There are an estimated 5.8 million people in the United States with heart failure.1
Stem cell therapy for the heart represents a novel method by which dysfunctional cardiac tissue might be repaired or replaced, thereby alleviating heart failure and decreasing or eliminating life-threatening arrhythmias.
Potential sources of stem cell therapy for the heart include the bone marrow, circulating progenitor cells, embryonic stem cells, and the heart itself.
Multiple modalities exist for delivery of stem cell therapy to the heart, including traditional and minimally invasive surgical methods as well as intravenous and percutaneous catheter-based techniques.
The heart was once thought to be a terminally differentiated organ, with no cell turnover or repair; the cardiac myocytes at the time of early adulthood were all the cells a person would ever have. It was for this reason that myocardial infarction (MI) was thought to be such a devastating event, as there was no native ability to repair or replace damaged heart tissue. This understanding began to change in the 1990s, when investigators first reported the presence of cardiac myocytes in various stages of cell division, found during histologic analysis of myocardial tissue.2 Since that time it was learned that the heart does have the capacity for cellular repair, both from stem cells resident in the heart, and from so-called “circulating” progenitor cells, which, following release from the bone marrow, may traffic to and enter heart tissue to effect repair.3–5 This endogenous repair system appears to have the capacity to handle routine cellular turnover, that is, normal “wear and tear” on the heart, but becomes overwhelmed in the setting of a sudden large insult, such as a MI, with the default fibroblast scar formation. With the discovery of the capacity for cellular repair of the heart, interest in augmenting or optimizing this system to the point that the damaged and failing heart could be biologically repaired has grown. For example, although the small number of resident cardiac stem cells (CSCs) might be insufficient to repair the damage of a large infarction, removal and expansion of these cells in ex vivo tissue culture might generate sufficient numbers of cells to result in a therapeutic benefit.
The interest in cell therapy is driven primarily by the nearly 6 million Americans with heart failure,1 as well as millions of others throughout the world, many of whom, despite optimal medical therapy, suffer significant morbidity and mortality from their condition. In many cases, temporary mechanical support or heart transplantation is necessary, but supply constraints and large expense limit availability. Thus, the great hope for cellular therapy for the heart is that end-stage heart failure could be eliminated by preventing or reversing the deleterious remodeling that occur following significant injury to the heart. Although such benefits are reported in animal models, clinical cell therapy for the heart remains in its infancy, and numerous questions remain regarding which cell type (or types) might be most effective at cardiac repair, when and how those cells should be delivered, and finally, the mechanisms by which the cells achieve any benefit. Below, the current state of cell therapy for the heart, focusing on results and lessons learned from those clinical studies reported to date, will be discussed, couched in the understanding that this field at present is young and rapidly changing.
To date, numerous different types of stem cells have been studied in clinical trials of cellular therapy for the heart. Here we discuss these different cell types, their potential advantages and disadvantages, and the clinical results to date (see also Table 53-1).
Stem Cell Type | Advantages | Potential Disadvantages |
---|---|---|
Bone marrow cells (BMCs) |
|
|
Mesenchymal stem cells (MSCs) |
| Large cell diameter may limit certain delivery methods (e.g., intracoronary infusion) |
Circulating progenitor cells (CPCs) |
|
|
Cardiac stem cells (CSCs) |
|
|
Skeletal myoblasts |
| Associated with malignant arrhythmias in MAGIC Trial |
Embryonic stem cells | Unlimited differentiation potential |
|
Perhaps the best characterized and most widely studied form of cell therapy for the heart is that using stem cells isolated from the bone marrow. In addition to serving as hematologic precursors, certain populations of bone marrow cells (BMCs) are released into the blood in response to signals from injured tissue, and subsequently traffic to and enter injured tissues or organs to assist in repair.6 BMCs were first shown to be capable of cardiac repair in 2001, in experiments in which cells were injected at the infarct border zone of small animals.7 A portion of these cells engrafted and subsequently differentiated into cardiac tissue, forming myocytes, smooth muscle, and vascular cells. Little more than a year later, in late 2002, the first clinical trial of stem cell therapy for the heart was reported, when the results of the TOPCARE-AMI trial were published.8 In this study, patients who had suffered a recent MI were randomized to receive intracoronary infusion of BMCs, circulating progenitor cells (CPCs), or to serve as a control. The results were promising, in that those patients who received either cell type had significantly improved left ventricular ejection fraction (LVEF) 4 months later compared with controls (results discussed in detail below).
Since the publication of TOPCARE-AMI, more than 800 patients have been enrolled in randomized control studies of BMC therapy for the heart, and generally these trials show a modest benefit.9–27 In a meta-analysis of the trials published as of 2007, BMC therapy after MI resulted in modest, but statistically significant improvements in measures of left ventricular (LV) function and remodeling compared with placebo (see Fig. 53-1).9 For example, patients receiving cell therapy had a 3.64 percent greater improvement in LVEF, compared with placebo (95 percent CI: 1.56 to 5.73). Similar modest, but significant relative benefits were seen in terms of infarct size and change in LV end systolic volume (ESV) across studies, as well as modest, but nonsignificant differences in end-diastolic volume. Perhaps most importantly, across studies BMC therapy was safe, suggesting that the modest beneficial effects did not come at a safety cost. These results have led to the question of how the beneficial effects of BMC therapy might be further augmented and optimized. Closer examination of specific BMC clinical studies led to certain conclusions that will guide future studies.
Figure 53-1
Forest plot of unadjusted difference in mean change in left ventricular ejection fraction [with 95 percent confidence intervals (CIs)] in patients treated with bone marrow–derived cells (BMCs) compared with controls from multiple randomized control trials (RCTs) and cohort studies. The overall effect was statistically significant in favor of BMC therapy. (Adapted with permission from Abdel-Latif A, Bolli R, Tleyjeh IM, et al. Adult BMCs for cardiac repair: A systematic review and meta-analysis. Arch Intern Med 2007 May 28;167:989–997. Copyright © (2007) American Medical Association. All rights reserved.)

Of the studies of BMC therapy for the heart to date, the results of the Reinfusion of Enriched Progenitor Cells and Infarct Remodeling in Acute Myocardial Infarction (REPAIR-AMI) trial, published in 2006, are perhaps the most informative.10 In this study, patients suffering an ST-elevation MI were randomized to receive autologous BMCs or placebo (carrier solution only) by intracoronary infusion in the infarct-related artery. A total of 204 patients were enrolled (101 receiving cells, 103 receiving placebo), and were followed for 1 year. As with the overall findings for BMCs, the effect of cell infusion on cardiac function in this study was modest, but significant, with the LVEF at 3 months in those who received cell therapy significantly greater than in those who received placebo (53.8 vs. 49.9 percent, p = 0.02). Cell-treated patients also had a significantly greater absolute increase in LVEF over 3 months (5.5 vs. 3.0 percent, p = 0.01). Although the effects on systolic function were also modest, they were associated with significant clinical benefits at 1 year. There were two deaths, no infarctions, and 21 revascularizations in the BMC group and six deaths, five infarctions, and 35 revascularizations in the placebo group, for a significantly lower rate of the combined outcomes in the BMC group (p = 0.01).
This study was particularly informative, however, when the results were further scrutinized. First, the patients who suffered the largest infarcts (as evidenced by the most severe LV dysfunction) benefited the most from cell therapy. When patients were stratified by their LVEF prior to treatment to above or below the median (48.9 percent), those patients with an EF below the median who received BMCs had a mean absolute increase in LVEF of 7.5 percent compared with only 2.5 percent in those receiving placebo (p = 0.002). However, in those patients whose baseline LVEF was above the median, cell therapy resulted in a mean absolute increase of only 4.0 percent, nearly identical to the 3.7 percent increase in such patients treated with placebo (p = 0.81; see Fig. 53-2a). These results indicate that the overall benefit seen in the study was driven primarily by those with a baseline LVEF below the median, and this finding is not surprising, as those patients with an LVEF greater than the median had essentially normal systolic function (~55 percent in adults), suggesting that the damage suffered from their infarction was limited. This suggests that those patients with significant damage and dysfunction are the ones who stand to benefit the most from cellular therapy and should be targeted for future clinical study.
Figure 53-2
Effect of baseline left ventricular ejection fraction (LVEF) and timing of intracoronary infusion of BMCs on absolute change in LVEF from the REPAIR-AMI Trial. Panel A shows that in those patients whose baseline LVEF was below the median (<48.9 percent), BMC treatment resulted in a significant increase in LVEF compared with placebo (left side of panel A). In contrast, no significant difference was seen between the treatment groups in those patients with a baseline LVEF above the median (right side of panel A). Panel B shows the effect timing of intracoronary infusion had on the relative effect of BMC therapy compared with placebo. When given <3 days after MI, there was no difference in absolute change in LVEF between BMC, and placebo-treated patients; however, the further from the time of MI, the greater the relative benefit of BMC therapy (p = 0.01 for interaction). (Reproduced with permission from Schachinger V, Erbs S, Elsasser A, et al. Intracoronary bone marrow-derived progenitor cells in acute myocardial infarction. N Engl J Med 2006 Sep 21;355(12):1210–1221. Copyright© (2006) Massachusetts Medical Society. All rights reserved.)

The REPAIR-AMI study was also informative in that the timing of cell delivery proved important, and the findings were contrary to what might be expected. While many believed that earlier delivery would be better, in fact the opposite proved true. In the study, patients received treatment between 3 and 7 days after their infarction. This variability resulted from differences in the time required for cell processing and other scheduling logistics, and was not a prespecified point of investigation. However, when patients were stratified by the day after infarction that they received their infusion, there was a direct relationship between time and absolute increase in LVEF, with those receiving BMCs later having greater improvement in systolic function, and the greatest difference compared to time-matched controls (see Fig. 53-2b). This would suggest that delivering cells to an area of new infarction, which is inflamed and filled with large numbers of dead or soon-to-be-dying myocytes, is delivering them to a hostile environment, which likely reduces BMC survival and engraftment, thereby limiting their effectiveness. It remains to be seen what the optimal time is for cell delivery to the heart after acute MI, as there is likely a point after which there are diminishing returns, but REPAIR-AMI has certainly indicated that early delivery is not optimal.
The TOPCARE-CHD Trial of BMC therapy in chronic LV dysfunction was published11 at the same time as REPAIR-AMI. There are many patients who develop ischemic cardiomyopathy well after their infarction, or have unrecognized or “silent” MIs, and only present sometime later with heart failure. In many ways such patients are those who stand to benefit most from cell therapy, as when their cardiomyopathy is discovered, they often receive limited benefit from revascularization. In TOPCARE-CHD, 75 patients who suffered a MI at least 3 months prior to enrollment, with a well-demarcated area of LV dysfunction, and a patent infarct-related artery, were randomized to receive intracoronary infusion of BMCs, CPCs, or to serve as a control (no cell infusion). The cells were delivered via intracoronary infusion in the infarct-related artery. After 3 months, there was a cross-over phase in which all patients received a second infusion of cells. Patients receiving cells at the first infusion received the other cell type at the second infusion, and placebo patients were randomized to one of the two cell types. The subjects were then followed for three additional months.
The primary outcome of the TOPCARE-CHD study was absolute change in LVEF. Patients receiving BMCs had a modest but significant increase in LVEF (+2.9 percent, p = 0.001 compared with baseline), while CPC treated (−0.4 percent, p = 0.60), and control patients (−1.2 percent, p = 0.12) did not. The significant increase in LVEF following administration of BMCs was present regardless of whether they were given as the initial treatment, or following administration of CPCs. There was also a trend toward lower adverse clinical outcomes 3 months after initial treatment in patients receiving BMCs. Using the combined end point of death, MI, stroke, rehospitalization for heart failure, or ventricular tachycardia, no patients treated with BMCs experienced these events, compared with five patients in the CPC group (15 percent), and three in the control group (13 percent; p = 0.07 across groups). These results suggest that patients with remote prior MI can still benefit from cell therapy, from both LV function and clinical standpoints. This benefit, however, appears limited to BMCs, as CPCs did not produce the same results. Although these results would indicate that not all stem cells have equal benefit, it should be interpreted cautiously as the mean dose of BMCs in this study was 205 million cells, while that for CPCs was only 22 million. The lack of effect seen with CPCs in this study may therefore be a function of cell dose rather than type.
Together the results from the BMC clinical trials to date support the promise of stem cell therapy for the heart, though admittedly the modest functional benefits have tempered clinical enthusiasm. There is the hope that the beneficial effects of BMCs can be improved upon in the future as parameters of patient selection, delivery timing, and dosing are further understood and optimized. Although the answers to these questions will require further clinical studies, the excellent safety profile of BMC cell therapy to date suggests that from an ethical standpoint, these can be pursued.
Mesenchymal stem cells (MSCs) are a subpopulation of the bone marrow, which are involved with repair of tissues of mesenchymal origin, including muscle, bone, and adipose tissue.28,29 They are multipotent and directable in their ultimate fate, so they have been used experimentally to treat not only bone and connective tissue disorders, but heart disease as well. In addition, they have potent immunomodulatory effects resulting from their ability to alter T-cell activation, thereby limiting inflammation and cell killing. Most importantly for cell therapy of the heart, the immunomodulatory effects of MSCs mean that they are relatively immune-privileged, and do not stimulate a typical rejection response when delivered to an allogeneic recipient.30 This has led to the concept that MSCs may be an ideal cell for early treatment of tissue injury, as allogeneic MSCs could potentially be stored like a drug, ready to be delivered at any time, “off-the-shelf.”
The ability of MSCs to take on a cardiac phenotype when delivered to the heart was first reported by Toma and colleagues in 2002, and led to further trials of MSCs for “cellular cardiomyoplasty.”31 In this study, human MSCs, delivered by direct injection to the normal (noninfarcted) hearts of adult immunodeficient mice, engrafted, and over time came to resemble cardiac myocytes, both morphologically and by surface marker expression. This finding was followed quickly by multiple studies in large animal models. Perhaps most relevant to the content at hand are the results reported by Shake et al. later the same year. They reported that when autologous MSCs were delivered by direct surgical injection to the hearts of pigs 2 weeks after creation of an infarction, the cells engrafted and significantly attenuated the wall thinning and regional dysfunction typically seen after an infarction, as compared with control animals.32 These findings were supported by multiple subsequent studies of MSC treatment in large animal models of MI and ischemic cardiomyopathy using various cell delivery modalities, including catheter-based endocardial injection.33–36
The first clinical trials of MSCs in the heart, like those involving BMCs, were performed using intracoronary infusion after MI. These studies by Chen et al.13 and Katritsis et al.19 were small (and in the latter only case-controlled), but showed promising effects in terms of attenuation of LV remodeling and preservation of cardiac function. Neither study reported complications related to intracoronary MSC infusion, which contrasted with multiple animal studies, which reported that such delivery can be complicated by MI due to vascular congestion.37–39 This may reflect a difference between the coronary vascular capacitance of human and large animal models, though safety concerns have limited further trials using intracoronary infusion to deliver MSCs.
Perhaps one of the more important studies of MSC therapy to date is the Safety Study of Adult Mesenchymal Stem Cells to Treat Myocardial Infarction study, the results of which were published in 2008.40 In this double-blind placebo-controlled study, patients within 10 days of a first ST-elevation MI were randomized to receive systemic intravenous infusion of allogeneic MSCs or placebo. Overall there was no significant improvement or difference in LVEF in those patients treated with MSCs compared with placebo, but in the subset of patients who had suffered an anterior MI, MSC infusion resulted in significant improvement in LV ejection fraction at 6 months (+7.3 ± 3.4 percent, p = 0.044, n = 12), while placebo treatment did not (3.4 ± 3.4 percent, p = NS, n = 8). Anterior MIs are those most likely to result in significant LV dysfunction, and thus the results of this study are similar to those of REPAIR-AMI, in that those patients with more LV dysfunction experienced greater benefit from BMC therapy.10 In addition, patients receiving MSCs had significantly fewer arrhythmic events over the 6-month follow-up period: only one patient in the MSC group (2.9 percent) had nonsustained ventricular tachycardia as compared with five patients who received placebo (26.3 percent; p = 0.018). Arrhythmic events after MI (and in heart failure in general) are a primary cause of morbidity and mortality, and therefore the antiarrhythmic effect of MSC treatment seen in this study is important. Furthermore, one of the potential concerns about cell therapy for the heart is that delivered cells could be a focus for arrhythmia,41 thereby negating any functional benefit. Indeed, in the Myoblast Autologous Grafting in Ischemic Cardiomyopathy (MAGIC) trial of intramyocardial injection of skeletal myoblasts for treatment of LV dysfunction (discussed below), cell therapy proved proarrhythmic.42
In summary, based on the results of studies done to date, the use of MSCs to treat cardiac dysfunction and repair the heart after infarction is being actively pursued for treatment of both acute and chronic ischemic disease, as well as for dilated (nonischemic) cardiomyopathy. Numerous studies are currently underway to explore the best delivery method, and whether there is an efficacy difference between autologous and allogenic MSCs. If allogenic MSCs are as effective as autologous cells, their use would eliminate the need to isolate and expand cells from each patient, and potentially make cell therapy for the heart more practical and cost-effective.
First described by Asahara et al. in 1997,43 CPCs, or endothelial progenitor cells (EPCs), as they are sometimes called, are a subpopulation of BMCs that can be found in the blood and express surface markers typical of both hematopoietic and endothelial cells.44 They are able to differentiate into mature endothelial cells, and contribute to vascular repair and creation of new blood vessels. They are stimulated by, and their concentration in the blood increases in response to, vascular injury or inflammation. Furthermore, their relative abundance in the blood is a predictor of vascular function and health and has been correlated with cardiac risk factor prediction models such as the Framingham Score.45
The ability of CPCs to contribute to neoangiogensis and vascular repair makes them a logical choice for the treatment of MI and ischemic cardiomyopathy, and to date CPCs have been studied in multiple clinical trials with mixed results. The potential beneficial effects of these cells were seen in the TOPCARE-AMI study. Twenty patients suffering an ST-elevation MI were randomized to receive intracoronary infusion of autologous BMCs (n = 9), or CPCs (n = 11).8 Treatment with either cell type resulted in an increase in LVEF and a significant reduction in ESV, a marker of adverse remodeling, compared with baseline at 4 months after treatment. In the CPC group patients had an average absolute increase in LVEF of 8.2 percent (51.3–59.5 percent), and decrease in ESV of 8.0 mL (56.9–48.9 mL). These results were nearly identical to those seen in the BMC group, and when all patients receiving cell therapy were pooled, the changes in LVEF and ESV from baseline to 4 months were significant (p = 0.003 and 0.011, respectively). There was no placebo group in this study, but LVEF and ESV in case-matched control patients (n = 11) who were enrolled and followed in the study did not significantly change. At 4 months, patients receiving cell therapy (CPC and BMC pooled) had a significantly greater mean LVEF compared with control patients (60.1 vs 53.5 percent, p < 0.05), and a significantly different change in ESV (−13.8 mL vs + 7.8 mL, p <0.02).
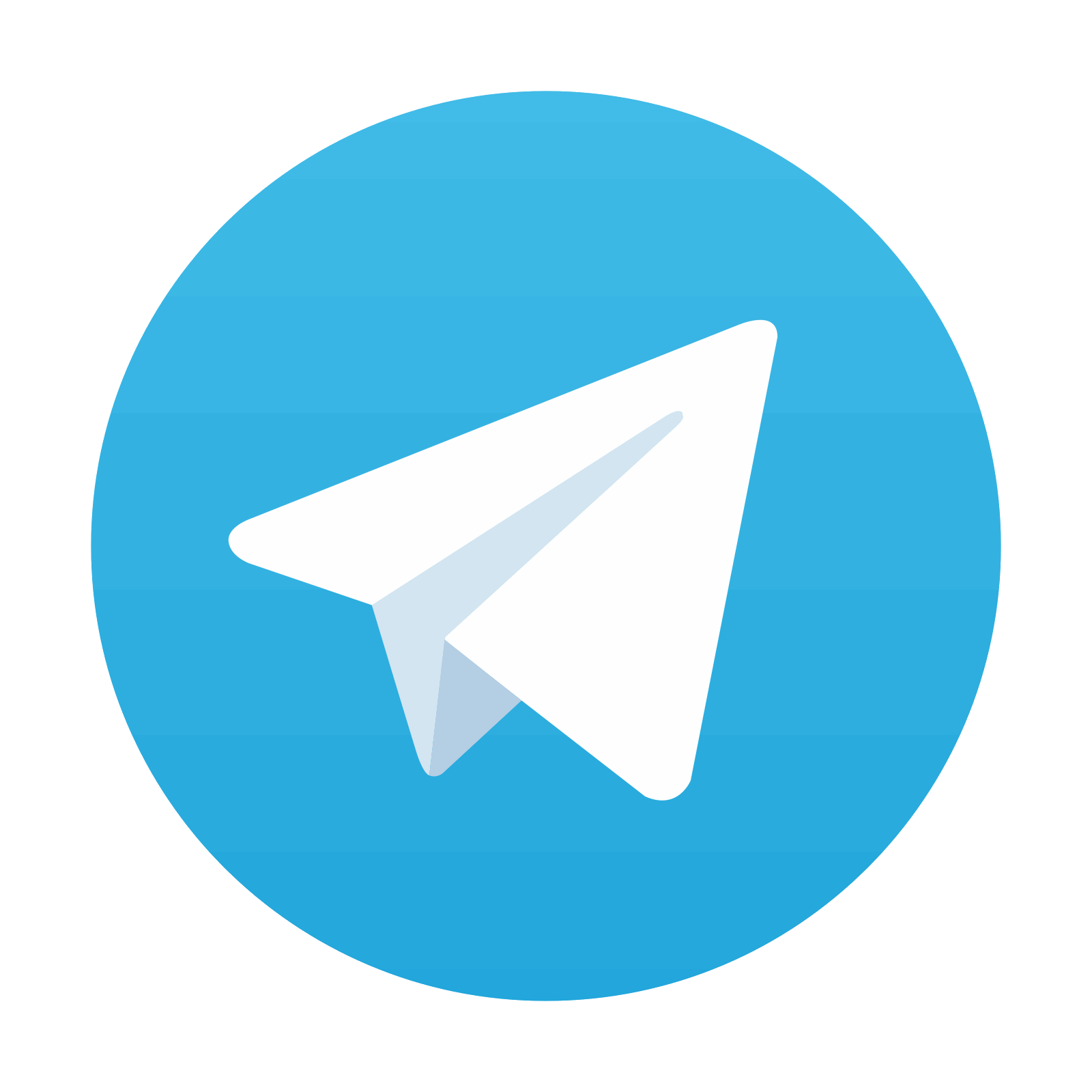
Stay updated, free articles. Join our Telegram channel

Full access? Get Clinical Tree
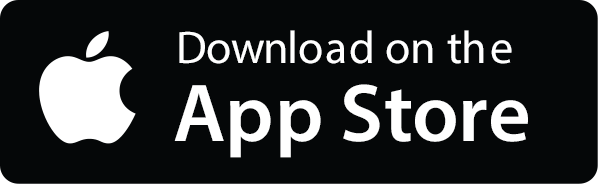
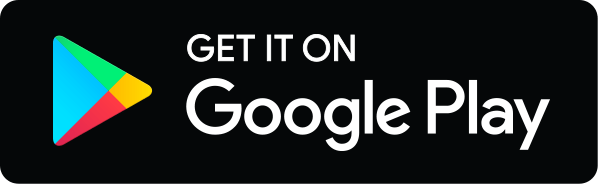