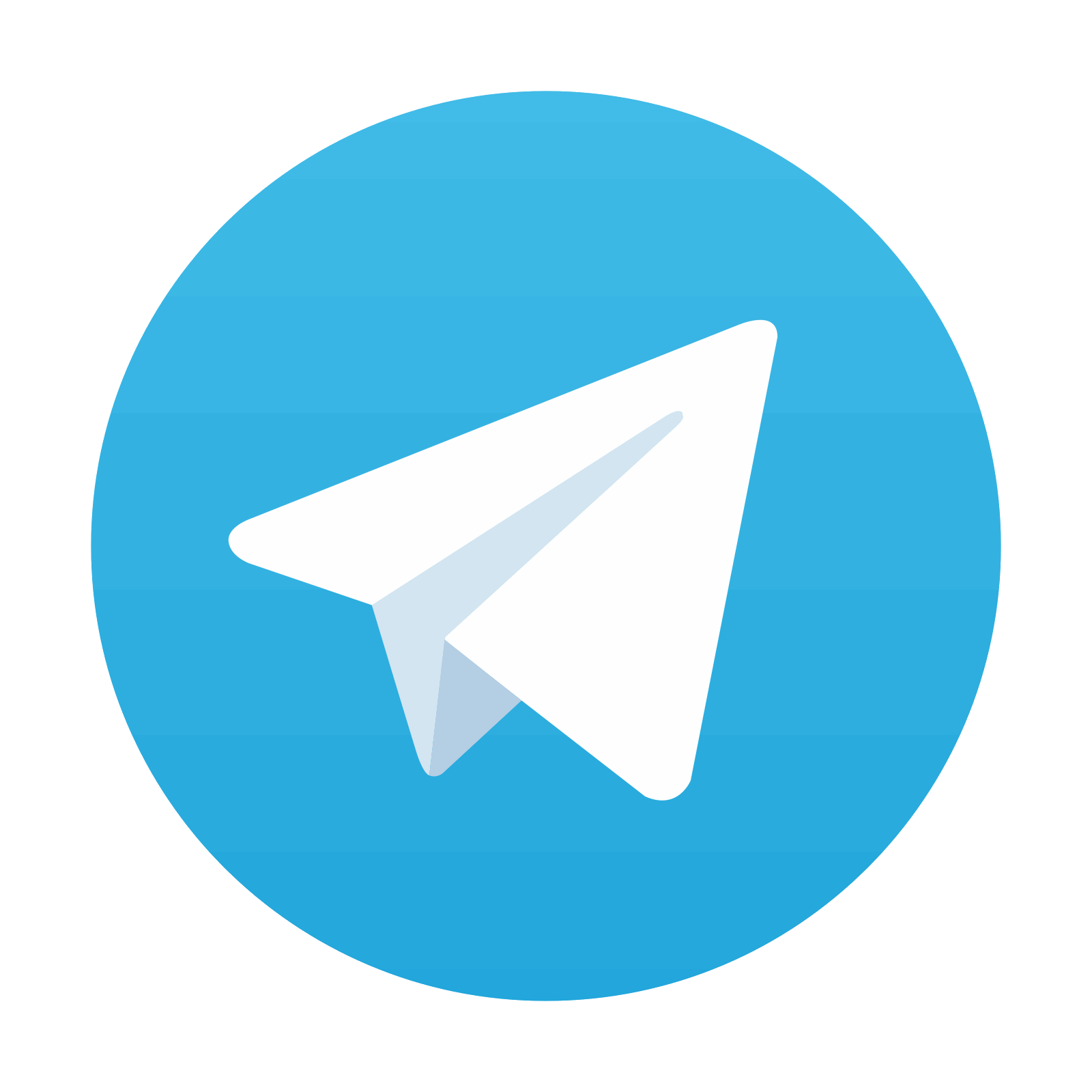
Stay updated, free articles. Join our Telegram channel

Full access? Get Clinical Tree
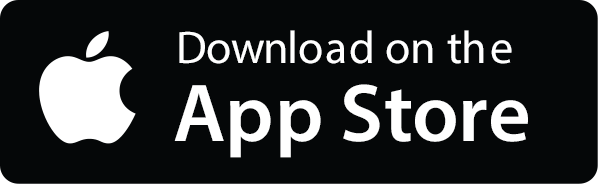
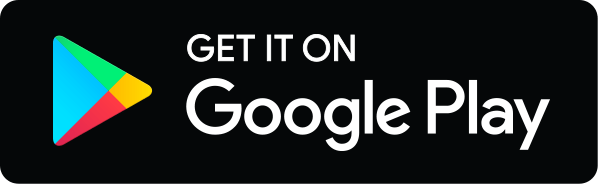