© Springer Science+Business Media LLC 2018
Robert Provenzano, Edgar V. Lerma and Lynda Szczech (eds.)Management of Anemiahttps://doi.org/10.1007/978-1-4939-7360-6_1010. The Complex Relationships Between Iron Regulation, Obesity, and Anemia
(1)
Department of Medicine, Baylor College of Medicine, 6624 Fannin, Suite 1400, Houston, TX 77030, USA
Keywords
ObesityIronAnemiaLeptinAdiponectinHepcidinCytokinesMacrophageIs Anemia Associated with Obesity in the Normal Population?
Obesity has become more common in the past several decades, and has had considerable influence upon health from both a quality of life and an economic perspective. The World Health Organization estimates that there are more than 1.9 billion adults who were overweight, of which over 600 million were obese. It was also estimated that 41 million children under the age of 5 years old are overweight or obese. By 2025, it is estimated that obesity will affect 18% of men and 21% of women worldwide [1]. It is a public health problem, associated with diabetes and cardiovascular disease. With its attendant comorbidities, it is a number one health concern.
Iron is essential for normal cellular function, but in excess can be toxic. Iron-deficiency anemia is the most common form of anemia worldwide, and affects 1.6 billion persons [2]. Obesity is related to the clinical picture of iron deficiency, but this may relate to inflammation rather than nutrition. This chapter will look specifically at obesity from the perspective of its relationship to inflammation and iron regulation.
In observational studies looking closely at the association between anemia and obesity, a clear relationship has yet to be identified. In the third National Health and Nutrition Examination Survey (NHANES) BMI was stratified into normal (<25 kg/m2 n = 6059), overweight (25 to <30 kg/m2 n = 5108), mildly obese (30 to <35 kg/m2 n = 2366), moderate (35 to <40 kg/m2 n = 850), and severe (≥40 kg/m2 n = 465). When data were adjusted for age, gender, menstrual periods, race, ethnicity, alcohol use, education, smoking, and dietary iron ingestion, those in the higher weight group did not have a lower hemoglobin. However, the iron stores were lower in the severely obese vs. the normal group (odds ratio (OR) 2.4; 95% CI 1.5–3.7) and the odds ratio for inflammation as measured by the C-reactive protein was higher (OR 10.5; 95% CI 6.6–16.6) [3].
The relationships between obesity and anemia are not direct, nor straightforward. In an observational study from Taiwan, the risk for iron-deficiency anemia was compared with dietary intake in 1274 females 19 years of age and older between the years 2005 and 2008. In the normal BMI group vs. the group of 24% who were overweight vs. the group of 25.3% who were obese, the incidence of anemia was 19.5, 8.6, and 6.2% respectively. Anemia was observed to be less in the group presenting with the highest BMI. What was noticed, however, was that the risk of iron-deficiency anemia rose in obese persons eating a higher fat and lower carbohydrate diet (OR 10.119; 95% CI 1.267–80.79) [4]. The relationship between iron status and obesity has been extensively reviewed in 15 studies dating from 1962 through 2011, and confirm that regardless of iron supplementation or other dietary factors, obesity is associated with iron-deficiency markers. Furthermore, iron absorption has been shown to be affected by obesity [5].
Paradoxically, in a Colombia nutritional survey , obesity was associated with a lower incidence of anemia. The survey investigated 3267 females between the ages 13 and 49. The group was divided into adolescent women, ages 13–17 and adult women, ages 18–49. Patients who were pregnant, who exhibited a high C-reactive protein level (>0.12 g/L) or who presented with a low plasma ferritin level (plasma ferritin <12.0 μg/L) were excluded. The anemia was altitude-adjusted. 29.2% of subjects were overweight and 13.1% were obese. 16.1% of the women had iron deficiency and 32.5% were anemic. Both being overweight or being obese were associated with a 20% lower incidence of being anemic than having a normal weight (P < 0.05). BMI was not significantly associated with iron deficiency, yet iron deficiency was present in 6.3% of women and anemia present in 12.8% of women who were overweight or obese. Iron deficiency (13.5%) and anemia (21%) were more prevalent in the older population of women [6].
Despite the lack of a clear relationship, it becomes apparent after reviewing these clinical nutrition survey findings that there is an association between iron deficiency and obesity that may be exacerbated by age or a high-fat diet. The next step is to determine what the proposed mechanisms can be and what lessons we can learn from a clinical perspective.
Proposed Mechanisms Linking Iron Regulation with Obesity
Iron transport and its relationship to anemia have been reviewed elsewhere in this book. This discussion will therefore be limited to the relationship between iron transport and obesity. First, we must have a clear understanding of what obesity is, and how it impacts the body with respect to inflammation.
Body Mass Index (BMI)
We can define obesity as an energy imbalance, and for purposes of this chapter base it upon the body mass index, a representation of the body weight or mass adjusted for height. BMI is measured as the ratio of kilograms per meter squared (kg/m2). The calculation of ones BMI is relatively simple and several tools are available on the web. Here is one that was programmed by this author (http://touchcalc.com/bmi). A BMI of greater than 25 kg/m2 is considered overweight, and if over 30 kg/m2 obese. The concern lies in strong observational data that links an elevated BMI to both cardiovascular disease and type 2 diabetes, but what is even more concerning comes from long-term follow-up studies of adolescents with high normal BMI ranges. An Israeli study demonstrated those in the high normal 50th–74th BMI percentiles had had a graded risk for death. The hazard ratio (HR) for persons in the 95th BMI percentile when compared with those in the 5th–24th percentiles was 4.1 (95% CI 3.1–5.4) for death from coronary artery disease during a follow-up period of 30–40 years [7]. There are two forms of obesity, truncal and visceral. In truncal obesity, adipocytes store free fatty acids and become engorged. In visceral obesity, however the fatty acids are first accumulated by the liver and then stored in the abdominal area. Visceral obesity has a higher risk of causing cardiovascular disease.
Obesity and Inflammation
Inflammation , in its broadest sense, is a mechanism the body uses to respond to an “attack” and to initiate repair, particularly from what it considers a foreign body such as a bacteria or a virus. Inflammation can also be triggered by obesity, and in this circumstance, is a low-grade insidious process. The inflammatory process associated with obesity is widespread, affecting the liver, pancreas, heart, brain, and muscle, in addition to adipocytes. In many disease states, the insidious inflammatory response leads to further harm in these tissues. The story of obesity and inflammation starts with the adipocyte (fat cell), which in addition for being a repository for free fatty acids, shares in the control of several metabolic processes, playing roles in the regulation of inflammatory mediators, iron, the appetite, lipids, and sugar. Overexpression of inflammatory and macrophage genes occurs when adipocytes are exposed to obesity and undergo a remodeling process. The presence of obesity recruits and then stimulates the macrophages interspersed with the adipocytes to release of inflammatory cytokines. The fat cell also releases adipokines that play key roles in an autocrine, paracrine, and endocrine fashion. The two most studied adipokines are adiponectin and leptin.
Adiponectin
Adiponectin is a 224-amino-acid collagen-like protein that is related to complement factor C1q and its C-terminal domain is similar to tumor necrosis factor alpha (TNF-α). It is often referred to in the literature as Acrp30. It is secreted from adipocytes, particularly in anorexia, and circulates in plasma, increases the oxidation of free fatty acids (FFA) in many tissues, especially skeletal muscle where it interacts via adiponectin receptor 1 and the liver where it interacts through adiponectin receptor 2. It leads to accumulation of free fatty acids inside the adipocyte, and clears free fatty acids from the circulation. Fat oxidation is mediated by adiponectin activation of AMP-activated protein kinase. This enzyme increases glucose transport into muscle and can be activated by exercise. It improves insulin resistance through the PPAR-α (peroxisome proliferator-activated receptor alpha) pathway. Exercise training may increase adiponectin receptors [8, 9].
Adiponectin increases insulin sensitivity and low adiponectin levels are associated with obesity, type 2 diabetes, and decreased FFA entering the subcutaneous adipocyte. The metabolic disease occurs as excess FFAs are deposited in other locations. Adiponectin levels fall in truncal obesity, and are also low in association with visceral obesity. As the levels fall, insulin resistance increases as do markers of inflammation. Lower adiponectin levels have been implicated in the relationship between macrophages and atherosclerosis. Thus, lowered levels of adiponectin are associated with cardiovascular disease and myocardial infarction [8]. Low adiponectin levels are correlated with elevated C-reactive protein, IL-6, the production of TNF-α and the inhibition of Nuclear factor-κB (NF-κB) signaling. High levels of adiponectin promote IL-10 which blocks inflammation.
The pathogenesis of this relationship is just becoming clearer and a strong relation between adiponectin and metabolic disorders such as type 2 diabetes with macrophage regulation and chronic low-grade inflammation are apparent. Monocytes from the bone marrow are recruited to adipose tissue by adipokines and differentiate into macrophages. There are two phenotypes of macrophages , M1 and M2, and their respond to different cytokines can change their phenotype. M1 macrophages are anti-inflammatory, while M2 type are proinflammatory. Macrophages infiltrate into adipose tissue and when polarized into a proinflammatory M2 phenotype reduce adiponectin receptor 1 and 2 expression, but when proinflammatory stimuli are altered and the M1 phenotype expressed, the adiponectin receptor is upregulated [10].
Adiponectin and Iron
The presence of iron inside the adipocyte decreases adiponectin production. In type 2 diabetes, a low adiponectin state, adiponectin is inversely related to serum ferritin. An increase in iron inside the adipocyte is associated with decreased adiponectin mRNA and regulates adiponectin transcription.
Like the macrophage and the enterocyte, adipocytes have an iron exporter, ferroportin. Ferroportin is responsible for shuttling iron out of the cell. When ferroportin decreases, cells such as adipocytes, macrophages, and enterocytes load with iron. In the adipocyte, this iron loading has been demonstrated to decrease adiponectin [11]. The amount of iron in the adipocyte also regulates leptin and food intake.
Leptin
Leptin is a member of helical cytokines, and its membrane receptors are structurally like the receptors on cytokines. IL-6 and leptin receptors have some subunits in common. It was the first adipokine discovered and has a direct effect on the hypothalamus to reduce appetite and control food intake. In obesity, leptin concentrations increase, but target cells become resistant to its action [8].
Leptin and Iron
Increasing dietary iron intake enhances the appetite and lowers serum leptin levels. This has been shown independent of inflammation and was demonstrated in a study of 75 subjects who had serum leptin levels that were inversely associated with serum ferritin (Pearson’s r = −0.396, P = 0.0004). Ferritin is an intercellular protein capable of storing and binding iron. Small amounts secreted serum function as an iron carrier.
Dietary iron overload increased iron accumulation in adipocytes by 115% in mice, and in turn decreased leptin mRNA. Serum leptin levels decreased with iron loading (Pearson’s r = −0.26, P = 0.001). Iron increased phosphorylation of the cAMP-Responsive Element Binding Protein (CREB) and decreased activity in leptin promoter region. Through this dual action, feeding effects on metabolism were exhibited; iron influenced mice eating behavior. After a 9–12-week period, mice fed a high iron chow ate more during each 15-min feeding interval over a 3-day period when studied in a metabolic chamber (P < 0.0001) [12].
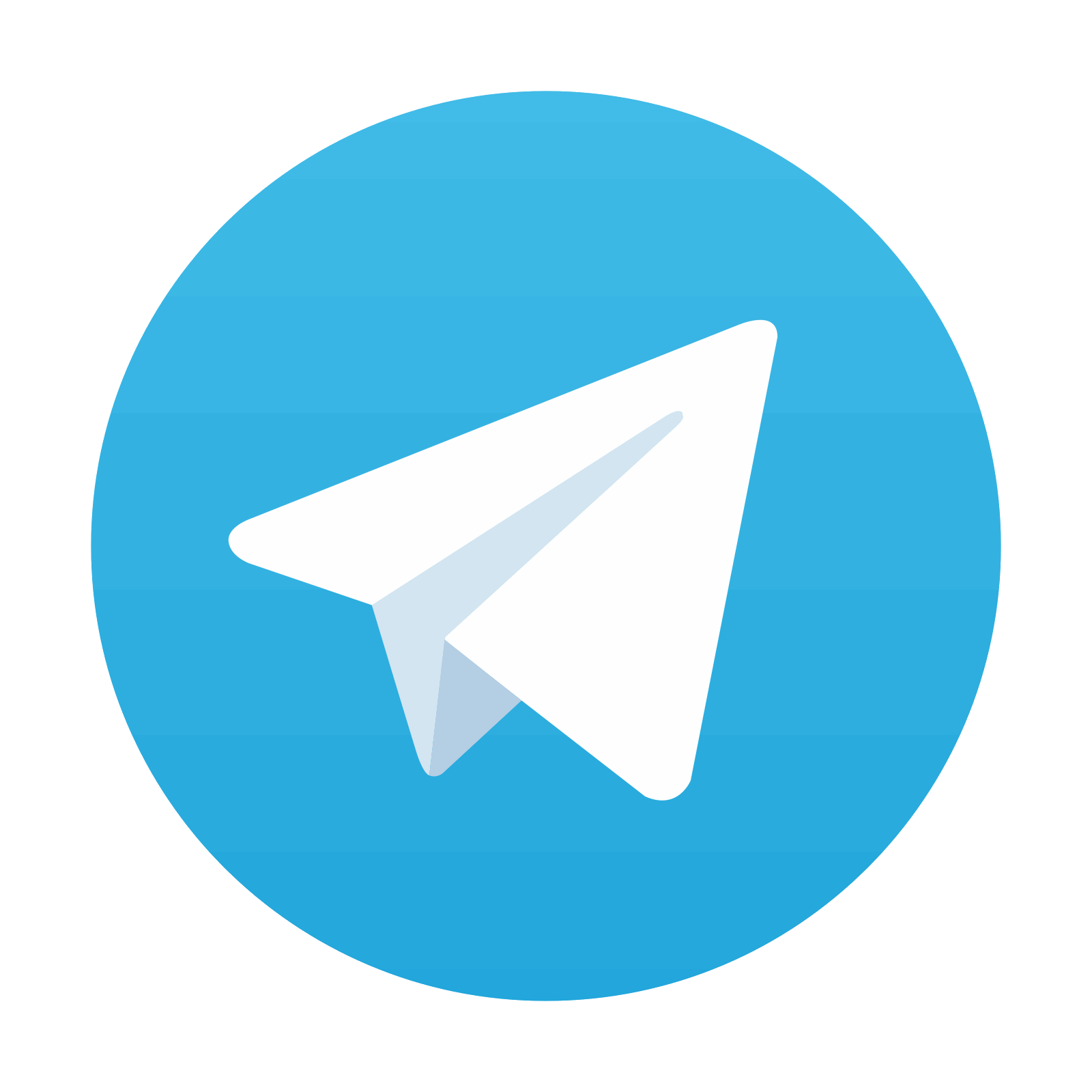
Stay updated, free articles. Join our Telegram channel

Full access? Get Clinical Tree
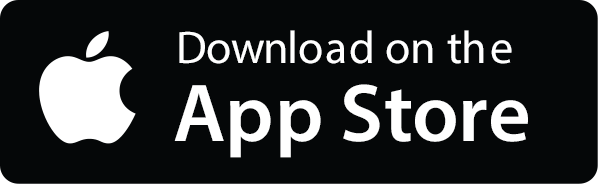
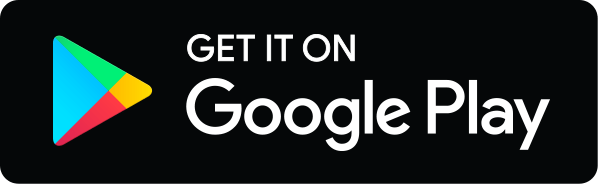