Robert M. Smith
A marked increase in the permeability of the alveolar–capillary membrane to water, solutes, and plasma proteins is the defining characteristic of the acute respiratory distress syndrome (ARDS); clinically, it is associated with diffuse lung infiltrates, respiratory distress, and respiratory failure. A prospective study using the 1994 American-European Consensus Conference (AECC) definitions of ARDS and acute lung injury (ALI) suggested that the age-adjusted incidence was 86.2 per 100,000 person-years. The incidence increased with age, reaching 306 per 100,000 person-years for those aged 75 to 84 years. On the basis of these statistics, it is estimated that more than 190,000 cases occur annually in the Unites States and that these cases are associated with 74,500 deaths. Although there is some suggestion that the incidence has decreased somewhat over time, ARDS remains a frequent and dreaded problem in modern intensive care units.
ETIOLOGY
Events that have preceded and appear to act as precipitants to ARDS are remarkably diverse (Table 67-1), although sepsis and trauma are the most common associated clinical settings. The wisdom of grouping patients with such disparate precipitating factors together under the umbrella of ARDS has been debated frequently. However, because the biochemical and cellular pathways that lead to ARDS are not understood and because patients have a number of apparent physiologic and histologic features in common, it has proven useful to consider them together. The probability of developing ARDS increases with multiple risk factors, but it is not known whether different precipitants act independently or via some common pathway that leads to lung injury.
Clinical Settings Associated with the Development of ARDS |
Clinical Processes Where ARDS Occurs in More Than 1% of Patients at Risk |
Aspiration of gastric contents |
Pneumonia requiring intensive care unit |
Severe sepsis |
Multiple trauma |
Disseminated intravascular coagulation (usually associated with other events) |
Other Clinical Processes Associated with ARDS |
Near drowning |
Smoke inhalation |
Inhalation of irritant or toxic gases |
Fat or air embolism |
Pancreatitis |
Hypertransfusion |
Thermal burn |
Cardiopulmonary bypass |
Narcotic administration |
The processes responsible for tissue injury in ARDS remain obscure. The lung is diffusely inflamed, and inflammatory cells (particularly neutrophils and their granular products) have been implicated in the pathogenesis of ARDS in animal models. Similarly, the production of highly reactive oxygen radical species by neutrophils or by resident lung macrophages may contribute to ARDS either by direct tissue injury or by modification of proteins, lipids, or DNA leading to inactivation (e.g., α1-proteinase inhibitor) or abnormal function. A number of factors may lead to neutrophil accumulation in the lung. Cytokines, elaborated systemically or locally in the lung following sepsis or trauma, may act as chemotactic factors (e.g., interleukin [IL]-8) or can cause up-regulation of endothelial and leukocyte adhesion molecules (e.g., IL-1). Preliminary studies suggested that elevated levels of IL-8 in alveolar lavage fluid of patients at risk for ARDS may predict the subsequent development of the full-blown syndrome. However, this finding has not been confirmed in larger studies, and the complex interplay between the various cytokines is not yet well understood. The occurrence of ARDS in severely neutropenic patients and the lack of neutrophil participation in some animal models of ARDS suggest that neutrophil-independent mechanisms of tissue injury also are important. Loss of surfactant activity in lung lavage specimens is seen early in ARDS and is a potential explanation for many of the physiologic abnormalities. The loss of surfactant activity is caused in part by alterations in surfactant production by type II pneumocytes and in part by inhibition of surfactant activity by the ingress of plasma proteins.
Over the last two decades it has become increasingly clear that the ways in which mechanical ventilatory support is applied may influence the progress and potentially the development of ARDS. In animal models, positive-pressure ventilation alone can initiate diffuse lung injury with radiographic and histologic features indistinguishable from ARDS. This injury occurs in animals with even modest levels of positive pressure (e.g., sustained peak airway pressures of 30 cm H2O for 24 hours). Data suggest that injury may be a result of cyclic opening and closing of alveoli or overdistension and stretching of the alveolar capillary membrane at peak inflation. Injury is diminished in these models by limiting maximal ventilatory excursion or by the application of positive end-expiratory pressure (PEEP). Further studies have demonstrated the elaboration of proinflammatory cytokines and the alteration of surfactant structure and function in the lungs of animals as a result of positive-pressure ventilation. Although these studies do not shed light on the etiologic events initiating ARDS, they do support the hypothesis that the ventilatory support necessary to preserve gas exchange may worsen or modify the course of the underlying lung injury.
PATHOPHYSIOLOGY
There are no specific physical or laboratory findings that identify patients with ARDS. Prior to the development of frank respiratory failure, respirations are rapid and shallow, and the patient may be cyanotic. Auscultation usually reveals bronchial breath sounds; rales are often absent. Serum chemistries and blood-cell counts tend to reflect the underlying diseases, rather than ARDS itself. The chest radiograph shows rapidly progressing widespread infiltrates, often with characteristics suggesting alveolar filling. Occasionally, there is a brief early period during which an interstitial infiltrate predominates. Particularly in the early stages, the infiltrates may look patchy or appear to spare some parts of the lung, leading to a mistaken diagnosis of pneumonia. Arterial blood gas analyses typically show markedly reduced Pao2 with a normal or reduced Paco2.
The 1994 AECC definition of ARDS utilized the level of hypoxemia to stage the severity. In that definition, a Pao2/FIO2 ratio between 200 and 300 corresponded to a diagnosis of ALI, while a Pao2/FIO2 ratio of less than 200 corresponded to full-blown ARDS. Despite its overall success and broad use as a research tool, the AECC definition had a number of limitations (such as the absence of a specification for PEEP). An expert panel (the ARDS Definition Task Force) has proposed an expanded definition (Table 67-2) that corrects these deficiencies. In the presence of PEEP or continuous positive airway pressure (CPAP) levels greater than or equal to 5 cm H2O, Pao2 levels between 200 and 300 mmHg correspond to mild ARDS, between 100 and 200 mmHg correspond to moderate ARDS, and those less than or equal to 100 mmHg define severe ARDS. When applied retrospectively, this consensus definition appeared to correlate with mortality and duration of need for mechanical ventilation.
The “Berlin” Definition of Acute Respiratory Distress Syndrome |
Timing | Within 1 wk of a known clinical insult or new or worsening respiratory symptoms |
Chest imaginga | Bilateral opacities—not fully explained by effusions, lobar/lung collapse, or nodules |
Origin of edema | Respiratory failure not fully explained by cardiac failure or fluid overload |
| Need objective assessment (e.g., echocardiography) to exclude hydrostatic edema if no risk factor present |
Oxygenationb |
|
Mild | 200 mmHg < Pao2/FIO2 ≤ 300 mmHg with PEEP or CPAP ≥ 5 cm H2Oc |
Moderate | 100 mmHg < Pao2/FIO2 ≤ 200 mmHg with PEEP ≥ 5 cm H2O |
Severe | Pao2/FIO2 ≤ 100 mmHg with PEEP ≥ 5 cm H2O |
Abbreviations: CPAP, continuous positive airway pressure; FIO2, fraction of inspired oxygen; Pao2, partial pressure of arterial oxygen; PEEP, positive end-expiratory pressure.
aChest radiograph or computed tomography scan.
bIf altitude is higher than 1,000 m, the correction factor should be calculated as follows: (Pao2/Fio2 * [barometric pressure/760]).
c
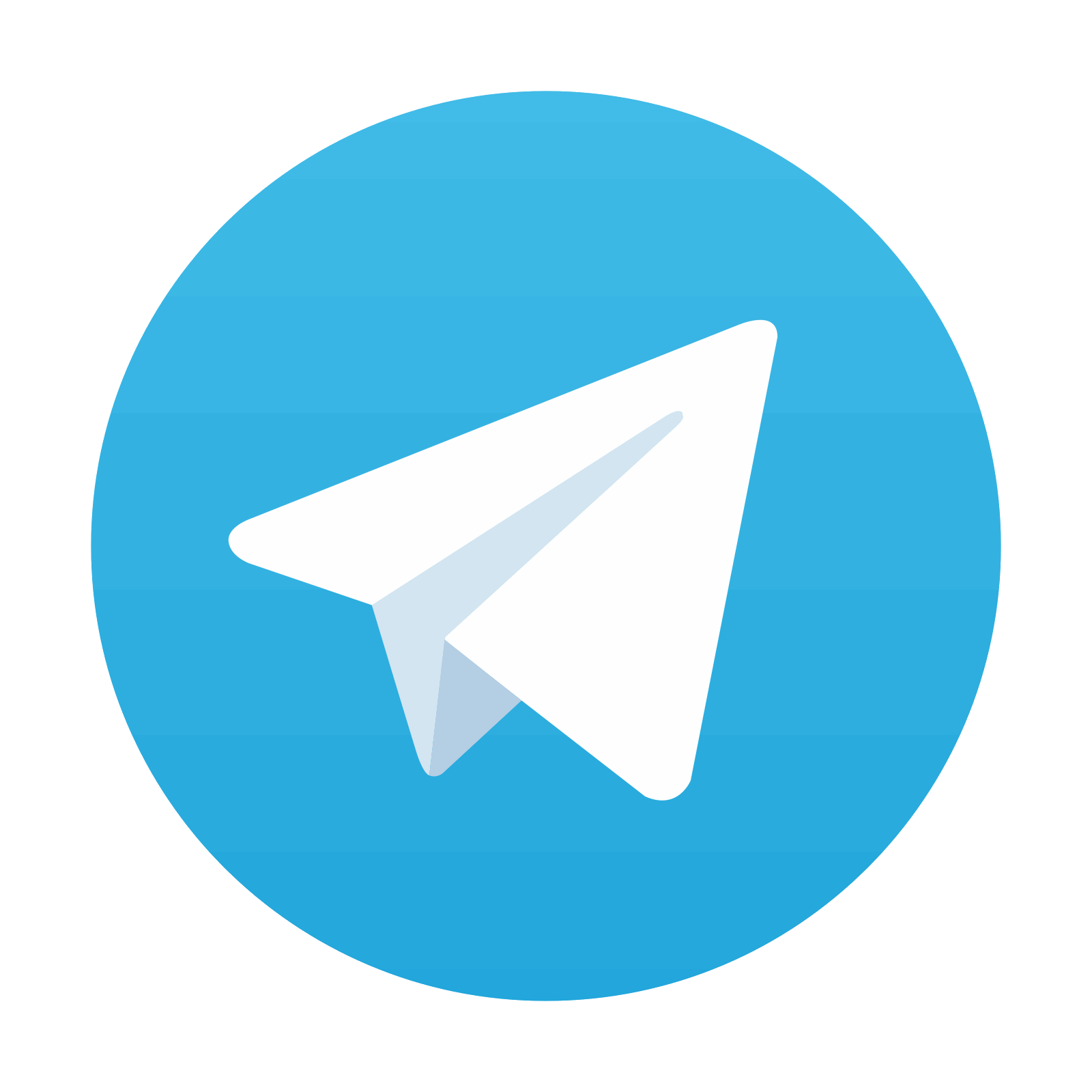
Stay updated, free articles. Join our Telegram channel

Full access? Get Clinical Tree
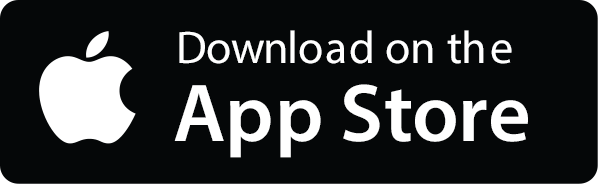
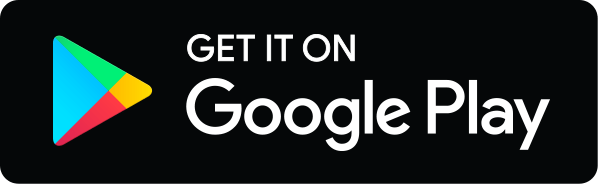