7. THE SMALL INTESTINE
After studying this chapter you should be able to:
1. Describe the structure of the small intestine and the major cell types present in the mucosa.
2. Understand the processes of water and electrolyte secretion and absorption in the small intestine.
3. Understand the mechanisms of diarrhoea, its consequences and treatment.
4. Describe the motility of the small intestine, and its control.
Introduction
In the human, most digestion and absorption occurs in the small intestine. Digestion in the stomach is dispensable and it is only preparatory. Pancreatic juice and bile from the liver enter the duodenum (Fig. 7.1). Intestinal juice is secreted along the entire length of the intestine from glands in the wall. In the normal individual, digestion is substantially complete when the chyme passes into the colon. The small intestine normally also absorbs over 95% of the water which enters the gastrointestinal tract. There is considerable reserve of function, and two-thirds of the small intestine can be removed without serious impairment of the quality of life.
Absorption is the central process of the digestive system and all other physiological processes that occur in the gastrointestinal tract subserve it. In this chapter we shall deal with the absorption of water and monovalent ions. Digestion and absorption of other nutrients will be dealt with separately. We shall also consider how the contractile activity of the intestines mixes and propels the food towards the ileum.
The importance of water and electrolyte absorption in the intestines is illustrated in this chapter, by the problems encountered in cholera, a condition in which there can be a massive loss of fluid from the body (Case 7.1: 1).
Case 7.1
An elderly man was carried by his son into a hospital, which was situated in a remote region of Bengal. The man appeared emaciated. He said he had initially been vomiting and suffering from abdominal distention. Now he was suffering from copious diarrhoea. The duty doctor noted that the man’s skin lacked turgor. The man’s pulse was barely detectable but his pulse rate was rapid (100b.p.m.). The younger man was also suffering from diarrhoea, but he was less severely affected. The doctor suspected that they were both victims of the latest cholera epidemic. Such epidemics are not uncommon in the region because of contamination of food and drinking water with the bacterium Vibrio cholerae. The elderly man was provided with electrolyte fluid via an intravenous drip. His plasma and urine K+ and HCO3− concentrations were monitored. He was also given intravenous tetracycline for 2 days. The younger man was given some packets containing a mixture of salt (NaCl) and sugar (glucose) and a supply of clean drinking water. He was told to dissolve the salt and sugar in clean water from the hospital supply and to drink large quantities of the solution over the next few days. He was given tetracycline to take by mouth. Both patients had recovered within a few days.
We shall address the following questions:
• What causes cholera?
• Why were the patients treated with tetracycline?
• Why was it necessary to monitor the patient’s plasma and urine K+ and HCO3− concentrations?
• How would the patients’ acid–base status have changed?
• What adjustments in respiratory and renal function would be taking place in response to the changes in acid–base status?
• How does the V. cholerae bacterium cause diarrhoea?
• Could other treatments counteract the effect of the toxin on the crypt cells?
• What is the rationale for treating people suffering from cholera with (a) oral fluid containing NaCl and glucose and (b) intravenous fluid? What is the likely composition of the intravenous fluid?
• Why was the elderly man’s pulse (a) feeble and (b) rapid?
• What adjustments in cardiovascular and renal function would take place in response to the hypovolaemia?
• Are changes in intestinal motility involved in this condition?
Intestinal phase of digestion
When chyme enters the small intestine from the stomach, it causes the release into the blood of the hormones secretin and CCK from endocrine (APUD) cells in the walls of the duodenum. Secretin stimulates secretion of alkaline pancreatic juice, alkaline bile, and alkaline intestinal juice. CCK stimulates secretion of enzyme-rich pancreatic juice. It also causes contraction of the gall bladder and relaxation of the sphincter of Oddi, which promotes the entry of bile and pancreatic juices into the duodenum (Fig. 7.1). At the same time, these hormones inhibit gastric emptying which enables processing of the contents of the small intestine to occur before the next portion of chyme enters from the stomach, and prevents the intestinal chyme from becoming too acid. The latter is important because the action of pancreatic enzymes and lipid absorption require an alkaline or neutral pH.
The chyme also stimulates contraction of the smooth muscle of the intestines, which mixes the intestinal contents and propels it towards the ileum. The intestinal phase of the control of digestion is summarized in Table 7.1.
Effect | Hormone | |
---|---|---|
Secretion | ||
Duodenal (alkaline) | Stimulation | Secretin |
Bile (alkaline) | Stimulation | Secretin |
Bile (hepatocyte) | None | |
Pancreatic juice (alkaline) | Stimulation | Secretin |
Pancreatic juice (enzyme-rich) | Stimulation | CCK |
Smooth muscle | ||
Stomach | Relaxation | CCK, secretin |
Gallbladder | Contraction | CCK |
Sphincter of Oddi | Relaxation | CCK |
Intestinal | Contraction | Various |
Anatomy and structure
In the adult human, the small intestine consists of approximately 6m of 3.5cm diameter tubing that is coiled in the abdomen. It leads from the stomach to the colon (Fig. 7.2). The duodenum comprises the first 25cm or so. This region differs from the rest of the small intestine in having no mesentery. The adjacent region is the jejunum that comprises approximately 40% (2.5m) of the small intestine. The remaining distal part is the ileum. The longitudinal smooth muscle in the wall is normally partially contracted (tone). After death, when it is relaxed, the small bowel reaches a length of approximately 7.5m.
Duodenum
The duodenum has an essential role in mixing digestive juices, derived from the liver and pancreas, and its own wall with the food. It forms an arc ending in a sharp bend, the duodeno-jejunal flexure. The head of the pancreas lies within the arc, with which it shares a blood supply via the pancreatico-duodenal artery (Fig. 7.2). At approximately two-thirds of the way down the descending part of the duodenum are two papillae. The major duodenal papilla is the location of the duct where the bile and pancreatic juice empty into the duodenum via the ampulla of Vater. The opening of the ampulla is controlled by the sphincter of Oddi (Fig. 7.1). An accessory pancreatic duct, present in most individuals, opens at the tip of the lesser papilla.
The surface of the duodenum is folded. The folds are known as plicae circularis (circular folds). Most are crescent-shaped, and do not disappear when the intestine is distended. The mucosa of the small intestine is covered with tiny projections, known as villi. These are tongue-shaped in the duodenum.
Two types of glands are present in the duodenal mucosa. At the base of the villi are tubular invaginations that reach almost to the muscularis mucosae, known as intestinal glands or crypts of Lieberkühn (Fig. 7.3). The submucosa of the duodenum contains coiled compound tubular mucous glands known as glands of Brunner that secrete an alkaline fluid rich in mucus. These glands are more numerous in the proximal region of the duodenum. They usually open at the base of the intestinal glands.
Jejunum and ileum
No anatomical feature separates the jejunum from the ileum. The structure of the jejunum and ileum is basically similar to that of the duodenum. However, there is a gradual decrease in diameter, and in the thickness of the wall, and in the number of mucosal folds, with distance from the duodenum (Fig. 7.4). The folds are absent altogether from the terminal ileum. In addition the villi gradually become less numerous, smaller, and more finger-like, with distance from the duodenum. Numerous lymph nodes, called Peyer’s patches are present in the mucosa and submucosa of the ileum. The junction between the ileum and the large intestine is the ileocaecal junction. It consists of a ring of thickened smooth muscle, known as the ileocaecal sphincter (see Ch. 10), which reduces reflux from the colon.
The jejunum and ileum are on a mesentery. This contains the arterial blood vessels (branches of the superior mesenteric artery), and the veno-lymphatic drainage vessels, which are supported in fatty connective tissue, and is covered by mesothelium.
Blood supply
At rest, approximately 10% of the cardiac output flows to the intestine. The blood vessels in the jejunum and ileum are derived from the superior mesenteric artery. Numerous arterial branches form an extensive network in the submucosa that supplies the wall of the intestine (Fig. 7.5).
Nerves, hormones and local paracrine factors control the intestinal circulation. Stimulation of the sympathetic nerves (which follow the arteries) causes vasoconstriction and reduced blood flow, enabling a redistribution of blood away from the intestine. This is particularly important during low cardiac output states such as shock, or during exercise when extra blood is required for skeletal muscle. In the blood vessels of the villi, the vasoconstriction is relatively short-lived. This is due to vasodilator metabolites, such as adenosine, which accumulate during the vasoconstrictor response.
The splanchnic blood flow increases by 50–300% during a meal (functional hyperaemia). Distension of the walls of the intestine and substances present in the chyme stimulate the blood flow. Other stimuli include products of carbohydrate and lipid digestion in the proximal small intestine, and bile acids in the distal ileum. Gastrin, CCK, secretin, serotonin and histamine all have the capacity to increase blood flow, and may be involved in this response during a meal.
The nutrients absorbed across the absorptive cells are transported to the liver in the portal veins (Ch. 6).
Countercurrent exchange in the villi
The blood vessels of the villi (Fig. 7.6) constitute a countercurrent exchange system, whereby there is net diffusion of dissolved substances across the interstitium from the venule to the arteriole, or vice versa, depending on which limb has the higher concentration. Thus oxygen tension is higher in the ascending arterial blood because it is extracted from the blood in the capillaries, and it diffuses from the ascending to the descending limb. This results in a lower oxygen tension at the tips of the villi than at their bases. The relative hypoxia at the tip has been causally implicated in the shedding of the epithelial cells from the tips of the villi. In hypovolaemic shock, a situation where hypotension is present, the reduced perfusion pressure together with increased smooth muscle relaxation that accompanies it, leads to a reduced oxygen supply. This can cause the tips of the villi to become severely hypoxic. During hypovolaemic shock this can lead to ulceration of the intestines, which can develop within hours of the onset of hypovolaemia. Such acute ulcers are seen in patients who have suffered severe burns, especially children, and after major haemorrhage, such as a leaking aortic aneurysm.
![]() |
Fig. 7.6 |
Structure of the intestinal wall
The wall of the small intestine has the same basic structure as other regions of the gastrointestinal tract (see Ch. 1). Beneath the serosa is the muscularis externa that consists of two layers of smooth muscle, an outer longitudinal coat and an inner circular coat. Preganglionic parasympathetic nerve fibres of the vagus nerve synapse with the cells of the terminal ganglia in the myenteric plexus. The postganglionic nerves stimulate muscle contraction and gland secretion (see Ch. 1). Postganglionic sympathetic nerve fibres arising largely from the prevertebral ganglia mostly innervate their target effector cells directly. The submucosal plexus contains a few parasympathetic ganglia of the vagal nerve, but postganglionic sympathetic fibres from the superior mesenteric plexus form the major proportion of the extrinsic nerves present.
Beneath the submucosa is the muscularis mucosae which consist of two thin layers of muscle with some elastic tissue. The inner muscle layer consists of circularly disposed fibres and the outer layer longitudinally disposed fibres. The muscularis mucosae permit localized movement of the mucous membrane. Small bundles of muscle fibres extend from it to the epithelium. Some of the fibres end on the epithelial basement membrane. Beneath the muscularis mucosa in the lamina propria is a layer of connective tissue that supports the epithelium and contains collagen, reticular fibres and some elastic fibres. It also contains blood capillaries and lymph capillaries that are situated close to the epithelial surface, especially in the villi (Fig. 7.6). It also contains numerous lymphatic nodules. Lymphocytes and plasma cells gain access to this layer across the epithelial membrane. These cells protect the tissue against bacteria that enter across the epithelial membrane. The plasma cells produce IgA immunoglobulins. The innermost layer is the epithelium which is described below.
The villus
The villus is regarded as the unit of absorption. Its length varies between 0.5 and 1.5mm, depending on its location in the small intestine. The structure of the villus is depicted in Figure 7.6. Each villus contains a blood capillary network and a blind-ended lacteal (or lymph vessel). It is covered by simple columnar epithelium. Most of these cells have numerous cytoplasmic extensions, known as microvilli at the luminal surface. The microvillous surface of the intestine is known as the brush border.
Histology
The mucosa of the small intestine is simple columnar epithelium. Four cell types are present:
1. Absorptive cells that produce digestive enzymes and absorb nutrients from the chyme
2. Goblet cells that produce mucus which lubricates the surface and protects it from mechanical damage
4. APUD cells that produce peptide hormones which regulate secretion and motility in the gastrointestinal tract, liver and pancreas. The general function and structure of APUD cells are described in Chapter 1.
The four cell types arise from undifferentiated cells in the crypts of Lieberkühn. The granular and endocrine cells remain at the bottom of the crypts but the absorptive and goblet cells slowly migrate up the sides of the villi, to the tips. Figure 7.7 shows the cell types and their typical locations in the mucosa. The cells that migrate are eventually shed from the tips. The process of migration from the crypts to the tips of the villi occurs over 3–6 days in the human. Thus, most of the intestinal epithelium is renewed every few days. The columnar cells mature as they travel towards the tips of the villi, and their functions change. There is a gradual transition from base columnar cells in the crypts (Fig. 7.7) to villous columnar cells; their size increases progressively as they ascend the walls of the crypts and villi, and their content of free ribosomes decreases, while their content of rough endoplasmic reticulum increases.
Cell types
Villous epithelium
About 90% of the cells covering the villi are the absorptive columnar cylindrical cells. Most of the rest are goblet cells, but ≤0.5% are endocrine cells. The absorptive cells have abundant cytoplasm and mitochondria but few ribosomes. They have a thick striated microvillous border and convoluted lateral cell membranes. Figure 7.7 shows the structure of an absorptive cell, and the structure of microvilli. The rough endoplasmic reticulum and Golgi saccules are well-developed in columnar cells at the base of the villi but less prominent in cells at the tips of the villi. The columnar cells produce a cell coat that is composed of glycoproteins, but the cells at the base of the villi are more active in this respect than the other cells, as is consistent with their well-developed Golgi saccules. Some of the glycoproteins present are enzymes involved in the digestion of nutrients such as disaccharides (see Ch. 8). They act in situ but are also active after being shed into the lumen.
Crypt epithelium
The base of the crypt contains approximately equal numbers of small columnar cells and Paneth cells. The small columnar cells have sparse cytoplasm containing few mitochondria and little rough endoplasmic reticulum and a small Golgi apparatus. However, they contain numerous free ribosomes, consistent with their active protein synthesizing function. They have smooth lateral membranes and are relatively undifferentiated. These are the stem cells that give rise to the other cell types.
Paneth cells are highly differentiated, possessing abundant rough endoplasmic reticulum and a prominent Golgi apparatus. They synthesize enzymes, such as lysozyme, and sequester them in zymogen granules, from which they are released into the lumen by exocytosis.
There are also oligomucous cells that contain few mucus globules in the crypts. These are the precursors of the goblet cells. They can divide but they lose this ability when they become distended with mucus after migrating up the villus.
Endocrine cells comprise about 1% of the cells in the crypts. They have a narrow apex, and a wide basal region that is packed with dense argentaffin granules. These cells produce hormones such as secretin, CCK, somatostatin or endorphins. Others produce serotonin.
There are a few caveolated cells, characterized by invaginations of the cell membrane extending into its cytoplasm (caveolae). They have long microvilli that contain long bundles of straight filaments that extend into the cytoplasm, and filaments encircling the apical region. Their role is unknown.
Intestinal secretions
An alkaline fluid containing electrolytes, mucus and water, is secreted throughout the length of the small intestine. The precise composition of the secretion, and the mechanisms that control it, vary from one region to another. It is secreted by the immature cells in the crypts of Lieberkühn. The mechanisms involved are outlined in Figure 7.8. The key step is the active transport of Cl− across the basolateral membrane of the cell. Cl− is actively transported into the cell via a co-transporter protein that transports Na+, K+ and 2Cl−. The transport of Na+ down its electrical gradient is the driving force for the operation of this transporter. The K+ is transported back out via K+ channels in the same membrane. This K+ flux maintains the electrical potential difference (cytosol negative) across the cell membranes. This potential difference contributes to the driving force for the basolateral influx of Na+ across the basolateral membrane, and also for Cl− transport across the luminal membrane. Cl− is transported into the lumen via the cystic fibrosis transport regulator (CFTR, see Ch. 5) which is an electrogenic Cl− channel in the luminal membrane. Na+ is then transported into the lumen between the cells, down the electrochemical gradient produced by the extrusion of Cl− from the cell. The opening time of the CFTR channel is prolonged by increased cAMP. Therefore secretion by the crypt cells is stimulated by substances that elevate cAMP, such as vasoactive intestinal peptide (VIP) and prostaglandins. Ca2+-mobilizing agents such as acetylcholine potentiate the actions of these substances. The mechanism of action of cholera toxin is also via increasing intracellular cAMP. In this case it causes a massive secretion of fluid (Case 7.1: 2). There is a defect in the CFTR channel in cystic fibrosis (see Ch. 5). The first manifestation of cystic fibrosis can be postnatal constipation (meconium ileus) that is directly related to absence of the CFTR channel.
![]() |
Fig. 7.8 |
Case 7.1
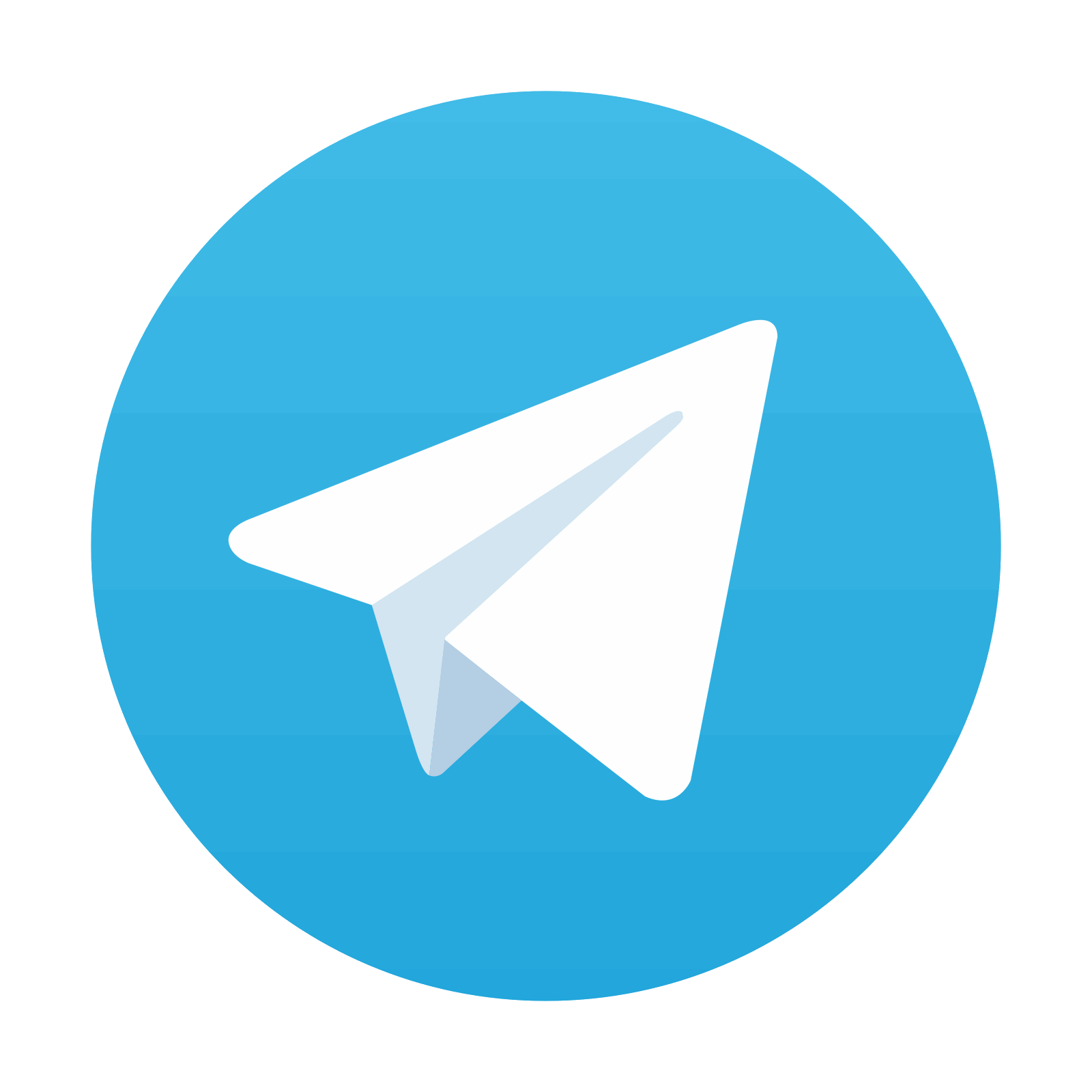
Get Clinical Tree app for offline access
Causes, mechanism of action and changes in electrolyte and acid–base balance
Causes
The V. cholerae bacterium present in contaminated water and food produces a toxin that elicits a massive secretion of fluid and electrolytes. The effect is produced mainly in the proximal small intestine. Epidemics in the Indian subcontinent usually occur in the early summer before the monsoon breaks. The bacteria are harboured in the gall bladder in asymptomatic carriers, which may comprise up to 5% of the population in this region.
Treatments with antibiotics such as tetracycline or chloramphenicol is effective but should be regarded as ancillary to rehydration therapy (see below). Thus, for example, tetracycline treatment decreases the average duration of the disease by 60%.
Anticholera vaccines have been developed, but these are of limited use during epidemics because it takes 2–3 weeks for them to become effective.
Bacteria are normally destroyed by gastric acid in the stomach, normally providing some protection. Increased susceptibility to the disease is seen in individuals with achlorhydria or those who have had a partial gastrectomy.
Mechanisms of cholera toxin action
Hypersecretion
There is an increased secretion of Cl−, Na+ and water into the crypts of Lieberkühn in patients with cholera. The cholera toxin is an 84 000kDa protein produced by V. cholerae. It binds to monosialoganglioside (Gm1) receptor molecules in the brush border membrane of the crypt cells, mainly in the proximal intestine. This results in activation of adenyl cyclase, which causes an increase in cAMP in the cells. The increase in cAMP causes activation of the CFTR Cl− channels in the brush border resulting in an increase in the secretion of Cl−. Na+ and water secretion increase as a consequence. Cholera victims sometimes produce up to 20L of watery stool per day. Treatment with somatostatin analogues such as octreotide which inhibit secretion by decreasing the levels of cAMP counteracts the effect of cholera toxin.
The intramural nerves are also involved in the mechanisms of the toxin action because substances that block nerve activity reduce the effects of the toxin. However, the precise neural mechanisms involved are unclear.
Hypermotility
Hypermotility of the small intestine can result in water and electrolytes being delivered rapidly into the colon. It can be delivered so fast that the colon may not be able to absorb it before it is lost in the faeces. This mechanism is also involved in conditions such as cholera, as distension of the intestines by the large volumes of secreted fluids stimulates peristaltic activity, which propels the fluid rapidly along the intestines.
Changes in electrolyte and acid–base balance
Secretions of the small intestines contain large amounts of HCO3−, Na+ and Cl− ions. K+ is absorbed during a meal, down the concentration gradient set up by the absorption of water (see below). However, when the concentration of K+ in the lumen is reduced below approximately 25mM the concentration gradient favours net secretion into the lumen. This occurs via the paracellular pathway. In diarrhoea, the luminal contents become diluted with respect to K+ and it is therefore transported into the lumen. Considerable losses of K+ can occur, leading to hypokalaemia.
As HCO3− is secreted into the lumen, H+ is transported into the blood, which becomes transiently acid. Excessive loss of HCO3− in secreted intestinal fluid causes a severe acidosis. The transient acidity in the blood is normally neutralized by the alkaline tide that accompanies the secretion of acid in the stomach (see Ch. 3). If there is excessive loss of alkaline fluid from the gastrointestinal tract however, the acidity in the blood is proportionately increased and it cannot be buffered. This metabolic acidosis will be partially compensated in the short term by an increased rate and depth of breathing which results in CO2 being blown off from the body. Longer-term adjustments are brought about by reabsorption of HCO3− in the tubules of the kidney, and excretion of H+ (see the companion volumes: The Respiratory System and The Renal System).
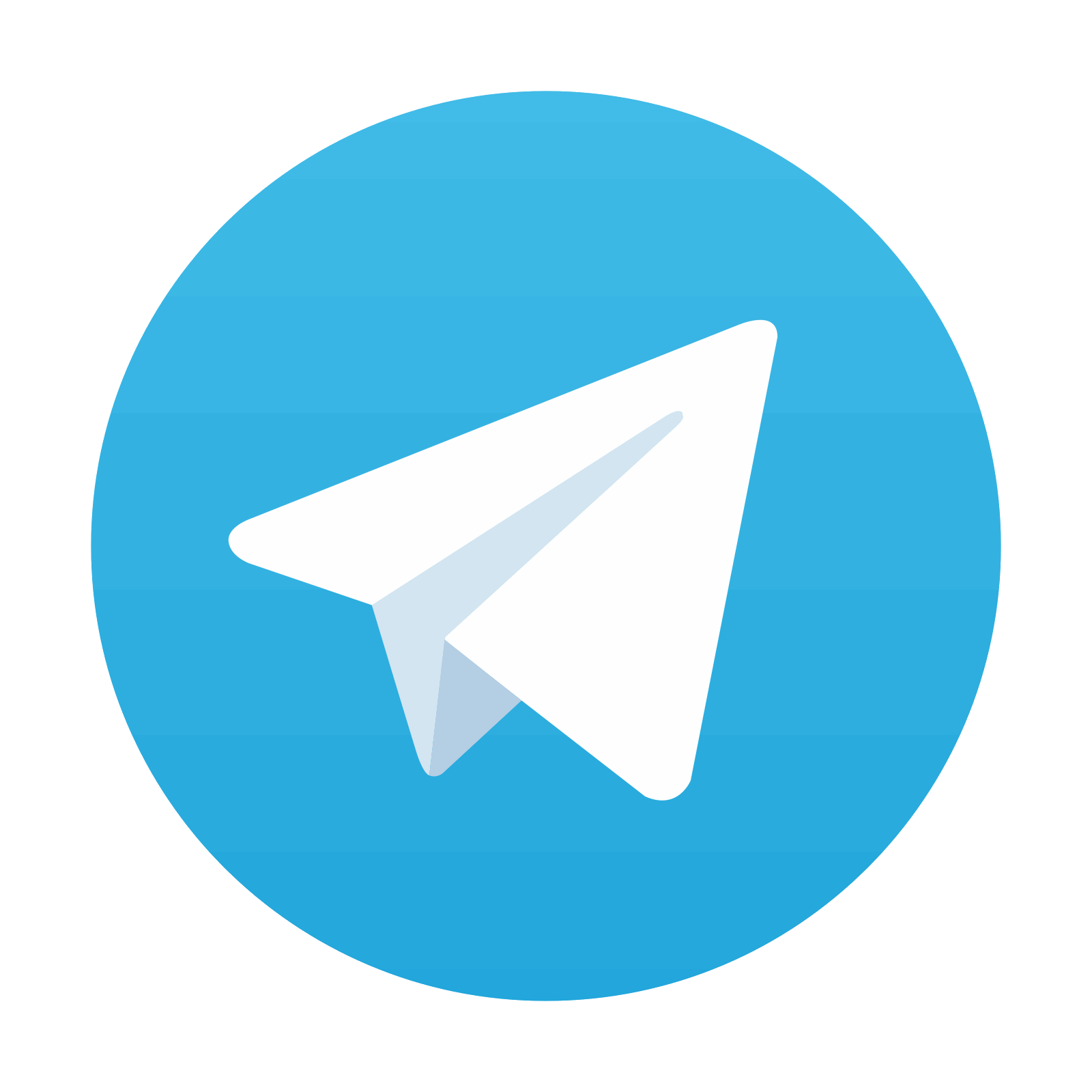
Stay updated, free articles. Join our Telegram channel

Full access? Get Clinical Tree
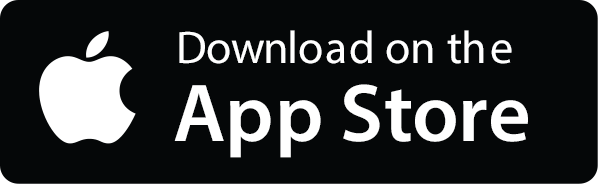
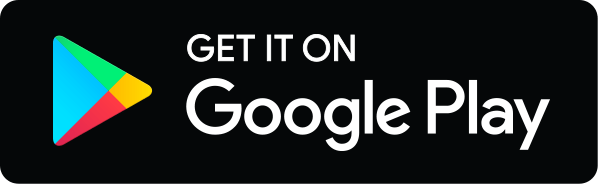
