Epidemiology
Left ventricular outflow tract obstruction (LVOTO) occurs in 2.8 of 10,000 live births, accounting for 3 to 6 percent of congenital heart defects.
Morphology
LVOTO is valvar in 50 percent, subvalvar in 25 percent, and supravalvar in 10 percent of cases. Multilevel obstruction is present in 15 percent of cases.
Pathophysiology
Obstruction at any level causes increased left ventricular systolic pressure and wall stress and consequent left ventricular hypertrophy. Left ventricular hypertrophy produces subendocardial ischemia and diastolic dysfunction. Eventually, myocardial fibrosis occurs with associated left ventricular systolic dysfunction. Low cardiac output, pulmonary edema, and ventricular arrhythmias may occur late and are associated with increased mortality.
Clinical features:
Neonates with critical valvar aortic stenosis (AS) present with low cardiac output and shock, requiring emergency treatment. Other forms of LVOTO are often asymptomatic and may be detected by the presence of a heart murmur or abnormalities on ECG. Chest pain, dyspnea, and palpitations occur with increased activity as the degree of obstruction worsens. Symptoms at rest occur with long-standing LVOTO.
Diagnosis
Diagnostic evaluation is performed in children with a characteristic systolic ejection murmur. Echocardiography allows diagnosis and determines the level(s) of obstruction. The left ventricular outflow tract gradient can be estimated with the use of Doppler flow velocity, and ventricular systolic and diastolic function can be assessed. Diagnostic cardiac catheterization is rarely necessary.
Treatment
Neonates with critical valvar AS and morphology amenable to biventricular repair respond well to percutaneous transcatheter balloon valvotomy. Aortic valve replacement is required in older children. Enlargement of a small annulus may be achieved with the use of Konno aortoventriculoplasty. Options for valve replacement include a bioprosthetic or mechanical prosthesis, or an autograft (Ross procedure), but all have significant disadvantages in the pediatric population. Discrete subaortic membrane is resected via aortotomy, but diffuse tunnel-like narrowing requires a modified Konno procedure. A variety of aortoplasty techniques are available for patients with supravalvar AS.
Outcomes
Excellent outcomes, with operative mortality of less than 5 percent, are achieved in most patients. Reoperation to upsize valves is expected in pediatric patients with somatic growth after aortic valve replacement or for structural valve deterioration. Discrete subaortic membrane recurs in approximately 15 to 20 percent of patients despite successful initial repair, and is dependent on the preoperative gradient.
Left ventricular outflow tract obstruction (LVOTO) is a relatively common form of congenital heart disease; it occurs in 2.8 per 10,000 births and accounts for 3 to 6 percent of congenital heart defects.1 The levels of obstruction are classified anatomically as valvar (50 percent), subvalvar (25 percent), and supravalvar (10 percent). Multiple levels of obstruction are present in about 15 percent of cases.2 LVOTO may be associated with other congenital heart defects, including atrioventricular canal defect, double-outlet right ventricle (RV), and some forms of functionally single ventricle. This chapter focuses on isolated congenital LVOTO in functionally biventricular hearts. Aortic arch interruption and coarctation are specifically discussed in Chapter 79.
The left ventricular outflow tract is positioned centrally in the heart in close anatomic relation to other important cardiac structures (Fig. 78-1). Below the level of the aortic valve, the outflow tract is bordered anteriorly and to the left by the infundibular septum. Posteriorly, it is bordered by the anterior leaflet of the mitral valve and by the central fibrous body. The membranous septum is positioned anteriorly and to the right, beneath the junction of the right coronary and noncoronary cusps of the aortic valve. This relationship is surgically important, as the bundle of His courses beneath the membranous septum on the left ventricular aspect and may be injured in the course of resection or suture placement, resulting in complete heart block.
Figure 78-1
Anatomy of the left ventricular outflow tract. A. Cranial view. The aortic valve is centrally positioned between the mitral and tricuspid valves. B. Schematic left-sided view: The noncoronary aortic cusp is in fibrous continuity with the anterior leaflet of the mitral valve. The bundle of His courses below the membranous septum. Ao, aorta; MV, mitral valve; TV, tricuspid valve; LA, left atrium. (Reproduced with permission from Shizuka N. Aoki.)

The normal aortic valve consists of three thin leaflets of equal size that are attached to the aortic wall in a semilunar fashion. The so-called aortic annulus, therefore, is not a circular ring but a scalloped coronet.3 The free edge of each leaflet has an area of central thickening termed the nodulus of Arantius. The leaflet edges coapt in diastole and open freely in systole, creating an unobstructed triangular orifice for the ejection of blood from the left ventricle to the aorta. Leaflets are designated in accordance with the adjacent coronary artery ostia as left, right, and noncoronary cusps.
The proximal ascending aorta constitutes the distal left ventricular outflow tract. Immediately above the ventriculoarterial junction, the aorta enlarges to form the sinuses of Valsalva. These sinuses, like their corresponding valve leaflets, are named for the coronary artery ostia that arise from them. They are important for normal aortic valve function and coronary artery blood flow.4 The sinotubular junction refers to the normal area of aortic narrowing at the junction between the sinuses of Valsalva and the tubular portion of the ascending aorta. The uppermost point of aortic valve leaflet attachment occurs at the sinotubular junction.
Regardless of the etiology, obstruction to flow through the left ventricular outflow tract results in increased left ventricular systolic pressure and wall stress. Left ventricular hypertrophy develops as a compensatory mechanism that maintains normal wall stress and stroke volume in accordance with Laplace’s law. However, increased ventricular wall mass increases myocardial oxygen demand while limiting diastolic coronary blood flow. Oxygen demand eventually exceeds supply, resulting in myocardial ischemia.5
Left ventricular systolic function is initially normal or hyperdynamic in the early stages of obstruction. With progressive left ventricular hypertrophy, decreased wall compliance restricts ventricular filling and produces diastolic dysfunction. A decreased preload reduces stroke volume and cardiac output. Left ventricular end-diastolic and left atrial pressures rise, resulting in left atrial hypertrophy. Patients with long-standing obstruction develop myocardial fibrosis, areas of ischemic injury, and decreased ventricular systolic function. Low cardiac output, pulmonary edema, and ventricular arrhythmias ensue, associated with an increasing risk of sudden death.
Relief of LVOTO ideally is achieved before the development of systolic dysfunction. Early relief of obstruction leads to regression of ventricular hypertrophy and normalization of cardiac function. In the later stages, some components of ventricular dysfunction are likely to be irreversible despite relief of obstruction. Surgical repair in these patients is associated with higher risk and poorer outcomes.
Valvar aortic stenosis (AS) in children is most commonly congenital in etiology. The leaflets are thickened and dysplastic, with variable degrees of commissural fusion (Fig. 78-2). The valve is typically bicuspid in morphology but may be tricuspid or even unicuspid. Fusion of the right and left cusps is associated most frequently with stenosis.6 Obstruction results from decreased leaflet mobility and a reduction in effective orifice size. Small annular size also may be present, further impeding left ventricular ejection.
Figure 78-2
Stenotic bicuspid aortic valve. Left: The leaflets are asymmetric, with a larger leaflet resulting from the fusion between the left and right coronary cusps at the raphe (R), poorly coapting against the thickened noncoronary leaflet (NC). Right: Severe calcific stenosis of the bicuspid aortic valve. (Image courtesy of Dr. Duke Cameron, Division of Cardiac Surgery, Johns Hopkins University Hospital.)

Neonates with critical AS develop symptoms soon after birth. After closure of the ductus arteriosus, severe outflow obstruction results in pulmonary congestion and poor peripheral perfusion. Dyspnea, tachypnea, and rales are present on pulmonary examination. A systolic ejection murmur may be present, but it may be difficult to hear when cardiac output is reduced severely. The distal extremities have cold, clammy skin with poor capillary refill and thready, rapid pulses. The differential diagnosis includes other causes of shock, including sepsis and other forms of congenital heart disease. Prompt diagnosis and institution of therapy are necessary to prevent rapid deterioration and death.
Older children present less acutely, often with the finding of an asymptomatic heart murmur on routine physical examination. Difficulty feeding in infants and decreased exercise tolerance in older children may be observed as the severity of obstruction increases. Later symptoms include exertional angina, congestive heart failure, and syncope. Physical findings are generally limited to the cardiovascular examination: a harsh systolic ejection murmur at the right upper sternal border radiating to the neck, S4 gallop, and poor upstroke of the carotid pulse. An ejection click may indicate the presence of a bicuspid valve.
Chest x-ray is usually nondiagnostic. In neonates with critical stenosis, cardiomegaly and pulmonary congestion are present. Findings in older children generally are limited to moderate left ventricular enlargement, left atrial enlargement, and prominence of the aortic knob.
The electrocardiogram shows left ventricular hypertrophy. Serial findings of increasing QRS voltage and the development of a strain pattern suggest worsening obstruction.
Echocardiography is the principal diagnostic method for the evaluation of LVOTO. Two-dimensional echocardiography allows assessment of aortic valve morphology including leaflet number, degree of thickening, and mobility. Doming of the leaflets is typical (Fig. 78-3). Dimensions of the subvalvar area, annulus, effective valve orifice, and aortic root are measured accurately. Left ventricular cavity size and the presence and severity of left ventricular hypertrophy can be assessed. Indexes of systolic and diastolic function can be calculated. Color Doppler imaging accurately identifies the level of obstruction, allowing a distinction between valvar, subvalvar, and supravalvar stenosis. Doppler measurement of blood flow velocity across the valve provides an estimate of the peak pressure gradient. Progression of disease and timing of intervention, therefore, can be determined in a noninvasive fashion in most cases.
Figure 78-3
Two-dimensional echocardiogram in a patient with congenital aortic stenosis. Long-axis view of bicuspid valve. The leaflets are doming in systole. Ao, aorta; BAV, bicuspid aortic valve; LV, left ventricle; RV, right ventricle. (Image courtesy of Dr. William Ravekes, Division of Pediatric Cardiology, Johns Hopkins Hospital.)

With accurate echocardiographic assessment, cardiac catheterization is rarely required in the diagnostic evaluation of AS. It may be indicated to confirm the diagnosis or assess the severity of obstruction when the clinical and echocardiographic findings are equivocal. Direct simultaneous measurement of left ventricular pressure and aortic pressure is the most accurate method for assessing the outflow tract gradient. However, identification of the precise level of obstruction may not be possible. Elevation of left ventricular end-diastolic pressure indicates impaired diastolic function. Left ventriculography allows assessment of ventricular systolic function and may outline the valve leaflets, providing some assessment of morphology.
Critical AS diagnosed in the newborn period constitutes a medical emergency. A neonatal presentation indicates severe outflow obstruction that requires urgent intervention. Initial stabilization includes endotracheal intubation and inotropic support. Prostaglandin infusion will establish or maintain patency of the ductus arteriosus and improve systemic perfusion. Emergency aortic valvotomy previously was the treatment of choice, performed soon after resuscitation. In the current era, percutaneous transcatheter balloon aortic valvotomy has supplanted surgical valvotomy in most centers. The procedure is performed in the catheterization laboratory, with the advantages of rapid relief of obstruction and avoidance of cardiopulmonary bypass and aortic cross-clamping. Risk factors for failure include a small aortic annulus, a bicuspid aortic valve, poor left ventricular function, and limited operator experience.7,8 Outcomes are generally similar to those for open valvotomy. Hospital mortality for either procedure ranges between 0 and 20 percent.9,10 Mortality is higher in patients with bicuspid valve morphology. Significant aortic regurgitation is more likely after balloon valvotomy, and is associated with an increased risk of early death.11 Earlier reports suggested that residual stenosis was more common after surgical valvotomy,9 though this has not borne out in recent studies. Miyamoto et al. reported an 85 percent freedom from reintervention at 5 years following surgical valvotomy among 34 neonates.12 Zain and colleagues similarly found that neonates undergoing balloon valvotomy had a higher reintervention rate than those having surgical valvotomy despite a more modest immediate gradient reduction following surgical valvotomy.13 Regardless of the technique, neonatal aortic valvotomy is considered a palliative procedure for most patients, with 41 percent undergoing reintervention within 10 years.9,14
In a small subset of neonates with critical AS and small left ventricular size, it may be difficult to determine whether a biventricular approach with aortic valvotomy/replacement or a single-ventricle approach with the Norwood procedure is more appropriate.15 Several studies have attempted to identify preoperative predictors for suitability of single ventricle versus biventricular repair in this challenging population.15 Hammon et al.16 proposed an angiographically derived 20 mL/m2 threshold for end-diastolic volume for successful biventricular repair. Rhodes and associates17 identified criteria, including body surface area, indexed aortic root dimension, ratio of left ventricle to heart length, and indexed mitral valve area, as predictors of successful valvotomy. However, the Rhodes score was developed using a small, retrospective group of 65 infants with valvar AS who were pre-selected for biventricular repair. The score has recently been revalidated by Colan et al.,18 but still has shown poor discrimination when applied to neonates with multiple levels of left heart obstruction or whose primary pathology is other than critical AS. The most robust clinical tool for triaging patients to a single or biventricular pathway, termed the “Critical Aortic Stenosis calculator,” was developed by the Congenital Heart Surgeons’ Society (CHSS). The calculator was derived from a prospective multi-institutional cohort of 320 patients with critical AS or atresia. Multivariable linear regression analysis identified independent factors associated with survival after biventricular repair versus single ventricle palliation.19 The derived regression equation requires the following values: age at entry, aortic valve z-score, grade of endocardial fibroelastosis, diameter of ascending aorta, presence of moderate or severe tricuspid regurgitation, and z-score of left ventricular length. A positive number favors the Norwood approach, a negative number favors biventricular repair, and the magnitude of the number represents the degree of the predicted survival benefit. Individual patient data can be entered and the relative risk of either approach can be estimated by using the website of the Congenital Heart Surgeons Society (www.chssdc.org). An updated analysis of the same CHSS cohort by Hickey et al.20 allowed refinement of the CHSS prediction algorithm, with substitution of two variables (mid-aortic arch diameter and minimum LVOT diameter) as surrogates for patient age, and the addition of the z-score of the mitral valve annulus.
Neonates with multiple levels of LVOTO represent a complex group. Surgical options must be tailored to the particular anatomic considerations (Fig. 78-4). Neonates undergoing single ventricle palliation can be managed with a staged approach, consisting of a Norwood or modified Norwood operation. Hybrid palliation, with implantation of a ductal stent with concomitant bilateral pulmonary arterial (PA) banding, is another recently developed option. This strategy, however, is generally reserved for marginal candidates with contraindications to standard Norwood-type palliation or as a bridge to heart transplantation. Orthotopic heart transplantation is an attractive strategy for infants with severe atrioventricular valve regurgitation, visceral heterotaxy, or poor systemic ventricular function.
Neonates undergoing biventricular repair have several potential options depending upon the associated intracardiac and arch pathology. Infants with combined valvar AS and aortic arch obstruction can be managed with standard patch aortoplasty concomitant with either a neonatal Ross operation or a mechanical aortic valve replacement with an annular enlargement (Konno aortoventriculoplasty). Our institutional preference has been to manage these patients with aortic valve replacement and Konno root augmentation, as results with the neonatal Ross operation have been poor, especially in neonates with associated mitral valve abnormalities.15,21–23 The presence of a ventricular septal defect adds an additional level of complexity, with options including those discussed above, arch repair with closure of the ventricular septal defect, or a Yasui reconstruction.
The objectives of surgical treatment of AS are relief of symptoms and reduction of the risk of sudden death. Sudden death is related directly to the severity of obstruction and correlates with the peak systolic gradient, though it is reported to be less than 1 percent.24 Stenosis is considered severe when the mean echocardiographic gradient is equal to or greater than 40 mm Hg, moderate when it is between 25 and 40 mm Hg, and mild when it is <25 mm Hg.24 Surgery is indicated in any symptomatic patient regardless of severity, and in asymptomatic patients with severe stenosis. Surgery is not recommended for asymptomatic patients with mild stenosis. There is controversy about the management of asymptomatic patients with moderate stenosis. Surgical management should be considered on an individual basis. The authors generally recommend surgery in patients who demonstrate reduced left ventricular function, a strain pattern on electrocardiography or abnormal exercise testing.
The two options for surgical management of AS are valvotomy and aortic valve replacement. Valve replacement in children poses unique challenges that are not seen in the adult population. The smallest mechanical and bioprosthetic valves available (17-19 mm) are too large for the annular size of smaller children. Even when replacement is feasible, unless an “adult-size” valve is used, somatic growth eventually results in recurrent outflow obstruction because the prosthesis size is fixed. Re-replacement with an appropriately sized valve may be limited by restricted annular growth from the original prosthesis. These limitations may necessitate concomitant annular enlargement, such as in a Konno procedure. Posterior enlargement procedures such as the Nicks or Manougian operations are often insufficient in children.25,26
The choices of prosthetic valves for children are more limited than are those for adults. Bioprostheses do not require anticoagulation, but calcific degeneration occurs at an accelerated rate compared with adults, limiting their use in children.27 Mechanical prostheses maintain their structural integrity and generally have excellent freedom from reintervention or re-replacement,28–30 but require anticoagulation with warfarin. Anticoagulation can be achieved safely in children, but issues of compliance, inconsistent diet, and potential for traumatic injury increase the risk of bleeding and thromboembolic complications in this patient population. A recent paper by Alsoufi and colleagues31 demonstrated that the use of either homograft or bioprosthetic valves in children was associated with an increased risk of valve-related reoperation (82 percent at 15 years) compared to both mechanical valve implantation and the Ross operation. However, long-term survival was excellent (86 percent at 15 years) in patients having homograft or bioprosthetic valve replacement, underscoring the fact that these valve types can be useful in female patients or those in whom anticoagulation cannot be utilized.
Allograft replacement of the aortic root became popular in the early 1990s. Small valves with excellent early hemodynamic performance were readily available, and it was hoped that degeneration would not occur. Subsequent reports, however, demonstrated severe accelerated degeneration that required early reoperation in younger children.32 Severe calcification and inflammatory reaction of surrounding tissues complicate allograft removal and place adjacent structures, including mitral valve, the bundle of His and the coronary arteries at risk during reoperation.
Pulmonary autografting and reconstruction of the RV outflow tract with an allograft (the Ross procedure) are often the best choice for aortic valve replacement in small children. The autograft has growth potential, does not develop calcific degeneration, and does not require anticoagulation. There are, however, a number of important limitations. The aortic and pulmonary annular sizes must be similar; stretching of the autograft to fit a dilated aortic root will result in neoaortic insufficiency. The operation is technically challenging, requiring longer periods of cardiopulmonary bypass and aortic cross-clamping compared with other, simpler techniques for valve replacement. Allograft reconstruction of the RV outflow tract invariably necessitates eventual reoperation for conduit stenosis from somatic growth or calcific degeneration. Finally, it has been recognized that the pulmonary autograft develops important dilation over time out of proportion to somatic growth, and that this dilation can result in progressive neoaortic insufficiency.33,34 The propensity for neoaortic dilation was initially thought to be limited to those with aortopathy associated with a bicuspid aortic valve. However, data from Pasquali et al.33 and others34 demonstrated that, using serial echocardiographic data, freedom from neoaortic root intervention was 88 percent at 6 years, freedom from moderate neoaortic regurgitation was 60 percent, and freedom from neoaortic root size Z-score more than 4 was only 3 percent. Interestingly, several recent papers33,34 reported that autograft dilation was not associated with the development of neoaortic insufficiency, which was the main reason for reintervention.
In summary, there is no ideal substitute for aortic valve replacement in children with valvar AS. Aortic valvotomy is, whenever feasible, the procedure of choice in this population. Although often not definitive, adequate palliation is usually achieved. Small residual gradients and mild aortic insufficiency are acceptable. Valvotomy can defer the need for valve replacement significantly, allowing for insertion of a larger prosthesis at a later time and reduction of the total number of re-replacements required over a patient’s lifetime.
Aortic Valvotomy. Surgical aortic valvotomy is usually performed via a median sternotomy. A single right atrial cannula provides venous drainage, and the aortic cannula is placed as distally as possible. Some surgeons advocate closed valvotomy that is performed by passing dilators of increasing size (usually up to 1 mm larger than the preoperative echocardiographic aortic annulus diameter) through the aortic valve; the dilators are introduced through a purse string in the left ventricular apex. This technique can be performed through a left thoracotomy without the need for cardiopulmonary bypass.35 The authors believe that open valvotomy with direct visualization of the aortic valve allows more precise leaflet separation, resulting in better relief of gradient and a decreased risk of significant aortic insufficiency.
The aorta is clamped, and cardioplegia is delivered via the aortic root. A transverse aortotomy is made just above the sinotubular junction, with care taken to avoid injury to the right coronary artery and the aortic valve leaflets. The valve is inspected carefully. Fused commissures are opened precisely with a scalpel (Fig. 78-5). The incisions are not extended to the aortic wall, since this may result in loss of support and aortic insufficiency. Excessive fibrous tissue, if present, is excised from the leaflets. Fibrous attachments between the base of the leaflets and the aortic wall are divided to maximize leaflet mobility. Despite these steps, the effective orifice may be inadequate, particularly in smaller bicuspid valves. Ilbawi and colleagues36 described a technique of extended valvotomy for these patients. Circumferential incisions are made above the true commissures and raphes (Fig. 78-6). They reported a low incidence of aortic insufficiency and significantly lower gradients compared with standard valvotomy. The aortotomy is closed precisely to avoid supravalvar narrowing, and the cross-clamp is removed. The patient is weaned from cardiopulmonary bypass with mild inotropic support (dopamine 5 μg/kg/min and milrinone 0.5 μg/kg/min). The adequacy of repair is assessed by transesophageal echocardiography and direct measurement of pressure in the left ventricle and ascending aorta. Modified ultrafiltration is performed before decannulation.
Early mortality in older infants and children undergoing aortic valvotomy is reported currently to be less than 2 percent,2,7 and late mortality is rare. Reintervention for progressive regurgitation or restenosis may be required, but this generally occurs later than in neonates. Reoperation rate in children over 1 year of age at the time of the initial valvotomy is 2 percent at 10 years but increases thereafter by 3.3 percent per year.37
Aortic Valve Replacement. Aortic valve replacement is required when valvotomy is not sufficient to reduce the transvalvar gradient adequately, or in patients with greater than mild aortic insufficiency. In larger children with adequate annular size, simple replacement of the valve is performed as it is in adults. Prosthesis selection should be individualized. The authors generally avoid porcine and bovine bioprostheses as well as allograft implantation because of the rapid degeneration and early failure observed in children. The remaining options, therefore, are limited to mechanical prosthesis and pulmonary autograft.
Aortic valve replacement with a mechanical prosthesis is performed via a median sternotomy. A single right atrial or two-stage venous cannula provides venous drainage, and the ascending aorta is cannulated distally. The aorta is clamped, and in the absence of significant aortic insufficiency, cardioplegia is infused in the aortic root. After aortotomy, additional cardioplegia is delivered every 30 min through a retrograde coronary sinus catheter or by direct coronary ostial perfusion. A left ventricular vent is placed through the left atrial appendage or the right superior pulmonary vein.
An oblique aortotomy is made and is extended into the noncoronary sinus of Valsalva. The aortic valve leaflets are excised. Interrupted pledgeted mattress sutures are placed circumferentially around the annulus. In smaller patients, intra-annular placement may be preferable to avoid coronary ostial obstruction by the sewing ring. The sutures are passed through the sewing ring, and the valve is parachuted into place. After the sutures are tied, the prosthetic leaflets are assessed carefully to ensure unhindered mobility. The aortotomy is closed, and the aortic clamp is removed. The patient is weaned from cardiopulmonary bypass on mild inotropic support. Transesophageal echocardiography is used to assess prosthetic function. Modified ultrafiltration is performed before decannulation.
Hospital mortality after mechanical aortic valve replacement in children is less than 5 percent. Early complications include permanent heart block in 3 percent and acute endocarditis in 2 percent of these patients. Late complications relate primarily to anticoagulation. In reports of long-term follow-up, valve thrombosis occurs in up to 2 percent of patients. This sometimes can be managed pharmacologically with thrombolytic agents but frequently requires urgent surgical thrombectomy or valve replacement. Embolic events are reported in 2 percent, and significant bleeding episodes occur at a rate of 0.15 percent/patient year.38,39 Freedom from reintervention or re-replacement is approximately 86 percent at 20 years, but is increased in younger patients having smaller prostheses.28–31
Ross Procedure. Ross40 reported aortic valve replacement with a pulmonary autograft and allograft reconstruction of the RV outflow tract in 1967 (Fig. 78-7). In the absence of significant size discrepancy or connective tissue disease, the Ross procedure is the preferred technique for aortic valve replacement in small children. In larger children, it frequently is preferred over mechanical prosthesis to avoid the need for anticoagulation.
Figure 78-7
Ross procedure. A. Dotted lines indicate incisions in the aorta, pulmonary trunk, and right ventricular infundibulum. B. The aorta and pulmonary artery are transected, and the coronary buttons are excised from the sinuses of Valsalva. C. Removal of the pulmonary autograft. D. The proximal anastomosis of the pulmonary autograft is begun posteriorly. E. The proximal autograft anastomosis is completed, and the coronary buttons are sutured to the autograft sinuses of Valsalva. F. A homograft restores continuity of the right ventricular outflow tract. G. The autograft is anastomosed distally to the ascending aorta. Ao, aorta; LAD, left anterior descending coronary artery; LCA, left coronary artery; PA, pulmonary artery; RCA, right coronary artery; RV, right ventricle. (Reproduced with permission from Shizuka N. Aoki.)

The approach is via a median sternotomy. The venae cavae are cannulated individually, and the ascending aorta is cannulated distally. The aorta is clamped, and in the absence of significant aortic insufficiency, cardioplegia is infused in the aortic root. Cardioplegia may also be delivered in a retrograde fashion with a coronary sinus catheter or by direct coronary ostial perfusion after an aortotomy. Additional cardioplegia is delivered every 30 min during the cross-clamp period. A left ventricular vent is placed through the left atrial appendage or the right superior pulmonary vein.
The aorta and the pulmonary trunk are separated, and the pulmonary artery is opened transversely just proximal to the bifurcation. The pulmonary valve is inspected to identify any pathology that would preclude its use as an aortic valve substitute. Transection of the pulmonary trunk is then completed. A right-angle clamp is passed carefully through the leaflets into the RV outflow tract, and a site for proximal transection is identified in the infundibular free wall approximately 5 mm below the level of the valve. A transverse infundibular incision is made and carried to the infundibular septum at each end. The infundibular septum is scored with a scalpel blade. A plane can be developed between the subconal muscle and the underlying interventricular septum. Dissection in this plane completes the harvest, and the autograft is stored in normal saline solution before implantation. The resulting posterior raw surface is cauterized. In their practice, the authors also apply a thin layer of biological sealant to ensure hemostasis. In dissecting the autograft, care must be taken at the leftward extent of the septal dissection to avoid injury to the first septal perforating branch of the left anterior descending coronary artery. The PA is sized, and an appropriate allograft is thawed and prepared for reconstruction of the RV outflow tract.
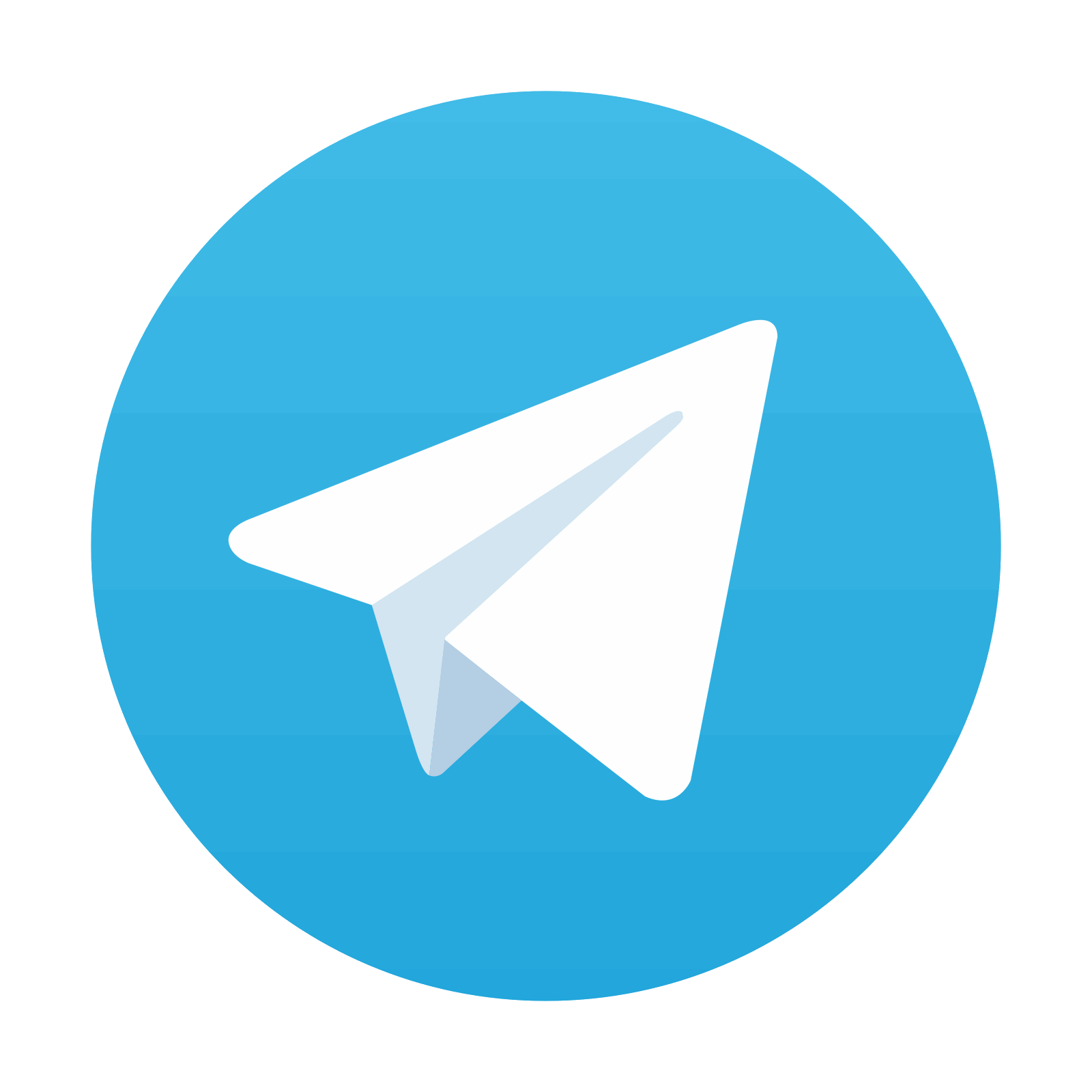
Stay updated, free articles. Join our Telegram channel

Full access? Get Clinical Tree
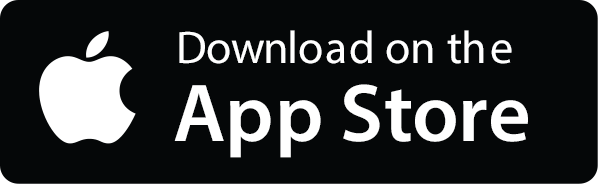
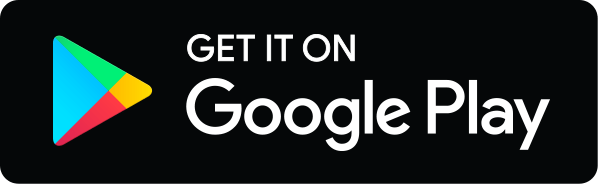