Epidemiology
Thoracoabdominal aneurysms (TAAs) develop in patients with atherosclerotic disease, chronic aortic dissection, and connective tissue disorders (e.g., Marfan syndrome, Ehler–Danlos syndrome).
Pathophysiology
Pathologic processes include chronic inflammation, remodeling of extracellular matrix, and depletion of vascular smooth muscle cells. Elastin depletion in the media layer of the aortic wall is a common finding.
Clinical features
Approximately 80 percent of TAAs are atherosclerotic in nature, associated with smoking, hypertension, hypercholesterolemia, and advanced age. Approximately 20 percent of TAAs develop from chronic aortic dissections and occur in patients with connective tissue disorders. The risk of rupture increases rapidly when aneurysmal diameter reaches or exceeds 6 cm. The most common presentation includes abdominal or back pain, although many TAAs are also discovered incidentally.
Diagnostics
TAAs are classified commonly according to the anatomic scheme developed by Crawford (types I through V). Helical computed tomography and magnetic resonance angiography are the diagnostic modalities of choice, largely having supplanted angiography.
Treatment
Medical therapy includes the reduction of risk factors for aneurysmal expansion (e.g., smoking, hypercholesterolemia) and tight blood pressure control predicated on the use of β-blockers.
Interventional therapy includes open surgical, hybrid and totally endovascular repair. Spinal cord protection is of particular concern in all methods of repair.
Outcomes
In-hospital mortality rates after surgery range between 8 and 15 percent in most series. Common postoperative complications include bleeding, respiratory failure, myocardial infarction or cardiac failure, renal failure, and paraplegia. Operative risk increases with advanced age, aneurysm complexity, emergency operation, coronary and cerebrovascular disease, pulmonary disease, and renal failure. Outcomes may be improved with the distal perfusion approach to repair. Five-year survival rates range between 53 and 73 percent.
Since its introduction by Michael DeBakey in 1956,1 thoracoabdominal aneurysm (TAA) repair generally presents the most technically challenging and controversial issues in vascular surgery. The surgical repair of TAAs is an undertaking that requires substantial preoperative planning and consideration of a wide array of techniques. Each of these techniques has its champions, and several authors have published comparably good results using widely different methods. In this chapter, the common principles that are applicable to all TAA surgery are described. In addition, most of the surgical techniques currently in use to repair these aneurysms are discussed, with emphasis on those that are preferred at the Johns Hopkins Hospital.
Insight into the pathophysiology of aortic aneurysm formation at the cellular level has come into focus only recently. The pathologic processes observed in aneurysm development include chronic inflammation, remodeling of the extracellular matrix, and depletion of vascular smooth muscle cells. A basic feature common to all aneurysms is the depletion of elastin, a key structural protein in the media layer of the aortic wall. Normal turnover of matrix proteins is mediated by a family of enzymes called matrix metalloproteinases (MMPs); their activity is regulated by proteins called tissue inhibitors of metalloproteinases (TIMPs). Several MMPs have elastase activity. In separate studies, MMP-2, MMP-3 (stromelysin-1), MMP-9, and MMP-12 [human macrophage elastase (HME)] have been found to have increased activity in aneurysmal tissue.2,3
Other components of the inflammatory response appear to play a role in aneurysm formation. The complement fixation pathway seems to be involved in matrix proteolysis, as large amounts of complement-fixing immunoglobulin G (IgG) and complement C3 have been detected in aortic aneurysms.4 Increased amounts of the inflammatory cytokines tumor necrosis factor-α (TNF-α), TNF-β, prostaglandin E2, and interleukin 6 also have been documented in aneurysm tissue.5,6 Finally, smooth muscle cells are eliminated at least in part by apoptosis, an active process of cell death associated with increased protein synthesis. This depletes a population of cells that are capable of directing connective tissue repair.7 All these findings suggest that aortic aneurysm formation is the result of a complex interaction of inflammatory mediators and structural components involved in maintaining the extracellular matrix.
A majority of TAAs are atherosclerotic in nature and, as with abdominal aortic aneurysms (AAAs), are related to smoking, hypertension, high cholesterol, and advanced age. TAAs are the most common type of aneurysm to develop in patients previously diagnosed with AAAs, occurring in 6 percent of those patients. Approximately 20 percent of TAAs occur in patients who develop chronic aneurysmal dilatation after an aortic dissection and in patients with connective tissue disorders, most notably Marfan syndrome and Ehlers–Danlos syndrome. Compared with AAAs, the natural histories of TAAs have not been well documented owing to the lower incidence of TAAs and the fact that the advanced imaging techniques required to diagnose TAAs have been in general use only for the past two decades. In most patients, slow progressive dilatation ultimately leads to death from rupture if it is not treated (Fig. 40-1).8–12 The other major cause of death in this population of patients is cardiac disease. The rate of aneurysmal dilatation is approximately a 10-percent increase in diameter per year, with higher average rates of growth in patients with TAAs resulting from aortic dissection. The risk of rupture is related to aneurysm size. It is low below 6 cm but increases dramatically with growth in maximum diameter beyond 6 cm; most vascular surgeons recommend conservative therapy for patients with TAAs that are less than 6 cm in diameter and repair if they are more than 6 cm in patients who are acceptable surgical candidates. However, Cambria and coworkers found a higher rate of rupture in patients with TAAs larger than 5 cm.13 TAAs that result from aortic dissection have been found to be at higher risk for rupture than are atherosclerotic TAAs in separate studies done by Crawford and Denatale and by Pressler and colleagues.8,14 In addition, Juvonen and colleagues found that patients with TAAs resulting from chronic dissections tended to experience rupture earlier.15 In that study, the median last known diameter of the aorta in patients who had a rupture was 5.4 cm. Additional risk factors for rupture in that study were chronic obstructive pulmonary disease (COPD) and poorly controlled hypertension. One therefore may consider repair of a TAA that is less than 6 cm in the setting of chronic dissection, particularly in a patient with poorly controlled hypertension and lung disease.
Figure 40-1
Kaplan–Meier probability survival curves from date of diagnosis and date of admission in 94 patients. Numbers of patients at risk at 12, 24, 36, and 48 months are in parentheses. (Reproduced with permission from Crawford ES, DeNatale RW. Thoracoabdominal aortic aneurysm: Observations regarding the natural course of the disease. J Vasc Surg 1986;3:578–582. Copyright Elsevier.)

TAAs usually are classified according to the anatomic scheme developed by Crawford and coworkers.16 They are classified as types I through IV. A type I TAA begins in the proximal thoracic aorta and extends to the level of the celiac artery. A type II TAA is the most extensive variety, generally involves the entire thoracic and abdominal aorta, and accounts for a large proportion of TAAs that develop years after an extensive aortic dissection. A type III TAA is defined by its proximal extent in the midthoracic aorta, generally is described in the region of the T6 vertebra or the inferior pulmonary vein, and may involve varying amounts of the abdominal aorta. A type IV TAA begins above the celiac axis and extends into the infrarenal aorta. Huynh and colleagues modified the original Crawford classification to include a type V TAA, which begins in the middescending thoracic aorta and terminates at the level of the renal arteries (Fig. 40-2).17 This classification not only reflects what is encountered at surgery but is important for reporting results, since more extensive aneurysms generally are associated with more complications. Aneurysms that involve only the descending thoracic aorta are not included in this classification and are considered separately, although many of the same principles of TAA repair are used to repair thoracic aneurysms.
Figure 40-2
Modified Crawford classification of thoracoabdominal aortic aneurysms. Extent I: from distal to left subclavian artery to above renal arteries. Extent II: from distal to left subclavian artery to aortic bifurcation. Extent III: from sixth intercostal space to aortic bifurcation. Extent IV: from diaphragm to aortic bifurcation (total abdominal aorta). Extent V: from sixth intercostal space to above renal arteries. (Reproduced with permission from Huynh TTT, Miller CC, Estrera AL, et al. Determinants of hospital length of stay after thoracoabdominal aortic aneurysm repair. J Vasc Surg 2002;35:648–653. Copyright Elsevier.)

Most TAAs are discovered as incidental findings on plain x-ray, computed tomography (CT), or magnetic resonance imaging (MRI) studies obtained for other reasons. Up to 40 percent of patients with TAAs become symptomatic.18 The symptoms commonly include acute abdominal or back pain. The development of severe acute pain is considered indicative of an impending rupture, and urgent repair generally is recommended within 24 h of presentation. However, the author has observed patients with histories of moderate subacute pain that had been present for weeks to months and resolved with surgical repair; it appears that not all patients with pain are at risk for imminent rupture. Less commonly, TAAs also present with gastric outlet obstruction, renal failure, aortic thrombosis, or failure to thrive. Rupture presents as acute pain with manifestations of shock or sudden death.
Currently, there are no recommended screening tests for TAAs because of their low incidence (1 per 100,000 population19) and substantial diagnostic imaging costs. Aortic aneurysms are found on plain x-ray studies about 25 percent of the time. Ultrasound is not useful since the suprarenal aorta is often difficult to image and the thoracic aorta cannot be imaged with this modality. The main diagnostic modalities used for TAAs are CT scanning, MRI, and angiography. A CT or MRI is mandatory for obtaining reliable data on the outer diameter of the aneurysm. A high-quality axial study may provide adequate information for operative planning in straightforward cases; however, helical CT or MRI angiography (MRA) with three-dimensional (3-D) reconstruction is important in defining arch involvement or pathology, especially in visceral branch vessels. Because of the high resolution of these studies, formal angiography rarely is required for preoperative planning (Fig. 40-3). In the author’s institution, a helical CT scan with 3-D reconstructions is the imaging method of choice. It provides virtually all the information required to plan surgical repair with data that are immediately accessible to the surgeon on a digital workstation. The software for 3-D reconstructions is very user friendly and permits retrieval and manipulation of images with minimal assistance from technicians. In contrast, MRA is slow and expensive and requires extensive postprocessing. It is more prone to false positives for occlusive disease of branch vessels, and reviewing the digital images requires extensive technical assistance. In the author’s practice, MRA is reserved for patients with contraindications to CT angiography, such as contrast allergy and renal insufficiency. Aortography played an important role in preoperative planning for TAA repairs in the past, but this modality has been replaced to a large extent by the high-quality imaging of CT and MRA. Nevertheless, the author does use aortography to identify and locate the spinal artery of Adamkiewicz (see below). Recently, however, Japanese investigators have been able to identify this elusive but important structure with CT and MRA.20,21 The author’s institution has not been able to reproduce the Japanese experience reliably thus far.
Medical therapy consists of controlling risk factors for continued aneurysmal expansion. These patients should be advised to quit smoking, and blood pressure and cholesterol should be controlled strictly. β-Blockers have been advocated to decrease the expansion rate of aortic aneurysms, but a randomized controlled trial conducted in Canada did not confirm their effectiveness.22 Doxycycline, a known metalloproteinase inhibitor, protects against aneurysm expansion in animal models, and one randomized trial demonstrated decreased expansion rates with daily administration of this drug in a phase 2 trial.23 However, it is premature to recommend chronic doxycycline therapy to all patients with aortic aneurysms.
Because of the high-risk nature of TAA repair, appropriate decision making is imperative in the selection of patients for surgery and in the performance of the surgical procedure to obtain good results (see Fig. 40-4). There are three important components to preoperative evaluation and planning in TAA surgery: determining operative risk, planning the extent and technical sequence of the repair, and selecting surgical adjuncts to minimize morbidity.
The determination that TAA repair is indicated for a patient assumes that the risk of observation is greater than the risk of repair. It is therefore necessary to have a reasonable understanding of a patient’s perioperative risk. Postoperative morbidity and mortality is related largely to the cardiac, pulmonary, and renal systems; hence, a thorough evaluation of those systems is imperative. At a minimum, cardiac function should be evaluated with an echocardiogram. An abnormal electrocardiogram (ECG), a history of coronary artery disease or symptoms of chest pain, congestive heart failure, or poor exercise tolerance should prompt evaluation by a cardiologist and some form of stress testing. Dobutamine echocardiography is considered to be contraindicated in patients with aneurysms because of the possibility of precipitating rupture, and so stress thallium scanning and formal exercise testing are the screening diagnostics of choice. Cardiac catheterization should be performed if reconstruction of the ascending aorta or aortic arch is anticipated. Pulmonary function tests, including spirometry and a room air blood gas, should be obtained, since single-lung ventilation usually is required intraoperatively. Renal function and hepatic function are evaluated with blood chemistries. An individualized decision to operate is based on an integration of the estimated risks of rupture and repair for a specific patient after this information has been acquired.
With TAA repair, it is imperative that the surgeon plan the entire operation in advance and anticipate the extent of and steps in the planned repair. This includes the location of the proximal and distal extent of the repair, the location and sequence of aortic clamping, and plans for reimplanting, bypassing, or reconstructing aortic branch vessels. This is important for several reasons. First, the extent of the repair will determine the level of the thoracotomy and the extent of the incision. It also will determine which adjuncts are required intraoperatively, such as cardiopulmonary bypass equipment and neurophysiologic monitoring. The most important consideration in preoperative planning relates to the pathology of the aortic arch. For TAAs involving the proximal descending thoracic aorta, it is crucial that one appreciate the patient’s arch anatomy, since arch involvement may warrant the participation of a cardiac surgeon to conduct the cardiopulmonary bypass and hypothermic circulatory arrest. With extensive arch involvement, it may be necessary to perform the repair in stages. For example, in cases in which the aortic arch and the descending thoracic aorta are diseased, the arch can be replaced first, leaving a graft extension (i.e., ‘elephant trunk’) in the descending thoracic aorta. The descending thoracic aorta is repaired several months later, at which time the new graft replacing the descending thoracic aorta is sewn to the elephant trunk proximally. This staged approach obviates the perilous prospect of clamping and anastomosing the graft to a diseased aortic arch.
The next important consideration in preoperative planning is the way one plans to prevent spinal cord and other end-organ damage from intraoperative ischemia. Multiple strategies are available to accomplish this goal. With respect to spinal cord protection, they include (1) spinal artery conservation, (2) selective intercostal artery conservation, (3) intraoperative monitoring with somatosensory evoked potentials (SSEP) or motor evoked potentials (MEP), (4) systemic hypothermia, (5) regional hypothermia, (6) lumbar drainage of cerebrospinal fluid (CSF), (7) distal aortic perfusion, either passive or active, (8) aortic conservation with aortic ‘tailoring,’ and (9) pharmacologic manipulation. With respect to visceral protection, the options include regional or systemic hypothermia and distal aortic perfusion.
The most detrimental form of intraoperative ischemia that can occur during TAA repair is spinal cord ischemia, which can occur in up to 40 percent of TAA repairs. The clinical manifestations include a spectrum of spinal cord deficits that can be observed immediately after surgery or up to 3 days later. The most severe form results in complete paraplegia and usually is associated with a mortality rate over 50 percent. The spinal cord becomes ischemic as critical blood flow through intercostal arteries is interrupted when these intercostal arteries are ligated in the course of the aneurysm repair. Over 100 years ago, it was recognized that in humans, a single intercostal artery often gives rise to a branch that provides a major proportion of blood flow to the anterior spinal cord: the spinal artery or the great radicular artery of Adamkiewicz. This artery usually arises from a left-sided intercostal artery between the eighth thoracic (T8) and the first lumbar (L1) vertebrae and has a characteristic radiographic appearance. Animal studies have suggested that preserving the spinal artery can prevent paraplegia.24 Clinical experience at the Johns Hopkins Hospital and elsewhere has confirmed that preserving the spinal artery in patients undergoing TAA repair can result in improved neurologic outcomes.25,26 Therefore, at the author’s institution, surgeons make an effort to identify the spinal artery of Adamkiewicz preoperatively by performing selective angiography of patent intercostal arteries in the lower descending thoracic aorta (Fig. 40-5). With careful technique, this blood vessel can be identified in up to 86 percent of cases.27 All efforts are made to conserve the involved intercostal artery at the time of surgery by reimplanting or bypassing to the aortic segment from which this intercostal arises or by including this area of aorta within the proximal or distal anastomosis. At the author’s institution, it has been found that identifying and preserving the spinal artery is particularly beneficial in repairs of atherosclerotic TAAs, in which the spinal artery is more likely to be one of the few intercostals that are not chronically occluded by aortic thrombus.28 In this subset of patients, spinal artery identification and preservation resulted in no cases of spinal cord injury in a group of 45 patients. Other authors dispute the value of identifying and preserving the spinal artery.29,30
If the spinal artery is not identified, consideration still is given to reimplantation of intercostal arteries in the lower thoracic region. Safi and colleagues and Cambria and coworkers have shown in separate studies that intercostal artery reimplantation in the T8 to L1 region improves neurologic outcomes,31,32 and Jacobs and colleagues have reported outstanding results with intercostal artery reimplantation guided partially by MEP.33,34 The disadvantage of nonselective intercostal artery reimplantation is that multiple intercostals generally must be reimplanted to assure that adequate spinal cord circulation has been maintained in this scenario. Reimplanting multiple intercostals requires a large intercostal patch, which can become aneurysmal in the future, or multiple small patches. The extra suture lines increase the length and complexity of the operation as well as the risk of bleeding. This approach is particularly unappealing to those who employ the clamp-and-sew method of TAA reconstruction, since without distal aortic perfusion, the time that is devoted to reimplanting intercostals adds to the visceral ischemic time because the visceral branch vessels generally are reimplanted after the intercostals.
To help limit intercostal reimplantation, attempts have been made to use intraoperative neurophysiologic monitoring to identify important intercostals and guide selective reimplantation of those vessels. Initial attempts at neurophysiologic monitoring were performed by measuring SSEPs. Laschinger and associates published early work in dogs,35 showing that SSEP was a sensitive detector of spinal cord ischemia and that distal aortic perfusion could prevent spinal cord ischemia. Subsequent clinical work by that group showed that SSEP loss for more than 30 min predicted spinal cord injury in TAA repair.36 SSEP is limited in that it detects ischemia primarily in the dorsal spinal cord and can miss ischemic injury in the ventral horns of the spinal cord, where the motor tracts are situated.37 As a result, intraoperative monitoring of MEPs has been proposed to overcome this limitation,38 and clinically, MEP monitoring has been shown to be more accurate than SSEP monitoring in detecting spinal cord ischemia.39,40 Most clinical experience with this technique comes from Holland, where separate groups have had excellent results using MEP to prevent spinal cord ischemia.33,34,41–43 Using MEP to guide selective intercostal artery reattachment, Jacobs and colleagues achieved paraplegia rates of under 3 percent in a series of almost 200 patients.34 The author’s group has been using MEP since 2000 but has had difficulty reproducing those results. This group has noted a delayed appearance of abnormal MEP signals that can occur with isolated ischemia of the thoracic spinal cord.44
The development of a hypothermic environment around the spinal cord confers at least partial protection from ischemia during TAA repair. In animal experiments, Colon and coworkers demonstrated that systemic hypothermia protected the spinal cord from periods of ischemia up to 45 min.45 The benefits of hypothermia can be realized at moderate temperatures (32°C) without incurring the risk of cardiac arrhythmias that occurs at lower temperatures, particularly below 30°C. Moderate hypothermia can be achieved passively or actively with a heat exchanger in a cardiopulmonary bypass circuit; the latter technique is preferred since it allows for active rewarming of the patient at the conclusion of the repair. The disadvantages of this approach include a higher propensity for bleeding stemming from the required anticoagulation and coagulopathies associated with cardiopulmonary bypass and variability in the availability of cardiopulmonary bypass support for some vascular surgeons. In the most extreme form of systemic hypothermia, TAA repair can be performed under profound hypothermia (18°C) and circulatory arrest, as advocated by Kouchoukos and coworkers in this country and by others elsewhere.46–48 Kouchoukos and coworkers reported very low rates of spinal cord injury with this approach.46 In the author’s practice, this strategy is applied selectively to difficult distal aortic arch anatomy, since circulatory arrest is not without significant morbidity, including neurocognitive dysfunction. Safi and colleagues reported that profound hypothermia and circulatory arrest did not offer significant benefit compared with moderate hypothermia and partial bypass.49
It is also possible to develop spinal cord hypothermia without systemic cooling by using a regional infusion of a cold solution via an epidural catheter. This technique has been championed by Cambria and colleagues at the Massachusetts General Hospital, who have been able to achieve excellent results with respect to spinal cord injury without systemic hypothermia or distal aortic bypass.32,50,51 With this technique, it is necessary to protect the viscera during extensive TAA reconstructions with cold regional perfusion of those organs as well. Despite good published results, the technique has not been adapted widely at other centers.
When the proximal thoracic aorta is clamped, the intracranial and CSF pressures rise. Conversely, blood pressure distal to the clamp drops dramatically. Spinal perfusion pressure, which is represented by mean arterial pressure of the spinal cord minus CSF pressure, therefore drops markedly. CSF drainage reduces CSF pressure in the spinal canal and therefore improves spinal perfusion pressure when spinal perfusion is low intraoperatively as well as in the postoperative period, when CSF pressure may rise as a result of the spinal cord edema that accompanies ischemia–reperfusion injury. Blaisdell and Cooley first demonstrated that decreasing spinal fluid pressure (pharmacologically with intravenous urea) can have a beneficial effect on paraplegia rates in dogs.52 Subsequently, it was found anecdotally to be useful in resolving delayed-onset paraplegia in the early postoperative period.53 However, a small prospective randomized study performed by Crawford and coworkers found that CSF drainage had no effect on paraplegia rates.54 Eleven years later at the same institution, Coselli and colleagues found a statistically significant benefit with CSF drainage in a repeat study, lowering neurologic deficits from 13 percent in the control group to 2.6 percent in the treatment group.55
Two fundamental surgical approaches are employed in TAA repair: (1) the so-called clamp-and-sew approach and (2) distal aortic perfusion techniques. The clamp-and-sew method originally employed by DeBakey and Crawford has been employed for over 40 years and still is used by some accomplished aortic surgeons, particularly in patients with less extensive aneurysms.56–59 In this approach, the aorta is clamped sequentially during the construction of the proximal anastomosis, the reimplantation of critical branches, and the construction of the distal anastomosis. Repair can be performed quickly by an experienced surgeon with less blood loss, since intraoperative anticoagulation is not necessary with this method. However, this technique can expose the viscera to perilously long ischemic times if technical difficulties are encountered with the proximal anastomosis or intercostal artery reimplantation, resulting in end-organ ischemia.
The distal aortic perfusion strategy maintains perfusion of the aorta and its branches distal to the area where the clamps are placed while an anastomosis is created. Distal perfusion can be passive or active. Examples of passive distal perfusion include the Gott aortic shunt,60–62 an axillofemoral bypass that is constructed before the thoracotomy is begun,63 and an ‘octopus’ of catheters whose proximal ends are placed in the Dacron tube graft after the proximal anastomosis is completed and whose distal ends are placed in and perfuse the orifices of the renal and mesenteric arteries while the distal thoracic aorta is repaired.64 Active distal aortic perfusion involves pumping blood through a centripetal pump into the abdominal aorta while the thoracic aorta is clamped. At the Johns Hopkins Hospital, surgeons believe that this technique is the standard of care in TAA repair as it has several important advantages. A heat exchanger attached to the pump circuit permits active cooling of the patient and, more important, active rewarming at the end of the procedure, when hypothermia contributes to coagulopathy. The advantage of being able to rewarm the patient actively to physiologic temperatures cannot be overstated. The flow in the pump circuit can be adjusted to manipulate the blood pressure proximal and distal to the aortic cross-clamp; typical pump flows range between 1.0 and 2.5 L/min. Proximal hypertension and distal hypotension are corrected by increasing pump flow; proximal hypotension and distal hypertension are corrected by decreasing pump flow. An obstruction to inflow or outflow can be detected by high resistance that is noted in the circuit but would go undetected in a passive shunt. The circuit can be converted quickly to a full cardiopulmonary bypass circuit if the patient experiences cardiac arrest or it becomes apparent intraoperatively that circulatory arrest is required to complete a difficult proximal anastomosis near the aortic arch. Numerous centers have demonstrated improved results in TAA surgery with distal aortic perfusion, and it is the preferred adjunct in most centers.65–69
The author’s group prefers the use of nonselective distal aortic perfusion, in which the abdominal aorta is perfused via the left femoral artery while the thoracic aortic reconstruction is performed. The patient is cooled to 32°C before spinal cord or visceral ischemia occurs. The mesenteric and renal arteries are clamped and not perfused while the segment of abdominal aorta containing those branches is reconstructed. The author’s institution finds that mesenteric and renal revascularization can be accomplished within 30 to 60 min and that renal failure and clinically significant visceral ischemia rarely are encountered with this technique. However, other investigators have shown improved results with selective distal perfusion using direct cannulation of the renal and mesenteric arteries while the visceral abdominal aorta is clamped. Jacobs used selective perfusion (with isothermic blood) in 73 patients and had excellent results, with permanent renal failure occurring in only 1 patient (1.4 percent) and transient dialysis required in 5 (6.8 percent). Koksoy and associates70 and Hassoun and associates71 independently undertook clinical trials to determine whether intraoperative cold perfusion of the viscera could minimize ischemic injury and improve postoperative outcomes, particularly with respect to renal function. Their techniques and findings differed, but both studies found some benefit to cold visceral perfusion. In the Koksoy trial, the kidneys were perfused with cold crystalloid containing steroids and mannitol, and the mesenteric vessels were perfused with warm blood from the left heart bypass circuit. In that study, renal failure was decreased in the cold perfusion group.70 In the Hassoun trial, all the visceral vessels were perfused selectively with blood that had been cooled to 4°C by being passed through a heat exchanger. The investigators in that trial found no difference in the rate of renal failure but did find improved survival in the group that received cold perfusion.71
Occasionally, one encounters a moderately dilated distal thoracic aorta while replacing a large aneurysm of the more proximal thoracic aorta in which formal aortic replacement may not be warranted but it may be desirable to try to prevent aneurysmal degeneration of this distal segment to avoid additional surgery in the future. Similarly, it is sometimes necessary to operate on a nondilated aortic segment to correct malperfusion from an aortic dissection. In these situations, it is often preferable not to replace the involved aorta to avoid spinal cord and visceral ischemia. This can be accomplished by using an aortic ‘tailoring’ procedure developed by G. Melville Williams at the Johns Hopkins Hospital. With this approach, a longitudinal aortotomy is made (in the case of an aortic dissection, the intimal flap subsequently is resected), and the aorta is closed with to a diameter of approximately 2 cm to decrease wall tension and prevent further aneurysmal degeneration. This procedure eliminates the need to sacrifice or reimplant any aortic branches and can be performed with very short cross-clamp times. Williams and colleagues first reported this technique in the treatment of patients with chronic dissecting aneurysms72 and then applied it to the management of acute aortic dissections complicated by malperfusion.73 Surgeons at the author’s institution routinely tailor a segment of aorta that is up to 4 cm in diameter and occasionally tailor even larger segments. Since Williams began performing this procedure, reoperation has not been required on any tailored segments for subsequent aneurysmal degeneration, with the exception of patients with connective tissue disorders; such disorders, including Marfan syndrome and Ehlers–Danlos syndrome, are contraindications to the aortic tailoring approach.
Most surgeons administer intravenous mannitol before any identified periods of renal ischemia. Mannitol is an osmotic diuretic and free radical scavenger that preserves urine flow after renal ischemia. It does not necessarily prevent ischemic renal failure but promotes a nonoliguric state that is easier to manage clinically.74–80 In addition, mannitol can ameliorate pulmonary injury associated with aortic surgery.81
Acher and coworkers use the partial opioid agonist naloxone for neuroprotection during TAA reconstruction, which they perform with the clamp-and-sew technique and routine ligation of all intercostal arteries. They reported excellent results, with paraplegia rates of 3 percent,56 although their experience has not been duplicated elsewhere.
A host of pharmacologic agents that interfere with the ischemia–reperfusion injury process have been tested for their ability to prevent spinal cord injury, and they are reviewed thoroughly by de Haan.82 There are no uniform recommendations for the use of any of these agents. At the author’s institution, it has been found that diazoxide, a mitochondrial potassium–adenosine triphosphate (ATP) channel opener, protects against ischemic spinal cord injury in a rabbit model.83 Further work has shown that this effect is achieved through decreased production of reactive oxygen species in the mitochondria (unpublished data). Current research in cardiology and neurology has focused on polyadenosine diphosphate (ADP) ribose polymerase (PARP), an enzyme that participates in multiple cellular pathways involved in DNA repair, apoptosis, and ischemic preconditioning. This area of investigation shows promise for improvement in outcomes with respect to partial or delayed neurologic deficits that result from a modest ischemic (and subsequent reperfusion) injury to the spinal cord.
The author’s protocol for surgical TAA repair begins 2 days before surgery with spinal angiography. A spinal drain is placed by the anesthesiologists the day before surgery so that if a bloody tap occurs during drain placement, there is time for the hemorrhage to resolve without postponing or canceling the operation. After anesthetic induction, the patient is intubated with a single-lumen endotracheal tube that then is exchanged for a double-lumen endotracheal tube to permit single-lung ventilation. Proper placement of the tube is confirmed bronchoscopically. A double-lumen endotracheal tube is important not only to collapse the left lung to facilitate exposure of the aorta in the left chest but also to prevent blood and secretions from depositing in the left lung. Bronchial blockers do not provide reliable isolation of the left lung throughout these long operations and are not recommended.
A Swan–Ganz pulmonary artery catheter and additional central venous lines are placed. A radial arterial catheter always should be placed in the right arm, since a catheter in the left radial artery becomes useless if is necessary to clamp the aortic arch between the left carotid artery and the left subclavian artery. A transesophageal echocardiogram probe is placed, and the patient is put in the right lateral decubitus position on a beanbag with the left arm padded on a Kraus retractor. The spinal drain is connected to a drainage apparatus with a pop-off valve set to 5 to 10 cm H2O. The hips are rotated obliquely to keep the right groin in the surgical field. The left chest is prepped to the axilla superiorly, the spine posteriorly, and the sternum anteriorly. The abdomen is prepped to the right of the midline. Both groins are prepped to provide surgical access to the femoral vessels. A cell-saver and a rapid infuser should be readily available in the operating room.
The incision is determined by the proximal extent of the aneurysm. In situations where the proximal clamp is placed on or just distal to the aortic arch, as in a type I or type II TAA or for chronic dissection arising just beyond the left subclavian artery, a standard posterolateral thoracotomy is done. The chest is entered in the fourth intercostal space to allow satisfactory exposure of the aortic arch. A type III TAA may be approached through a sixth or seventh interspace incision, whereas a type IV TAA may be approached through an eighth or ninth interspace incision. The incision is carried down onto the abdominal wall, across the oblique muscles lateral to the left rectus abdominus muscle. The retroperitoneal plane is entered deep to the transversus abdominus muscle and developed posteriorly to the psoas muscle under the diaphragm. It is easy to enter the peritoneal cavity inadvertently at this point, especially medially by the edge of the rectus abdominus muscle, where the peritoneum is thin and adherent. The costal margin is divided with heavy scissors, connecting the left chest cavity to the retroperitoneum, and the left internal mammary artery is ligated.
A single simple thoracotomy incision rarely provides enough exposure to repair an extensive TAA. The author’s preference is to perform a subperiosteal resection of the rib above or below the interspace that is entered, depending on where exposure is needed most. Additional exposure is provided by ‘notching’ an additional rib adjacent to the excised rib; a 2-cm segment is excised subperiosteally near the costospinal junction. This approach usually provides adequate exposure to repair even the most extensive TAA. Other surgeons recommend performing a double thoracotomy through one or two skin incisions or a stepwise thoracotomy, in which two or more ribs are divided vertically to extend the thoracotomy through more than one interspace.84 A self-retaining retractor is placed in the wound and is critical to maintaining exposure of the large field in these long cases: The Omni, Thompson, and Buchwalter retractors are examples of such retractors.
After the chest is entered, ventilation of the left lung is arrested to collapse the lung. The aneurysm, left lung, and heart are inspected. There can be extensive adhesions between the lung and the aneurysm. Filmy adhesions are taken down to mobilize the lung off the aneurysm. If the adhesions are dense, lysis of them can cause extensive intrathoracic and intrapulmonary hemorrhage, and it may be necessary to work around the lung and even open the aneurysm without mobilizing the lung off it. The diaphragm is divided to expose the entire thoracoabdominal aorta, joining the left pleural cavity and the retroperitoneum into a single operative field. This can be done in two different ways. A radial incision can be made from the anterior edge of the diaphragm where the costal margin is divided directly down to the aortic hiatus. This incision is simple to close but results in paralysis of the diaphragm lateral to the incision. Alternatively, the diaphragm can be taken down peripherally, leaving a 2-cm cuff along the chest wall all the way to the aortic hiatus. This is the preferred incision at the author’s institution because it preserves the function of the diaphragm and has a minimal effect on postoperative respiratory function, facilitating repair of TAAs in patients with even marginal respiratory status.
The patient then is placed on partial (left heart) bypass with a Bio-Medicus centripetal pump circuit. The inflow site for the cardiopulmonary bypass pump circuit can be one of three sites: the proximal descending thoracic aorta, the left atrium, or the left inferior pulmonary vein. Aortic cannulation can be hazardous, especially in a diseased aorta, and can lead to extensive bleeding while the cannula is in place or after it is removed. The author never cannulates the ascending aorta or the aortic arch because of difficulty with exposure and the risk of stroke. Atrial cannulation requires a pericardiotomy and manipulation of the heart. The preferred site at the author’s institution is the left inferior pulmonary vein, which can be accessed in an extrapericardial location. It is easy to locate after division of the inferior pulmonary ligament and mobilization of the lower lobe of the left lung, although occasionally it cannot be used because of adhesions in this area. The patient is anticoagulated systemically with 100 to 150 U/kg of intravenous heparin, and an activated clotting time (ACT) is checked. A pledgeted purse-string suture of 2-0 Ticron is placed in the left inferior pulmonary vein, and the vein is opened after the anesthesiologist delivers a Valsalva breath to prevent air embolism. An angled Sarns venous cannula (20F to 24F) is placed into the pulmonary vein through the purse-string suture and connected to the inflow limb of the pump circuit.
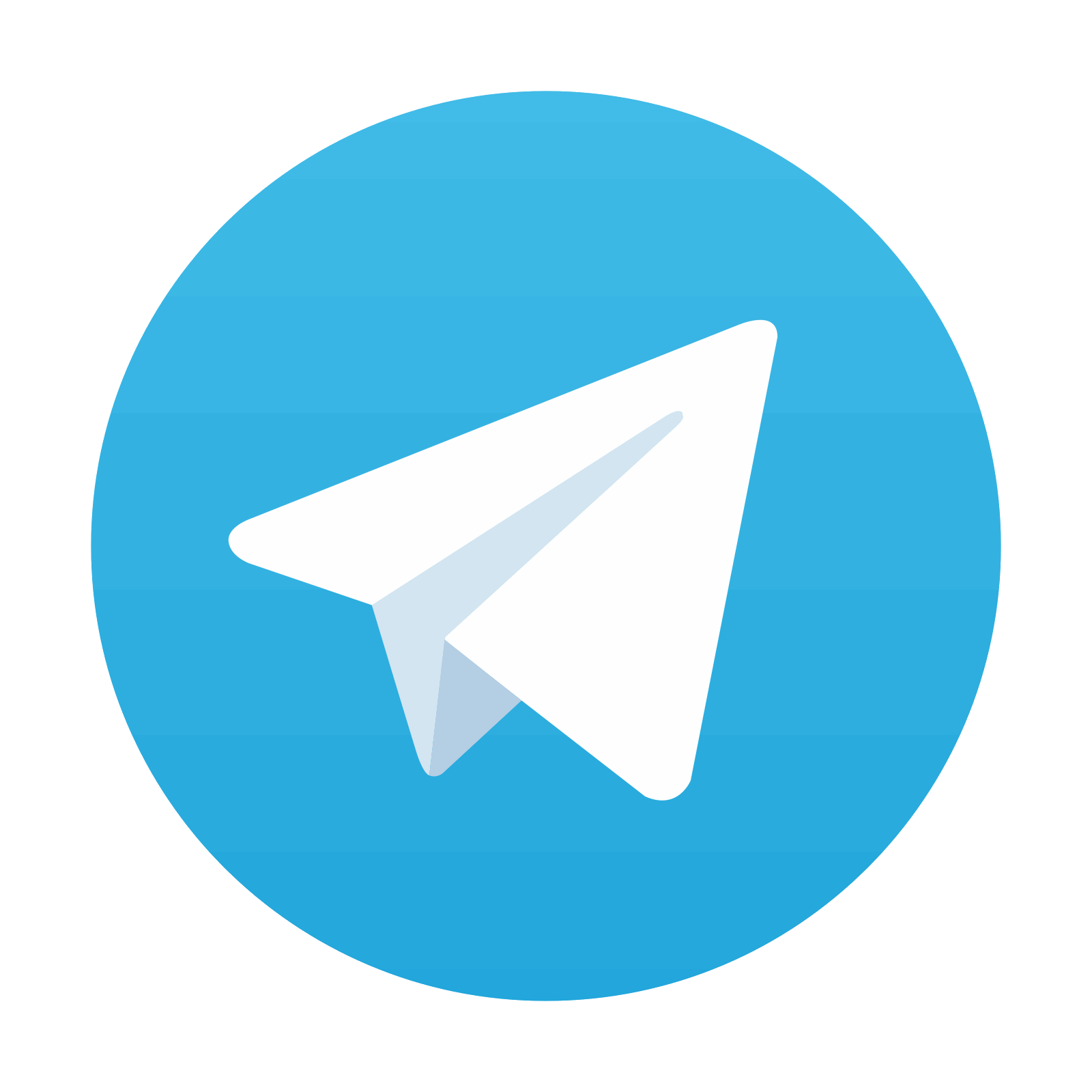
Stay updated, free articles. Join our Telegram channel

Full access? Get Clinical Tree
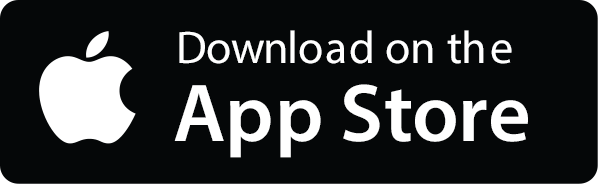
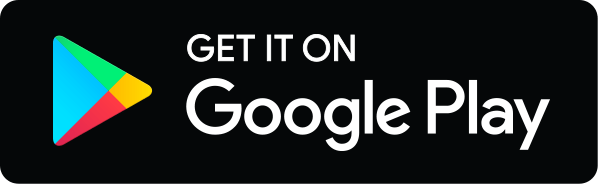