Outline
Introduction 233
Stem Cells in the Adult Kidney 233
Ontogenesis of the Renal Stem Cell Niche 235
The Adult Renal Stem Cell Niche 236
The Renal Niche in Aging 238
The Renal Niche in Glomerular Disorders 239
Conclusion 241
The kidney is classically regarded as an organ incapable of true regeneration, since no new nephrons appear after birth in humans. However, the kidney has the potential to regenerate functional tissues following tubular and even glomerular injury. In most adult epithelia replacement of damaged or dead cells is maintained through the presence of stem and progenitor cells. Accordingly, a large body of evidence has recently shown that the parietal epithelium of Bowman’s capsule represents a reservoir of renal stem cells in adult kidney which can generate novel podocytes and also proximal tubular cells. The discovery of a stem cell niche within the adult glomerulus provides a new point of view for the understanding of renal physiology. Indeed, renal stem cells can generate novel podocytes during kidney growth and following injury, thus explaining why glomerular disorders can undergo remission. However, recent evidence also suggests that the regenerative process can sometimes be inadequate because of an inefficient proliferative response of renal stem cells, as may happen in aging patients or during focal segmental glomerulosclerosis. Moreover, an excessive proliferative response of renal stem cells following podocyte injury initiates extracapillary lesions in crescentic glomerulonephritis or collapsing glomerulopathy and is involved in the generation of the tip lesion.
Introduction
Stem cells are one of the fundamental underpinnings of tissue biology . They allow adult tissues to be replenished by fresh cells throughout life. Additional stem cells lie dormant, but can be activated at particular life-cycle stages, or following injury. These potent agents are controlled within restricted tissue microenvironments known as “niches” . Accurately identifying stem cells in vivo remains the biggest obstacle to progress in understanding stem cell biology. Normal stem cells within tissues can rarely be identified by means of histological methods . Some properties that have been widely assumed to mark stem cells, such as preferential bromodeoxyuridine (BrdU) label retention (caused by an expected tendency of stem cells to divide more slowly than many of their progeny) have frequently proven to be unreliable where definitive independent markers are available . However, three functional properties characterize stem cells obtained from different tissues: (i) the ability to self-renew, which is a prerequisite for sustaining the stem cell pool; (ii) the ability to generate at the single cell level differentiated progeny cells, in general of multiple lineages; and (iii) the ability to functionally reconstitute a given tissue in vivo . The typical adult stem cell, present in many tissues, is considered multipotent as it can only give rise to differentiated cell types from the tissue of origin, in contrast to embryonic stem cells, which can give rise to cells from the three somatic germ layers (ectoderm, mesoderm and endoderm), as well as to germ cells, and are thus pluripotent .
Stem Cells in the Adult Kidney
The kidney is one of the few organs that undergo mesenchymal–epithelial transition during their development . Structures present in the adult kidney arise from reciprocal interactions between two discrete embryonic appendages: the ureteric bud and metanephric mesenchyme . The adult kidney contains more than 24 mature cell types arranged in distinct vascular, interstitial, glomerular and tubular compartments . This unique organogenesis and structural complexity of the adult kidney has been the cause of many challenges to the identification and characterization of kidney stem cells . In addition, the kidney has been regarded as an organ incapable of regeneration, since no new nephrons appear after 36 weeks of gestation in humans as a result of the exhaustion of progenitor mesenchyme . This does not rule out the existence of a renal stem cell able to elicit repair through replacement of postnatal renal cell types as opposed to formation of new nephrons . Indeed, the mammalian kidney shares with the majority of organs the ability to repopulate and at least partially repair structures that have sustained some degree of injury . Tubular integrity can be rescued after acute damage, and even severe glomerular disorders sometimes may undergo regression and remission, suggesting that glomerular injury is also reparable . For all these reasons, the existence of renal stem cells has been a matter of long debate.
Attempts to identify adult kidney stem cells were made on the basis of the broad principles of stem cell biology, such as prolonged cell-cycling time (label-retaining cells), ability to extrude Hoechst dye (side population cells), by restrictive cell culture conditions, or by using markers expressed by other stem cells or developing kidney . Some of these studies suggested the existence of putative interstitial renal stem cells . In particular, Oliver et al. identified a population of BrdU-label-retaining cells within the interstitium of renal papilla in the rat kidney. However, as already reported, the use of BrdU labeling does not seem to be a specific method for identification of stem cells . In addition, this hypothesis has recently been questioned by a study by Humphreys et al., who have developed a method for distinguishing the source of kidney tubular regeneration based on studies in transgenic rodents for the homeodomain transcriptional regulator Six2 . In this model, the Six2 promoter drives a fusion protein of green fluorescent protein (GFP) and Cre recombinase, which is expressed transiently in renal epithelial precursors during the developmental period of active nephrogenesis . GFPCre expression is not present in the adult, and this expression is not observed after injury. When Six2-GFPCre mice are crossed with a floxed STOP reporter strain, Cre-dependent removal of the stop sequence in progeny leads to constitutive and heritable expression of a marker gene so that all mesenchyme-derived renal epithelial cells, from Bowman’s capsule to the junction of the connecting segment and collecting duct, are heritably labeled . In contrast, the entire interstitial compartment is unlabeled . Thus, the maintenance of labeled tubules postinjury supports a model of epithelial tubule repair due to surviving tubular epithelial cells or stem/progenitor cells localized within the labeled nephron. In addition, in an update of their previous work, Oliver et al. demonstrated that following ischemic injury, label-retaining cells of the papilla migrate in the interstitium and to a lesser extent in collecting ducts, but only occasionally within the cortex. Finally, more recent results suggest that label-retaining cells of the papilla represent a population of cells that exits the cell cycle during embryonic development because it differentiates early . Taken together, these findings indicate that papillary interstitial cells or other types of interstitial stem/progenitor cells do not directly contribute to regeneration of glomerular or tubular epithelial cells, while their contribution to collecting ducts or interstitial cells regeneration remains to be established . These observations also indicate that repair of injured renal epithelium is predominantly accomplished by intrinsic, surviving tubular epithelial cells or a subset of stem/progenitor cells localized within the nephron .
However, converging data definitively demonstrated the existence of a population of stem/progenitor cells within the Bowman’s capsule of adult human kidney . These renal stem cells were identified through the assessment of the presence of both CD24, a surface molecule that has been used to identify different types of human stem cells , and CD133, a marker of several types of adult tissue stem cells . The results showed that both markers were coexpressed by a subset of parietal epithelial cells selectively localized at the urinary pole of Bowman’s capsule . Once isolated, CD24 + CD133 + renal stem cells were found to lack lineage-specific markers, to express transcription factors that are characteristic of multipotent stem cells, and to exhibit self-renewal, high clonogenic efficiency and multidifferentiation potential . When injected intravenously in severe combined immunodeficiency (SCID) mice that had acute kidney injury, CD24 + CD133 + renal stem cells regenerated tubular structures from different portions of the nephron and also reduced the morphological and functional kidney damage . The identification of CD24 + CD133 + renal stem cells has been confirmed by results obtained in transgenic mice, which suggest that endogenous cells of the nephron are responsible for repair of injured tubular epithelium , and leads to the hypothesis that, from the urinary pole of Bowman’s capsule, CD24 + CD133 + renal stem cells may initiate the replacement and regeneration of glomerular, as well as tubular epithelial cells in adult human kidney ( Fig. 15.1 ).

Ontogenesis of the Renal Stem Cell Niche
Regenerative biology draws on the understanding of normal developmental processes. It is generally believed that adult stem/progenitor cells directly derive from the organ-specific embryonic progenitor that is involved in organogenesis during fetal life . Nephrons, the basic functional units of the kidney, are generated repetitively during kidney organogenesis from a mesenchymal stem cell population ( Fig. 15.1 ). Development of the mature mammalian kidney results from reciprocal signaling between the branching ureteric bud tips and the undifferentiated metanephric mesenchyme, which leads to the aggregation and condensation of renal epithelial stem cells to form the renal vesicle, which then undergoes transformation in S-shaped body . At this stage, the proximal end of S-shaped body becomes invaded by blood vessels, differentiates into podocytes and parietal epithelial cells, and then generates glomeruli. Simultaneously, the middle and the distal segments of the S-shaped body begin to express proteins that are characteristic of tubular epithelia .
The existence of renal stem cells in the metanephric mesenchyme is supported by the observation that some metanephric mesenchyme-derived cells display multidifferentiation potential . In embryonic kidneys, coexpression of CD133 and CD24 characterized a subset of cells in the cap mesenchyme, renal vesicles and S-shaped bodies that displayed self-renewal and multidifferentiation potential ( Fig. 15.1 ). Thus, to track down embryonic renal stem cells, the expression of CD133 and CD24 during kidney development was evaluated. During nephron development, coexpression of CD133 and CD24 remained selectively localized to cells of the urinary pole of Bowman’s capsule . When injected into mice with acute renal failure, CD24 + CD133 + renal embryonic stem cells regenerated cells of different portions of the nephron, reduced tissue necrosis and fibrosis, and significantly improved renal function . In agreement with their nature, CD24 + CD133 + renal embryonic stem cells also expressed high levels of the stem cell-related transcription factor BmI-1, which is a critical regulator of self-renewal in different types of stem cells . The existence of a putative metanephric mesenchyme cell with stem cell properties had already been suggested by studies performed by the group of Reisner, who demonstrated that functioning renal tissue can be reconstituted by metanephric mesenchyme derived from kidneys of 8 weeks of gestation . Indeed, fetal kidney tissue obtained from 10 to 14 weeks of gestation maintained the property to generate de novo functional nephrons, but generated a smaller number of mature glomeruli and tubuli than kidneys of 8 weeks of gestation . Accordingly, CD24 + CD133 + renal embryonic stem cells are enriched in the kidneys of 8–9 weeks of gestation, substantially decrease during 10–14 weeks of gestation, and represent < 2% of whole renal cells in adults. In both fetal and adult kidney, CD24 + CD133 + stem cells persist as parietal epithelial cells localized at the urinary pole of Bowman’s capsule, supporting the concept that CD24 + CD133 + renal embryonic stem cells directly derive from renal embryonic stem cells and the urinary pole of Bowman’s capsule represents a stem cell niche, which is a specific site in adult tissues where stem cells reside . In agreement with this hypothesis, blastocyst-derived mouse embryonic stem cells once differentiated towards renal tubular cells selectively migrated to the tubuloglomerular junction following their injection into developing kidneys .
More recently, studies performed in transgenic rodents for the homeodomain transcriptional regulator Six2 have confirmed the existence in the cap mesenchyme of a multipotent nephron progenitor population . Indeed, Six2 -expressing cells give rise to all cell types of the main body of the nephron during all stages of nephrogenesis . Pulse labeling of Six2 -expressing nephron progenitors at the onset of kidney development suggests that the Six2 -expressing population is maintained by self-renewal and is multipotent, generating the multiple domains of the whole cortical nephron . Notably, podocytes, proximal and distal tubule structures were all descendants of a Six2 + cell, further confirming that a single multipotent progenitor is the source of both the glomerular and tubular epithelial cells that constitute the adult nephron .
The Adult Renal Stem Cell Niche
Stem cells are functionally defined by their ability to self-renew and to differentiate into the cell lineages of their tissue of origin . The ability to self-renew is maintained through a process called symmetric division, which consists of the generation of another cell which maintains the functional and phenotypic properties of a stem cell . However, once activated, epithelial stem cells can generate a proliferating progeny, which is often referred to as transiently amplifying cells or committed progenitors, through a process called asymmetric division . In their normal environment, committed progenitors retain the capacity to divide and then differentiate along a particular cell lineage to make the tissue. In the kidney, CD24 + CD133 + stem/progenitor cells are localized at the urinary pole of Bowman’s capsule and exhibit self-renewal properties as well as the potential to differentiate in tubular cells or podocytes . Additional studies recently performed in adult mice have confirmed that parietal epithelial cells of the glomeruli represent a population of stem/progenitor cells with renal regenerative capacity . Of note, in vitro induction of an epithelial–mesenchymal transition induced the regression of adult renal progenitors of Bowman’s capsule to the phenotype of the embryonic renal progenitor . Accordingly, following their injection under contralateral kidney capsule of unilaterally nephrectomized mice, these renal progenitors generated novel renal tissue including neoglomerular and tubular structures at 3 weeks postengraftment .
Thus, the discovery of a population of renal stem cells localized at the urinary pole of Bowman’s capsule suggests its possible involvement in regeneration of adult tubular, as well as of glomerular, cells. However, the role of these renal stem cells in tubular and glomerular injury may be different. Indeed, following acute tubular injury, the kidney undergoes a regenerative response leading in most cases to recovery of renal function . The cell source for regenerating cells is poorly understood but several studies suggest that the tubular epithelium can be self-renewing after acute kidney injury and that differentiated tubular cells proliferate and migrate to replace the neighboring dead cells ( Fig. 15.2 ). Indeed, the tubular epithelium is resting in G1 rather than G0 and, as such, is primed to enter the cell cycle if injured ( Fig. 15.2 ). Accordingly, in most adult epithelial tissues, proliferation and migration of epithelial cells ensure replacement of adjacent injured cells, as previously reported for the skin . However, during chronic injury or in cases of extended damage, resident stem cell compartments are also activated and critically participate in regeneration .

The discovery of CD24 + CD133 + renal stem cells and their localization at the urinary pole of Bowman’s capsule suggests that these cells may participate in tubular regeneration, progressively migrating and differentiating in proximal tubular cells at the tubuloglomerular junction ( Fig. 15.3 ). Several previous studies demonstrated that the proximal tubule arises at a variety of angles from Bowman’s capsule and that the tubuloglomerular junction has an area of intermediate appearance, with prominent microvilli on parietal cells in humans, mammals and fish ( Fig. 15.3 ). These findings suggest that parietal epithelium may be able to change to tubular tissue and that this may particularly occur as the kidney grows . Accordingly, CD24 + CD133 + renal stem cells isolated from adult human kidneys generated novel tubular structures and reduced the morphological and functional kidney damage once injected in mice affected by acute renal failure, suggesting that these cells can participate in tubular regeneration in adult human kidneys . Thus, renal stem/progenitor cells may contribute to tubular epithelium repair by generating novel tubular-committed progenitors when proliferation of neighboring unwounded tubular cells is exhausted, as may occur during chronic tubular injury ( Fig. 15.3 ). In addition, transdifferentiation of parietal epithelial cells of Bowman’s capsule in cells with a proximal tubule phenotype has been reported during many types of chronic tubular injury, in association with interstitial fibrosis and tubular atrophy, or during aging . Taken together, these results suggest that injured tubular cells are mostly regenerated through proliferation of neighboring differentiated tubular cells, but that renal progenitors may participate in tubular regeneration during severe or chronic tubular injury. However, further studies are needed to verify this possibility.

By contrast, evidence is already available to demonstrate that renal stem cells are essential for glomerular regeneration. The glomerular tuft comprises three resident cell types: mesangial cells, endothelial cells and podocytes . Some studies have shown that primary injury to each of these cell types may be associated with glomerular disease . However, injury to endothelial and mesangial cells can be repaired by proliferation of adjacent cells . In addition, several lines of evidence suggest that bone marrow-derived stem cells physiologically contribute to mesangial and endothelial cell regeneration . By contrast, podocytes cannot divide, and podocyte depletion is a common determining factor that can result in glomerulosclerosis . Recently, the first evidence was provided that podocytes can be regenerated by the CD24 + CD133 + renal stem cells of Bowman’s capsule . In addition, it was demonstrated that CD24 + CD133 + renal stem cells are arranged in a precise sequence within Bowman’s capsule of adult human kidneys ( Fig. 15.2 ). A subset of more undifferentiated cells expressing renal progenitor markers in the absence of podocyte markers is localized at the urinary pole of Bowman’s capsule and acts as a bipotent progenitor for both tubular cells and podocytes, and exhibits self-renewal potential ( Fig. 15.2 ), as shown by clonal analyses demonstrating that this subset of parietal epithelial cells represents multipotent epithelial stem cells and not simply a mixture of unipotent progenitors . This was accomplished by first culturing the progeny of cells derived from single CD24 + CD133 + cells and then by their transplantation into SCID mice affected by focal segmental glomerulosclerosis (FSGS) . A transition population expressing both renal stem cells and podocyte markers is localized between the urinary and the vascular pole of Bowman’s capsule, exhibits a committed differentiative potential only towards the podocyte lineage and lacks self-renewal properties ( Fig. 15.2 ) . Finally, differentiated cells, which do not express renal stem cell markers, but display high levels of podocyte specific markers, localize at the vascular pole of Bowman’s capsule ( Fig. 15.2 ) .
These findings obtained in human kidneys were confirmed in a parallel study performed in murine kidney by Appel et al. , who also demonstrated that transitional cells with morphological and immunohistochemical features of both parietal epithelial cells and podocytes could be detected in proximity of the glomerular vascular stalk. More importantly, using genetic tagging of parietal epithelial cells in rodents, the same authors unequivocally demonstrated that podocytes are recruited from parietal epithelial cells, which proliferate and differentiate from the urinary to the vascular stalk, then generating novel podocytes ( Fig. 15.2 ) . This occurs as the kidney grows, during childhood and adolescence , and may also take place following an injury which allows a slow, regulated generation of novel podocytes, such as uninephrectomy.
The discovery that renal stem cells of Bowman’s capsule represent a potential source for podocyte generation provides the basis for a novel concept that injured podocytes can be replaced. However, in glomerular disorders characterized by acute or severe podocyte loss, regeneration requires other pathways that should allow faster replacement of injured podocytes. Indeed, the possibility that parietal epithelial cells can also migrate from Bowman’s capsule to the capillary tuft in different areas than the vascular pole was previously suggested by some studies, which proposed that areas of adhesion as well as bridges between Bowman’s capsule and the glomerular tuft may represent such sites of migration ( Fig. 15.2 ) . A recent study using genetic tagging of parietal epithelial cells demonstrated that bridges between Bowman’s capsule and the glomerular tuft in experimental models of glomerular disorders are exclusively generated by parietal epithelial cells . Taken together, the results of these studies suggest that, following podocyte injury, parietal epithelial cells generate cell bridges between Bowman’s capsule and the glomerular tuft. This may provide a slide for the migration and differentiation of an adjacent progenitor and a quick replacement of lost podocytes ( Fig. 15.2 ), or alternatively bridging parietal epithelial cells may acquire podocyte markers following injury, as recently suggested , and directly replace the lost podocytes ( Fig. 15.2 ). In summary, a large body of evidence indicates that Bowman’s capsule of adult kidneys contains a population of renal stem cells that can replace lost podocytes through multiple mechanisms and allow glomerular regeneration.
However, as in other adult organs, a response to injury can shift the balance from regeneration to repair depending on a complex interaction between the stem cell compartment and the surrounding environment . In normal conditions, the response of stem cell to injury is strictly regulated. As a consequence of resident stem cell depletion and/or uncontrolled growth, healing problems can manifest in every injured tissue and induce previously functional tissue to become a patch of cells and disorganized extracellular matrix that is referred to as a scar . Therefore, at least in proteinuric glomerular disorders, glomerulosclerosis could be envisioned like a scar induced by insufficient or exaggerated responses of renal stem cells to podocyte injury. Taken together, these results support the concept that the outcome of glomerular disorders may depend on a strictly regulated balance between the degree of podocyte injury and the amount of regeneration provided by renal stem cells.
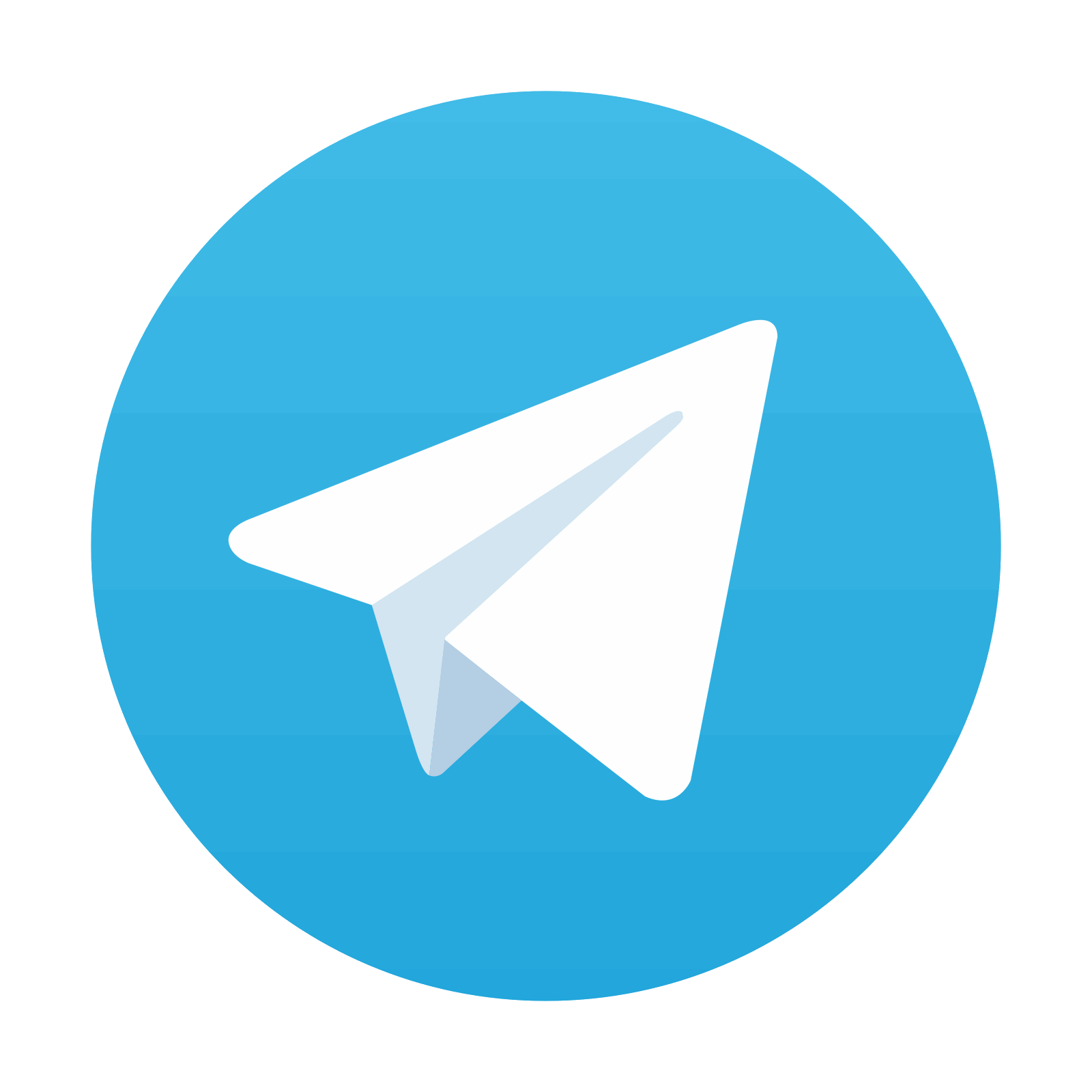
Stay updated, free articles. Join our Telegram channel

Full access? Get Clinical Tree
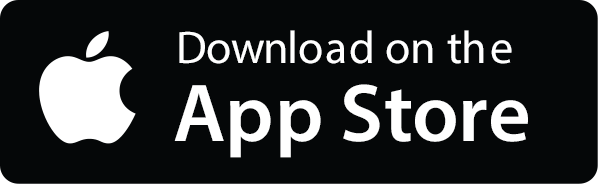
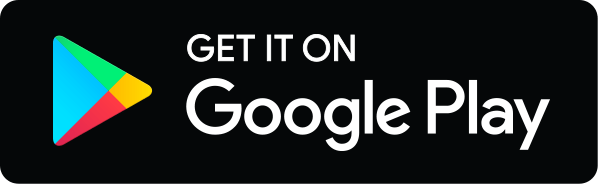