Objectives
Upon completion of this chapter, the student should be able to answer the following questions:
- •
What is the physiologic importance of Ca ++ and inorganic phosphate (P i )?
- •
How does the body maintain Ca ++ and P i homeostasis?
- •
What roles do the kidneys, intestinal tract, and bone play in maintaining plasma Ca ++ and P i levels?
- •
What hormones and factors regulate plasma Ca ++ and P i levels?
- •
What are the cellular mechanisms responsible for Ca ++ and P i reabsorption along the nephron?
- •
What hormones regulate renal Ca ++ and P i excretion by the kidneys?
- •
What is the role of the calcium-sensing receptor?
- •
What are some of the more common clinical disorders of Ca ++ and P i homeostasis?
- •
What is the role of the kidneys in the production of calcitriol (active form of vitamin D)?
- •
What effects do loop and thiazide diuretics have on Ca ++ excretion?
Key Terms
Tetany
Hypoalbuminemia
Hyperalbuminemia
Hypocalcemia
Hypercalcemia
Parathyroid hormone (PTH)
Calcitriol
Vitamin D 3
Calcium-sensing receptor (CaSR)
Hypocalcemic tetany
Parathyroid hormone–related peptide
Thiazide diuretics
Claudin-16
Nephrolithiasis
Familial hypocalciuric hypercalcemia
Autosomal dominant hypoparathyroidism
Rickets
Osteomalacia
Na + -P i symporter
Chronic renal failure
Renal osteodystrophy
Addison disease
Fibroblast growth factor 23 (FGF-23)
PHEX
Tumor-induced osteomalacia
Klotho
Ca ++ and inorganic phosphate (P i ) a
a At physiologic pH, P i (inorganic phosphate) exists as <SPAN role=presentation tabIndex=0 id=MathJax-Element-1-Frame class=MathJax style="POSITION: relative" data-mathml='HPO4−2′>HPO−24HPO4−2
HPO 4 − 2
and <SPAN role=presentation tabIndex=0 id=MathJax-Element-2-Frame class=MathJax style="POSITION: relative" data-mathml='H2PO4−’>H2PO−4H2PO4−
H 2 PO 4 −
(pK = 6.8). For simplicity, we collectively refer to these ion species as P i .
In adults the kidneys play important roles in regulating total body Ca ++ and P i by excreting the amount of Ca ++ and P i that is absorbed by the intestinal tract (normal bone remodeling results in no net addition of Ca ++ and P i to the bone or Ca ++ and P i release from the bone). If the plasma concentrations of Ca ++ and P i decline substantially, intestinal absorption, bone resorption (i.e., the loss of Ca ++ and P i from bone), and renal tubular reabsorption increase and return plasma concentrations of Ca ++ and P i to normal levels. During growth and pregnancy, intestinal absorption exceeds urinary excretion, and these ions accumulate in newly formed fetal tissue and bone. In contrast, bone disease (e.g., osteoporosis) or a decline in lean body mass increases urinary Ca ++ and P i loss without a change in intestinal absorption. These conditions produce a net loss of Ca ++ and P i from the body. Finally, during chronic renal failure, P i accumulates in the body because absorption by the intestinal tract exceeds excretion in the urine. This situation can lead to the accumulation of P i in the body and changes in bone, including increased bone resorption with replacement by fibrous tissue, which renders bone more susceptible to fracture (see In The Clinic box on chronic renal failure on page 148).
This brief introduction reveals that the kidneys, in conjunction with the intestinal tract and bone, play a major role in maintaining plasma Ca ++ and P i levels as well as Ca ++ and P i balance. Accordingly, this chapter discusses Ca ++ and P i handling by the kidneys, with an emphasis on the hormones and factors that regulate urinary excretion.
Calcium
Cellular processes in which Ca ++ plays an important role include bone formation, cell division and growth, blood coagulation, hormone-response coupling, and electrical stimulus–response coupling (e.g., muscle contraction and neurotransmitter release). A total of 99% of Ca ++ is stored in bone and teeth, approximately 1% is found in the intracellular fluid (ICF), and 0.1% is in the extracellular fluid (ECF). The total Ca ++ concentration ([Ca ++ ]) in plasma is 10 mg/dL (2.5 mmol/L, or 5 mEq/L), and its concentration is normally maintained within very narrow limits. Approximately 50% of the Ca ++ in plasma is ionized, 40% is bound to plasma proteins (mainly albumin), and 10% is complexed to several anions, including P i , bicarbonate ( <SPAN role=presentation tabIndex=0 id=MathJax-Element-3-Frame class=MathJax style="POSITION: relative" data-mathml='HCO3−’>HCO−3HCO3−
HCO 3 −
), citrate, and <SPAN role=presentation tabIndex=0 id=MathJax-Element-4-Frame class=MathJax style="POSITION: relative" data-mathml='SO4=’>SO=4SO4=
SO 4 =
( Fig. 9.1 ). The pH of plasma influences this distribution ( Fig. 9.2 ). Acidemia increases the percentage of ionized Ca ++ at the expense of Ca ++ bound to proteins, whereas alkalemia decreases the percentage of ionized Ca ++ , again by altering the Ca ++ bound to proteins. Individuals with alkalemia are susceptible to tetany (tonic muscular spasms), whereas individuals with acidemia are less susceptible to tetany, even when total plasma Ca ++ levels are reduced. The increase in [H + ] in patients with metabolic acidosis causes more H + to bind to plasma proteins, P i , <SPAN role=presentation tabIndex=0 id=MathJax-Element-5-Frame class=MathJax style="POSITION: relative" data-mathml='HCO3-‘>HCO−3HCO3-
HCO 3 –
, citrate, and <SPAN role=presentation tabIndex=0 id=MathJax-Element-6-Frame class=MathJax style="POSITION: relative" data-mathml='SO4−’>SO−4SO4−
SO 4 −
, thereby displacing Ca ++ . This displacement increases the plasma concentration of ionized Ca ++ . In alkalemia the [H + ] of plasma decreases. Some H + ions dissociate from plasma proteins, P i , <SPAN role=presentation tabIndex=0 id=MathJax-Element-7-Frame class=MathJax style="POSITION: relative" data-mathml='SO4=’>SO=4SO4=
SO 4 =
, citrate, and <SPAN role=presentation tabIndex=0 id=MathJax-Element-8-Frame class=MathJax style="POSITION: relative" data-mathml='SO4=’>SO=4SO4=
SO 4 =
in exchange for Ca ++ , thereby decreasing the plasma concentration of ionized Ca ++ . In addition, the plasma albumin concentration also affects ionized plasma [Ca ++ ]. Hypoalbuminemia increases the ionized [Ca ++ ], whereas hyperalbuminemia decreases ionized plasma [Ca ++ ]. The total measured plasma [Ca ++ ] does not reflect the total ionized [Ca ++ ], which is the physiologic relevant measure of plasma [Ca ++ ]. A low ionized plasma [Ca ++ ] ( hypocalcemia ) increases the excitability of nerve and muscle cells and can lead to hypocalcemic tetany. Tetany associated with hypocalcemia occurs because hypocalcemia causes the threshold potential to shift to more negative values (i.e., closer to the resting membrane voltage; Fig. 9.3 ). An elevated ionized plasma [Ca ++ ] ( hypercalcemia ) may decrease neuromuscular excitability or produce cardiac arrhythmias, lethargy, disorientation, and even death. b
b In clinical practice the terms hypercalcemia and hypocalcemia are often used to describe a high or low total plasma [Ca ++ ], respectively, even though this usage is not the physiologically correct usage of hypercalcemia and hypocalcemia.
This effect of hypercalcemia occurs because an elevated plasma [Ca ++ ] causes the threshold potential to shift to less negative values (i.e., further from the resting membrane voltage). Plasma [Ca ++ ] is regulated within a very narrow range primarily by parathyroid hormone (PTH) ; calcitriol (1,25-dihydroxyvitamin D), the active metabolite of vitamin D 3 ; and plasma Ca ++ .


Within cells, Ca ++ is sequestered in the endoplasmic reticulum and mitochondria or it is bound to proteins. Thus the free intracellular [Ca ++ ] is very low (∼100 nmol). The large concentration gradient for [Ca ++ ] across cell membranes is maintained by a Ca ++ –adenosine triphosphatase (ATPase) (PMCA1b) in all cells and by a 3Na + -Ca ++ exchanger (NCX1) in some cells.
Overview of Ca ++ Homeostasis
Ca ++ homeostasis depends on two factors: (1) the total amount of Ca ++ in the body and (2) the distribution of Ca ++ between bone and the ECF. The total body Ca ++ level is determined by the relative amounts of Ca ++ absorbed by the intestinal tract and excreted by the kidneys ( Fig. 9.4 ). Importantly, regulation of Ca ++ excretion by the kidneys is one of the major ways that the body regulates ECF [Ca ++ ].

The intestinal tract absorbs Ca ++ through an active, carrier-mediated transport mechanism that is stimulated by calcitriol, the active metabolite of vitamin D 3 that is produced in the proximal tubule of the kidneys. Net Ca ++ absorption by the intestine is normally 200 mg/day (5 mmol/day), but it can increase to 600 mg/day (15 mmol/day) when calcitriol levels rise. In adults, Ca ++ excretion by the kidneys equals the amount absorbed by the gastrointestinal tract (200 mg/day), and it changes in parallel with intestinal absorption. Thus, in adults, Ca ++ balance is maintained because the amount of Ca ++ ingested in an average diet (1000 mg/day or 25 mmol/day) equals the amount lost in the feces (800 mg/day or 20 mmol/day), the amount that escapes absorption by the intestinal tract, plus the amount excreted in the urine (200 mg/day).
The second factor that controls Ca ++ homeostasis is the distribution of Ca ++ between bone and the ECF (see Fig. 9.4 ). Two hormones (PTH and calcitriol c
c Calcitonin is secreted by thyroid C cells (also known as parafollicular cells), and its secretion is stimulated by hypercalcemia. Calcitonin decreases the plasma [Ca ++ ] mainly by stimulating bone formation (i.e., deposition of Ca ++ in bone). Although calcitonin plays an important role in Ca ++ homeostasis in lower vertebrates, it plays only a minor role in Ca ++ homeostasis in humans and thus it will not be discussed further.
) regulate the distribution of Ca ++ between bone and the ECF and thereby, in concert with the kidneys, regulate the plasma [Ca ++ ]. PTH is secreted by the parathyroid glands, and its secretion is stimulated by a decline in the plasma [Ca ++ ] (i.e., hypocalcemia). Plasma Ca ++ is an agonist of the calcium-sensing receptor (CaSR) , which is in the plasma membrane of chief cells in parathyroid glands (see the discussion that follows). Hypercalcemia activates the CaSR, which decreases PTH release, whereas hypocalcemia reduces CaSR activity, which in turn increases PTH release. PTH increases the plasma [Ca ++ ] by (1) stimulating bone resorption, (2) increasing Ca ++ reabsorption by the distal tubule of the kidney, and (3) stimulating the production of calcitriol, which in turn increases Ca ++ absorption by the intestinal tract and facilitates PTH-mediated bone resorption.The production of calcitriol in the kidney is stimulated by hypocalcemia and hypophosphatemia. Calcitriol increases the plasma [Ca ++ ] primarily by stimulating Ca ++ absorption from the intestinal tract. It also facilitates the action of PTH on bone and enhances Ca ++ reabsorption in the kidneys by increasing the expression of key Ca ++ transport and binding proteins in the kidneys.
Hypercalcemia activates the CaSR in the thick ascending limb of Henle’s loop, inhibiting Ca ++ reabsorption in this segment, which results in an increase in urinary Ca ++ excretion and thereby reduces plasma [Ca ++ ]. Hypocalcemia has the opposite effect.
Conditions that lower PTH levels (e.g., hypoparathyroidism after parathyroidectomy for an adenoma) reduce plasma [Ca ++ ], which can cause hypocalcemic tetany (intermittent muscular contractions). In severe cases, hypocalcemic tetany can cause death by asphyxiation. Hypercalcemia also can cause lethal cardiac arrhythmias and decreased neuromuscular excitability. Clinically the most common causes of hypercalcemia are primary hyperparathyroidism and malignancy-associated hypercalcemia. Primary hyperparathyroidism results most often from the overproduction of PTH caused by a benign tumor of the parathyroid glands. In contrast, malignancy-associated hypercalcemia, which occurs in 10%–20% of all patients with cancer, is caused by the secretion of parathyroid hormone–related peptide, a PTH-like hormone secreted by carcinomas in various organs. Increased levels of PTH and parathyroid hormone–related peptide cause hypercalcemia and hypercalciuria.
Ca ++ Transport Along the Nephron
The Ca ++ available for glomerular filtration consists of the ionized fraction and the amount complexed with anions. Thus about 60% of the Ca ++ in the plasma is available for glomerular filtration. Normally, 99% of the filtered Ca ++ is reabsorbed by the nephron ( Fig. 9.5 ). The proximal tubule reabsorbs about 50% to 60% of the filtered Ca ++ . Another 15% is reabsorbed in the loop of Henle (mainly the cortical portion of the thick ascending limb), about 10% to 15% is reabsorbed by the distal tubule, and <1% is reabsorbed by the collecting duct. About 1% (200 mg/day) is excreted in the urine. This fraction is equal to the net amount absorbed daily by the intestinal tract.

Ca ++ reabsorption by the proximal tubule occurs primarily via the paracellular pathway (see Fig. 4.1 ). This passive, paracellular reabsorption of Ca ++ is driven by solvent drag, the lumen-positive transepithelial voltage across the second half of the proximal tubule, and by a favorable concentration gradient of Ca ++ , both of which are established by transcellular sodium and water reabsorption in the first half of the proximal tubule.
Ca ++ reabsorption by the loop of Henle also occurs primarily via the paracellular pathway. Like the proximal tubule, Ca ++ and Na + reabsorption in the thick ascending limb parallel each other. These processes are parallel because of the significant component of Ca ++ reabsorption that occurs via passive, paracellular reabsorption secondary to Na + reabsorption that generates a lumen-positive transepithelial voltage. Loop diuretics inhibit Na + reabsorption by the thick ascending limb of the loop of Henle and in so doing reduce the magnitude of the lumen-positive transepithelial voltage (see Chapter 10 ). This action in turn inhibits the reabsorption of Ca ++ via the paracellular pathway. Thus loop diuretics are used to increase renal Ca ++ excretion in patients with hypercalcemia.
In the distal tubule, where the voltage in the tubule lumen is electrically negative with respect to the blood, reabsorption of Ca ++ is entirely active because Ca ++ is reabsorbed against its electrochemical gradient ( Fig. 9.6 ). Thus Ca ++ reabsorption by the distal tubule is exclusively transcellular. Calcium enters the cell across the apical membrane by Ca ++ -permeable ion channels (TRPV5). Inside the cell, Ca ++ binds to calbindin-D28k. The calbindin-Ca ++ complex carries Ca ++ across the cell and delivers it to the basolateral membrane, where it is extruded from the cell primarily by the 3Na + -Ca ++ antiporter (NCX1); however, the Ca ++ -ATPase (PMCA1b) may also contribute. Urinary Na + and Ca ++ excretion usually change in parallel. However, the excretion of these ions does not always change in parallel, because the reabsorption of Ca ++ and Na + by the distal tubule is independent and is differentially regulated. For example, thiazide diuretics inhibit Na + reabsorption by the distal tubule and stimulate Ca ++ reabsorption by this segment. Accordingly, the net effects of thiazide diuretics are to increase urinary Na + excretion and reduce urinary Ca ++ excretion (see Chapter 10 ). Accordingly, because thiazide diuretics reduce urinary Ca ++ excretion, they often are given to reduce the urinary [Ca ++ ] in individuals who produce kidney stones that contain Ca ++ .
Mutations in the tight junction protein claudin-16 (CLDN16) reduce the permeability of the paracellular pathway to Ca ++ and Mg ++ and thereby reduce the diffusive, resorptive movement of Ca ++ and Mg ++ across tight junctions in the thick ascending limb of Henle’s loop. Familial hypomagnesemic hypercalciuria is caused by mutations in claudin-16 (previously termed paracellin 1 [PCLN1]), a component of the tight junctions in thick ascending limb cells. This disorder is characterized by enhanced excretion of Ca ++ and Mg ++ because of a decrease in the passive reabsorption of these ions across the paracellular pathway in the thick ascending limb. The mutation in the claudin-16 gene reduces the permeability of the paracellular pathway to Ca ++ and Mg ++ , thereby reducing passive, paracellular reabsorption of both ions. These individuals have high levels of Ca ++ in their urine, which leads to stone formation ( nephrolithiasis ).

Regulation of Urinary Ca ++ Excretion
Several hormones and factors influence urinary Ca ++ excretion ( Table 9.1 ). Of these PTH exerts the most powerful control on renal Ca ++ excretion, and it is the primary hormone responsible for maintaining Ca ++ homeostasis. Overall this hormone stimulates Ca ++ reabsorption by the kidneys (i.e., reduces Ca ++ excretion). Although PTH inhibits the reabsorption of NaCl and fluid, and therefore Ca ++ reabsorption by the proximal tubule, PTH stimulates Ca ++ reabsorption by the thick ascending limb of the loop of Henle and the distal tubule. Thus the net effect of PTH is to enhance renal Ca ++ reabsorption. Changes in the plasma [Ca ++ ] also regulate urinary Ca ++ excretion, with hypercalcemia increasing excretion and hypocalcemia decreasing excretion. Hypercalcemia increases urinary Ca ++ excretion by (1) reducing proximal tubule Ca ++ reabsorption (reduced paracellular reabsorption as a result of increased interstitial fluid [Ca ++ ]); (2) inhibiting Ca ++ reabsorption by the thick ascending limb of the loop of Henle via activation of the CaSR located in the basolateral membrane of these cells (NaCl reabsorption is decreased, thereby reducing the magnitude of the lumen-positive transepithelial voltage); and (3) suppressing Ca ++ reabsorption by the distal tubule by reducing PTH levels. As a result, urinary Ca ++ excretion increases. Hypocalcemia has the opposite effect on urinary Ca ++ excretion, primarily by increasing Ca ++ reabsorption by the proximal tubule and thick ascending limb. Calcitriol enhances Ca ++ reabsorption by the distal tubule, but it is less effective than PTH.
Factor/Hormone | Nephron Location | ||
---|---|---|---|
Proximal Tubule | TAL | Distal Tubule | |
PTH (PTHrP) ∗ | Decrease | Increase | Increase |
Calcitriol | Increase | ||
Volume expansion | Decrease | No change | Decrease |
Hypercalcemia | Decrease | Decrease (CaSR) | Decrease (via PTH) |
Hypocalcemia | Increase | Increase | |
Phosphate loading (hyperphosphatemia) | Increase (via PTH) | ||
Phosphate depletion (hypophosphatemia) | Decrease | Decrease (via PTH) | |
Acidemia | Decrease | ||
Alkalemia | Increase | ||
Loop diuretics | Decrease | ||
Thiazide diuretics | Increase |
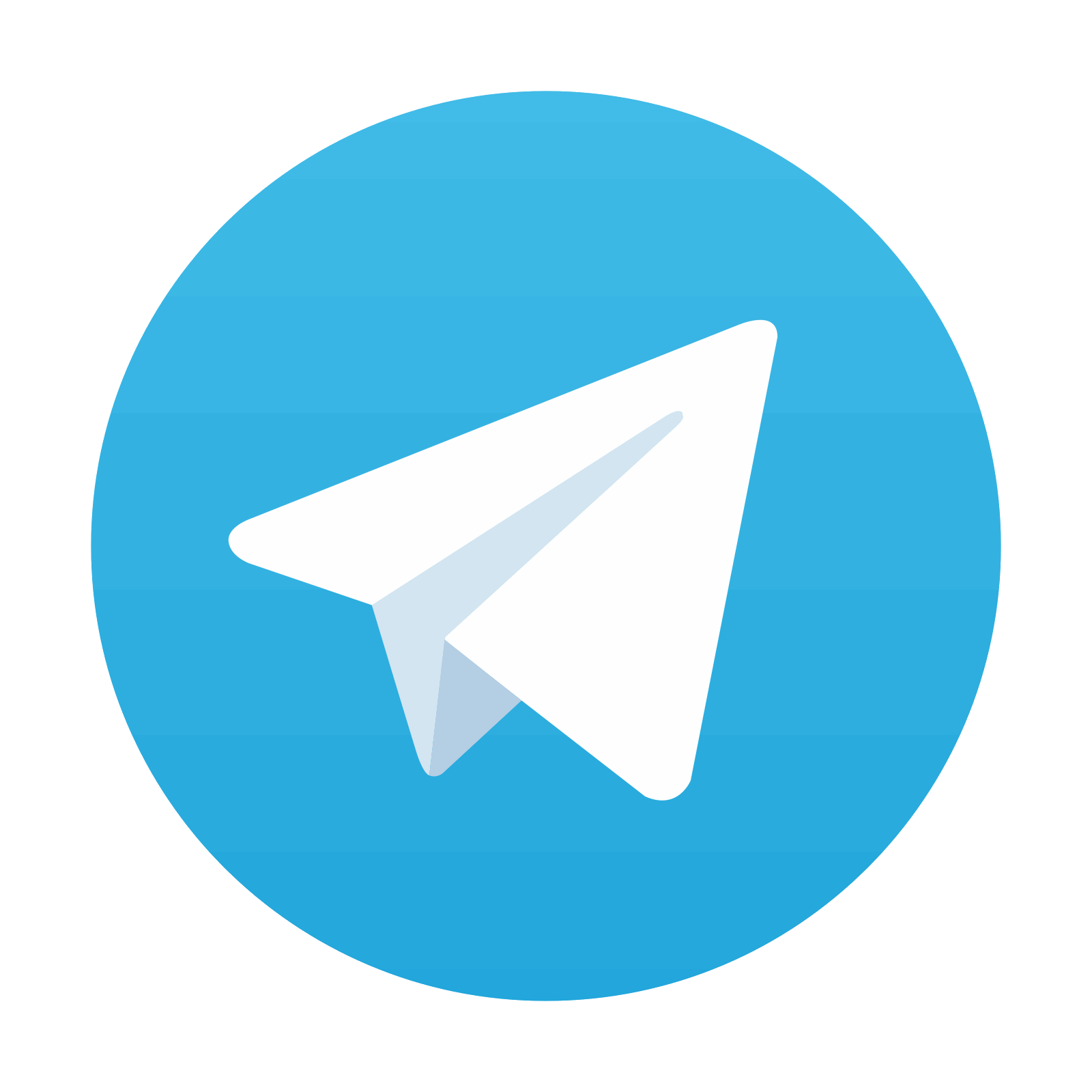
Stay updated, free articles. Join our Telegram channel

Full access? Get Clinical Tree
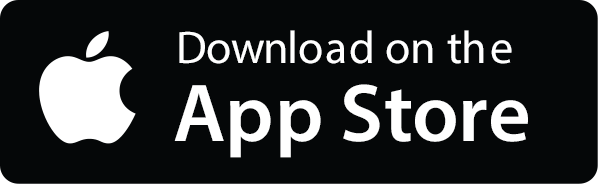
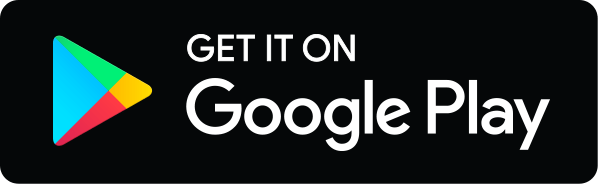
