Objectives
Upon completion of this chapter, the student should be able to answer the following questions :
- •
Which structures in the glomerulus are filtration barriers to plasma proteins?
- •
What is the physiologic significance of the juxtaglomerular apparatus?
- •
Which blood vessels supply the kidneys?
- •
Which nerves innervate the kidneys?
In addition, the student should be able to describe the following :
- •
The location of the kidneys and their gross anatomic features
- •
The different parts of the nephron and their locations within the cortex and medulla
- •
The components of the glomerulus and the cell types located in each component
Key Terms
Cortex
Medulla
Nephrons
Renal pyramids
Minor calyx
Major calyces
Pelvis
Urinary bladder
Interlobar artery
Arcuate artery
Interlobular artery
Afferent arteriole
Glomerular capillaries
Efferent arteriole
Peritubular capillaries
Interlobular vein
Arcuate vein
Interlobar vein
Renal vein
Renal corpuscle
Proximal tubule
Loop of Henle
Distal tubule
Collecting duct systems
Bowman’s capsule
Descending thin limb (of Henle)
Ascending thin limb (of Henle)
Thick ascending limb (of Henle)
Macula densa
Glomerulus
Cortical collecting duct
Outer medullary collecting duct
Inner medullary collecting duct
Brush border
Principal cells
Intercalated cells
Autosomal dominant polycystic kidney disease
Superficial nephrons
Juxtamedullary nephrons
Vasa recta
Collecting ducts
Bowman’s space
Podocytes
Visceral layer
Parietal layer
Filtration barrier
Filtration slits
Filtration slit diaphragm
Nephrin (NPHS1)
NEPH-1
Podocin (NPHS2)
CD2-AP
α-actinin 4 (ACTN4)
Proteinuria
Alport’s syndrome
Mesangium
Mesangial cells
Mesangial matrix
Extraglomerular mesangial cells
Immune complex–mediated glomerular disease
Juxtaglomerular apparatus (JGA)
Renin
Angiotensin II
Aldosterone
Nephrotic syndrome
Structure and function are closely linked in the kidneys. Consequently, an appreciation of the gross anatomic and histologic features of the kidneys is a prerequisite for an understanding of their functions.
Structure of the Kidneys
Gross Anatomy
The kidneys are paired organs that lie on the posterior wall of the abdomen behind the peritoneum on either side of the vertebral column. In the adult human, each kidney weighs between 115 g and 170 g and is approximately 11 cm long, 6 cm wide, and 3 cm thick.
The gross anatomic features of the human kidney are illustrated in Fig. 2.1A . The medial side of each kidney contains an indentation through which pass the renal artery and vein, nerves, and pelvis. If a kidney were cut in half, two regions would be evident: an outer region called the cortex and an inner region called the medulla . The cortex and medulla are composed of nephrons (the functional units of the kidney), blood vessels, lymphatics, and nerves. The medulla in the human kidney is divided into conical masses called renal pyramids . The base of each pyramid originates at the corticomedullary border, and the apex terminates in a papilla, which lies within a minor calyx . Minor calyces collect urine from each papilla. The numerous minor calyces expand into two or three open-ended pouches, which are the major calyces. The major calyces in turn feed into the pelvis . The pelvis represents the upper, expanded region of the ureter , which carries urine from the pelvis to the urinary bladder. The walls of the calyces, pelvis, and ureters contain smooth muscle that contracts to propel the urine toward the urinary bladder .

The blood flow to the two kidneys is equal to about 25% (1.25 L/min) of the cardiac output in resting individuals. However, the kidneys constitute less than 0.5% of total body weight. As illustrated in Fig. 2.1B , the renal artery branches progressively to form the interlobar artery , the arcuate artery , the interlobular artery , and the afferent arteriole , which leads into the glomerular capillaries . The glomerular capillaries come together to form the efferent arteriole, which leads into a second capillary network, the peritubular capillaries , which supply blood to the nephron. The vessels of the venous system run parallel to the arterial vessels and progressively form the interlobular vein, arcuate vein, interlobar vein , and renal vein , which courses beside the ureter.
Ultrastructure of the Nephron
The functional unit of the kidneys is the nephron. In young adults each human kidney contains approximately 1 million nephrons, which are essentially hollow tubes composed of a single epithelial cell layer. With age, however, the number of nephrons declines—for instance, by about 50% for individuals in their 70s—thereby reducing the functional capacity of the kidneys and increasing the risk for high blood pressure (see Chapter 6 for a discussion on how the kidneys regulate extracellular fluid volume and blood pressure). The nephron consists of a renal corpuscle , proximal tubule, loop of Henle, distal tubule , and collecting duct system a ( Fig. 2.2 ). The renal corpuscle b consists of glomerular capillaries enclosed within Bowman’s capsule . The proximal tubule exits this structure and initially forms several coils, followed by a straight piece that descends toward the medulla. The next segment is the loop of Henle, which is composed of the straight part of the proximal tubule, the descending thin limb (which ends in a hairpin turn), the ascending thin limb (only in nephrons with long loops of Henle), and the thick ascending limb . Near the end of the thick ascending limb, the nephron passes between the afferent and efferent arterioles of the same nephron. This short segment of the thick ascending limb abutting the glomerulus is called the macula densa (see Fig. 2.2 ). The distal tubule begins a short distance beyond the macula densa and extends to the point in the cortex where two or more nephrons join to form a cortical collecting duct. The cortical collecting duct enters the medulla and becomes the outer medullary collecting duct and then the inner medullary collecting duct .
a The organization of the nephron is more complicated than presented here. However, for simplicity and clarity of presentation in subsequent chapters, the nephron is divided into five segments. The collecting duct system is not actually part of the nephron. However, again for simplicity, we consider the collecting duct system part of the nephron.
b Although the renal corpuscle is composed of glomerular capillaries and Bowman’s capsule, the term glomerulus is commonly used to described the renal corpuscle.

Each nephron segment is made up of cells that are uniquely suited to perform specific transport functions ( Fig. 2.3 ). Proximal tubule cells have an extensively amplified apical membrane (the ultrafiltrate or urine side of the cell) called the brush border , which is present only in the proximal tubule of the nephron. The basolateral membrane (the interstitial or blood side of the cell) is highly invaginated. These invaginations contain many mitochondria. In contrast, the descending and ascending thin limbs of Henle’s loop have poorly developed apical and basolateral surfaces and few mitochondria. The cells of the thick ascending limb and the distal tubule have abundant mitochondria and extensive infoldings of the basolateral membrane.

The collecting duct is composed of two cell types: principal cells and intercalated cells. Principal cells have a moderately invaginated basolateral membrane and contain few mitochondria. Principal cells play an important role in sodium chloride (NaCl) reabsorption (see Chapter 4, Chapter 6 ) and potassium (K + ) secretion (see Chapter 7 ). Intercalated cells , which play an important role in regulating acid-base balance , have a high density of mitochondria. One population of intercalated cells secretes H + (i.e., reabsorbs bicarbonate, HCO 3 – ) and a second population of intercalated cells secretes HCO 3 – (see Chapter 8 ). Intercalated cells can either reabsorb K + or secrete K + , depending on K + balance (see Chapter 7 ). The final segment of the nephron, the inner medullary collecting duct, is composed of inner medullary collecting duct cells that have poorly developed apical and basolateral surfaces and few mitochondria.
Except for intercalated cells, all cells in the nephron have in the apical plasma membrane a single nonmotile primary cilium that protrudes into tubule fluid ( Fig. 2.4 ). Primary cilia are mechanosensors (i.e., they sense changes in the flow rate of tubule fluid) and chemosensors (i.e., they sense or respond to compounds in the tubule fluid), and they initiate Ca ++ -dependent signaling pathways, including those that control kidney cell function, proliferation, differentiation, and apoptosis (i.e., programmed cell death).
Polycystin 1 (encoded by the PKD1 gene) and polycystin 2 (encoded by the PKD2 gene) are expressed in the membrane of primary cilia and mediate entry of Ca ++ into cells. PKD1 and PKD2 are thought to play an important role in flow-dependent K + secretion by principal cells of the collecting duct. As described in more detail in Chapter 7 , increased flow of tubule fluid in the collecting duct is a strong stimulus for K + secretion. Increased flow bends the primary cilium in principal cells, which activates the PKD1/PKD2 Ca ++ conducting channel complex, allowing Ca ++ to enter the cell and increase intracellular [Ca ++ ]. The increase in [Ca ++ ] activates K + channels in the apical plasma membrane, which enhances K + secretion from the cell into the tubule fluid.
Autosomal dominant polycystic kidney disease (ADPKD) is the most common inherited kidney disease, occurring in about 1 in 1000 people. Approximately 12.5 million people worldwide have ADPKD, which is caused primarily by mutations in PKD1 (85% of cases) or PKD2 (∼15% of cases). The major phenotype of ADPKD is enlargement of the kidneys as a result of the presence of hundreds or thousands of space-occupying renal cysts, which can be as large as 20 cm in diameter. Cysts also occur in the liver and other organs in this condition. About 50% of patients with ADPKD progress to renal failure by the age of 60, which requires dialysis or a kidney transplant. Although it is not clear how mutations in PKD1 and PKD2 cause ADPKD, renal cyst formation may result from defects in Ca ++ uptake that alter Ca ++ -dependent signaling pathways, including those controlling kidney cell proliferation, differentiation, and apoptosis.

Nephrons may be subdivided into superficial and juxtamedullary types (see Fig. 2.2 ), with approximately 10 superficial nephrons for each juxtamedullary nephron. The glomerulus of each superficial nephron is located in the outer region of the cortex. The corresponding loops of Henle are short, and associated efferent arterioles branch into peritubular capillaries that surround its associated nephron segments, as well as those of adjacent nephrons (see Fig. 2.1B ). This capillary network conveys oxygen and important nutrients to the nephron segments in the cortex, delivers substances to individual nephron segments for secretion (i.e., the movement of a substance from the blood into the tubular fluid), and serves as a pathway for the return of reabsorbed water and solutes to the circulatory system. A few species, including humans, also possess very short superficial nephrons whose Henle loops never enter the medulla.
The glomerulus of each juxtamedullary nephron is located in the region of the cortex adjacent to the medulla (see Fig. 2.2 ). Compared with the superficial nephrons, the juxtamedullary nephrons differ anatomically in two important ways: The loop of Henle is longer and extends deeper into the medulla, and the efferent arteriole forms not only a network of peritubular capillaries but also a series of accompanying vascular loops called the vasa recta .
As shown in Fig. 2.1B , the vasa recta descend into the medulla, where they form capillary networks that surround the collecting ducts and ascending limbs of the loop of Henle. The blood returns to the cortex via the ascending vasa recta. Although less than 0.7% of the blood enters the vasa recta, these vessels subserve important functions in the renal medulla, which include (1) conveying oxygen and important metabolic substrates to support nephron function, (2) delivering substances to the nephron for secretion, (3) serving as a pathway for the return of reabsorbed water and solutes to the circulatory system, and (4) concentrating and diluting the urine. (Urine concentration and dilution are discussed in more detail in Chapter 5 .)
Ultrastructure of the Glomerulus
The first step in urine formation begins with the passive movement of a plasma ultrafiltrate from the glomerular capillaries into Bowman’s space. The term ultrafiltration refers to this passive movement of fluid, which is similar in composition to plasma, except for the fact that the protein concentration in the ultrafiltrate is lower than that in the plasma, from the glomerular capillaries into Bowman’s space. To appreciate this process of ultrafiltration, one must understand the anatomy of the glomerulus, which consists of a network of capillaries supplied by the afferent arteriole and drained by the efferent arteriole ( Fig. 2.5 ). During embryologic development, the glomerular capillaries press into the closed end of the proximal tubule, forming Bowman’s capsule. As the epithelial cells thin on the outside circumference of Bowman’s capsule, they form the parietal epithelium ( Fig. 2.6 ). The epithelia cells in contact with the capillaries thicken and develop into podocytes , which form the visceral layer of Bowman’s capsule (see Figs. 2.6 to 2.8 ). The space between the visceral layer and the parietal layer is Bowman’s space, which at the urinary pole (i.e., where the proximal tubule joins Bowman’s capsule) of the glomerulus becomes the lumen of the proximal tubule.




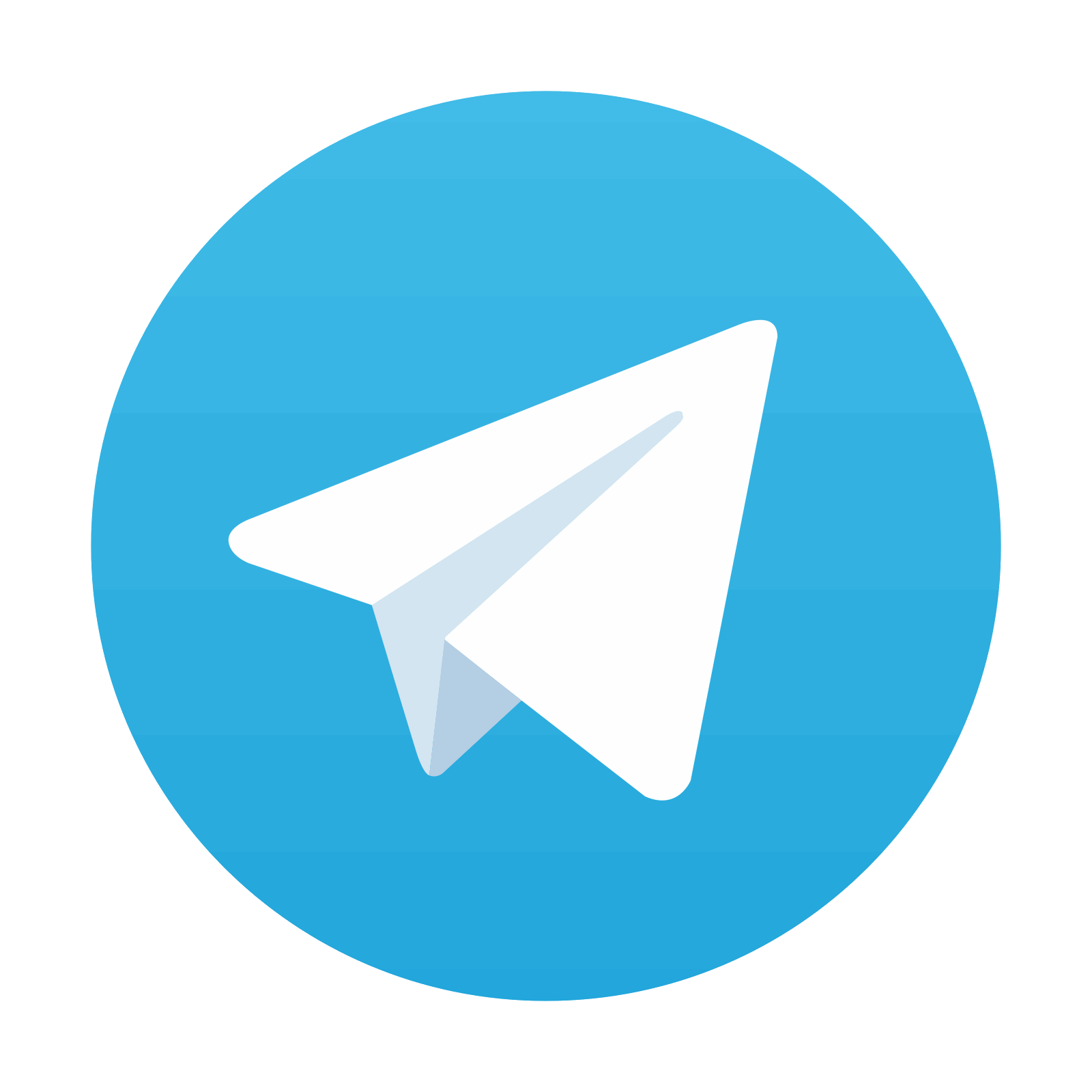
Stay updated, free articles. Join our Telegram channel

Full access? Get Clinical Tree
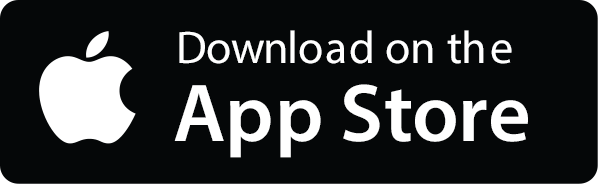
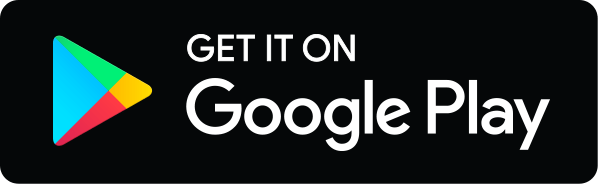
