Outline
Introduction 275
Molecular Mechanisms of Stem Cell Exhaustion 277
Reactive Oxygen Species 277
Proinflammatory Cytokines 277
Proatherogenic Risk Factors 278
mTOR–Wnt Pathway 278
Adrenergic and GABA Receptor Stimulation 278
Micro-RNAs as Potent Modifiers of Stem Cell Proliferation and Differentiation 278
Impairment of Stem Cell Function 279
Mismatched Niche Capacity 279
Impaired Mobilization 280
Impaired Engraftment 281
Impaired Signaling and Transformation 282
Impaired Self-protection 282
In Vivo and Ex Vivo Therapy of Stem and Endothelial Progenitor Cells 283
Potential Mechanisms of Stem Cell-induced Organ Repair 283
Use of Artificial Niches to Store and Deliver Endothelial Progenitor Cells 284
This overview of a multitude of examples of stem cell incompetence in renal and cardiovascular disease attempts to summarize diverse molecular mechanisms inducing cell dysfunction, cell cycle arrest and loss of viability as leading causes for impaired organ regeneration after injury. Compromised stem cell trafficking from their niches to mature differentiated phenotypes accounting for the failure of repair processes is tracked. Some emerging strategies for in vivo and ex vivo stem cell therapy are summarized, along with the mechanisms of stem cell-induced organ repair.
Introduction
The preceding chapters have outlined the plethora of examples of stem cell participation in regenerative processes. An obvious question that arises with thus accrued knowledge is: why do chronic kidney diseases tend to progress? Is it a matter of a simple imbalance between the intensity of destructive processes of a particular disease and the regenerative processes linked to stem cells? Or do regenerative processes become compromised and, if this is the case, what are the mechanisms and how this ineptitude could be overcome? These straightforward enquiries will guide us next to the thick of the stem cell cycle, from quiescence and maintenance of stemness to mobilization, engraftment and differentiation into a mature cell.
The idea that stem cells may become dysfunctional in the course of a disease derives from the demonstration of their defective regenerative capacity, compromised clonogenicity, migration and capillary formation. Similar to all somatic cells, stem cells are subjected to various exogenous and endogenous stressors that could impair their competence; however, such impairment of stem cells has much more ominous consequences owing to their extended lifespan. There are plenty of examples of stem cell incompetence developing in the course of a disease. For instance, patients with systemic lupus erythematosus manifest reduced levels of circulating hematopoietic stem cells (HSCs) and endothelial progenitor cells (EPCs) even during remission . Mesenchymal stem cells (MSCs) are functionally abnormal in immune thrombotic microangiopathy . Aging is the most common cause of stem cell dysfunction . Recent studies showed that bone marrow-derived cells (BMDCs) and bone marrow-derived EPCs from db/db mice are functionally incompetent, whereas adoptive transfer of BMDCs from syngenic non-diabetic dbm mice significantly improved vasculopathy and insulin sensitivity, and partially improved renal function in db/db recipients . Hyperglycemia has been reported to reduce survival and impair the function of circulating EPCs . In addition, EPC dysfunction has been documented in type 1 and 2 diabetes, coronary artery disease (CAD), atherosclerosis, vasculitis with kidney involvement and end-stage renal disease . There is emerging evidence that senescence may serve as an important mechanism mediating EPC dysfunction. Decreased numbers and an increased proportion of senescent EPCs has been reported in patients with pre-eclampsia or hypertension . Angiotensin II can induce EPC senescence through the induction of oxidative stress and its influence on telomerase activity . Oxidized low-density lipoprotein (OxLDL) induces EPC senescence and dysfunction . Collectively, these data are supportive of the “stem cell aging” hypothesis of organismal aging . There is emerging evidence that senescence may serve as an important mechanism mediating EPC dysfunction. Dzau et al. summarized clinical situations associated with abnormalities in the number and function of EPCs ( Table 18.1 ).
Disease | Number | Function |
---|---|---|
Cardiac | ||
CAD | Reduced | Reduced |
CHF | Reduced | Reduced |
Unstable angina | Reduced | ND |
Myocardial infarction | Increased | Reduced |
Vascular | ||
Atherosclerosis | Reduced | Reduced |
Acute injury/inflammation | Increased | ND |
Peripheral limb ischemia | Increased | ND |
Transplant arteriopathy | Reduced | ND |
In-stent restenosis | Reduced | ND |
Hypertension | Reduced | Reduced |
Hyperlipidemia | Reduced | Reduced |
Diabetes | Reduced | Reduced |
Chronic kidney disease | ||
Hemodialysis | Reduced | Reduced |
The precise definition of stem cell incompetence is lacking. Based on the accepted functions of stem cells, i.e. self-renewal and asymmetric (less proven in mammals) proliferative capacity, mobilization, recruitment and engraftment, cell-dependent or paracrine signaling, viability and resistance to the existing stressors , it can be extrapolated that stem cell incompetence is characterized by the dysfunction of at least one of these properties, as depicted in Fig. 18.1 , which illustrates the cycle of injury to regeneration involving all these functions of stem cells and sites where these functions can be perturbed.

From the outset, it should be recognized that an element of uncertainty creeps into all methods of detection of stem cell populations during this train of events. Considering the stem cell cycle, its identifiable feature is that along the path of self-replication, differentiation and maturation an individual cell undergoes perpetual metamorphosis ( Fig. 18.2 ) marked by the changing landscape of the defining markers. Unless there is a one-to-one exchange of a maturing cell with a differentiating one, which is quite unlikely, the size of the pool of cells characterized by each set of markers should have an oscillatory profile. Thus, each measurement of the population of such cells inevitably represents a snapshot, while the broader picture of oscillations remains hidden from view. Until accelerating and inhibitory feedback loops along the stem cell cycle are better refined and integrated into the whole picture, it will be difficult to tell whether any particular measurement was performed at the acme or nadir point of the curve. Accepting this uncertainty may help us to understand some discrepancies in the experimental results.

Hence, examples of stem cell dysfunction abound and some general or cell-specific mechanisms can be discerned, as outlined below.
Molecular Mechanisms of Stem Cell Exhaustion
Reactive Oxygen Species
In general, most adult stem and progenitor cells show superior resistance to acute challenge with oxidants owing to their higher levels of glutathione and superoxide dismutases compared with their mature progeny. Chronic challenge with oxidants, however, dissipates this advantage. Ito et al. demonstrated that chronic states of oxidative stress induced either by an inhibitor of glutathione synthesis or by deletion of Atm (ataxia telangiectasia mutated) gene, acting through the activation of p38 mitogen-activated protein (MAP) kinase, shorten the lifespan of HSCs through increase in proliferation, resulting in stem cell exhaustion and bone marrow failure, expressed as defective bone marrow reconstitution. Fanconi anemia is an example of reactive oxygen species (ROS)-induced impairment of hematopoiesis . Mitochondrial dysfunction and increased generation of ROS may be involved in the developing stem cell incompetence .
Another example of ROS-induced stem cell incompetence is represented by Adriamycin (doxorubicin) toxicity. Adriamycin toxicity has been ascribed in part to mitochondrial dysfunction due to the excessive production of ROS . Potential downstream signaling of ROS may explain why Adriamycin-associated nephropathy (AAN) has been attributed to activation of p38 MAP kinase and transforming growth factor-β 1 (TGF-β 1 )/Smad signaling . It is instructive that both the kidney-resident side population cells, as well as the side population-excluded main population of cells, adoptively transferred to mice with AAN decreased proteinuria . Deleterious effects of Adriamycin on glomerular endothelial cells have been linked to the development of proteinuria . Vascular dysfunction manifesting in blunted responses to acetylcholine was also demonstrated in in vitro studies of isolated thoracic aorta obtained from Adriamycin-injected rats , arguing for the development of endothelial dysfunction under this condition. The viability of EPCs after Adriamycin administration was examined in vitro . EPCs obtained from AAN mice showed impaired clonogenicity. Cultured EPCs treated with various doses of Adriamycin exhibited apoptosis, necrosis and signs of stress-induced premature senescence. Previous studies on Adriamycin nephropathy showed that bone marrow-derived cells engrafted the kidneys, and transdifferentiated into endothelial cells and myofibroblasts, which coexpressed endothelial markers suggestive of endothelial–mesenchymal transformation . In the case of Adriamycin-associated nephropathy, EPCs also develop signs of impaired viability, premature senescence and functional incompetence, as judged by the higher frequency of apoptosis, staining for senescence-associated β-galactosidase and reduced clonogenic potential. The finding that exogenous intact EPCs improve structural and functional consequences of Adriamycin administration not only further supports the role of these cells in kidney regeneration, but also argues in favor of EPC competence as the necessary prerequisite for accomplishing regenerative functions. Other examples of ROS-induced stem cell incompetence have been recently reviewed and are presented below in the context of various initiating factors.
Proinflammatory Cytokines
Cytokines modulate various aspects of stem cell behavior. In MSCs pretreated with dexamethasone and committed to osteogenic differentiation, interleukin-6 (IL-6) and its soluble receptor (sIL-6R) enhance osteoblastic differentiation . This effect depends on the expression of Gp130 and requires phosphorylation of Stat-3. Indeed, IL-6 is a robust marker of inflammation and endothelial dysfunction and its levels correlate with atherosclerotic burden in carotid arteries of patients with chronic kidney disease (CKD) . Whether IL-6 can participate in the development of vascular calcification by misdirecting vascular wall MSC differentiation remains to be examined. IL-6 and tumor necrosis factor-α (TNF-α) inhibit differentiation and maturation of a broad range of stem cells, including neuronal, whereas IL-4 promotes these processes . Macrophage inhibitory protein-1α (MIP-1α) inhibits proliferation of multipotent stem cells , although these data could not be confirmed (M. Ratajczak, personal communication). TGF-β 1 can also provide inhibitory instructions for stem cell proliferation , although this issue is in need of re-evaluation. Members of the TGF-β family, bone morphogenetic proteins (BMPs), have emerged as negative regulators of proliferation in stem cells of intestinal crypts and hair follicles . In combination with leukemia inhibitory factor (LIF), BMPs, acting via Smad pathway on Id genes, suppress differentiation of embryonic stem cells and maintain their self-renewal .
The chemokine RANTES (regulated on activation, normal T cell expressed and secreted) promotes homing of bone marrow-derived endothelial progenitors to inflamed glomeruli, although in the particular case of a rat model of anti-Thy-1.1 glomerulonephritis induced by mesangiolysis, manipulation of EPC homing does not appear to affect the outcome . IL-10 secretion by monocytes/macrophages activated by LPS is enhanced by the reprogramming executed by bone marrow-derived MSCs in a cyclooxygenase- and Toll-like receptor-4 (TLR-4)-dependent manner, thus resulting in better survival of endotoxemic mice .
Delta-like-1/fetal antigen-1 (dlk1), a member of the epidermal growth factor-like homeotic protein family known as a modulator of MSC and HSC differentiation, is overexpressed during liver and muscle regeneration and at early stages of liver fibrosis, and has been found to stimulate IL-1α, IL-1β, IL-6, CXCL1 (growth-related oncogene-α/keratinocyte), CXCL2 (macrophage inflammatory protein-2/GRO-β) CXCL3 (GRO-γ), CXCL6 (granulocyte chemotactic protein-2), CXCL8 (IL-8) and CCL20 (MIP-3α) in human MSCs, where it inhibits adipocytic and osteoblastic differentiation .
Proatherogenic Risk Factors
It has been demonstrated that atherosclerotic risk factors inversely correlate with the number of circulating EPCs . Hyperglycemia, type 1 and 2 diabetes and hypertension are well-established causes of EPC dysfunction . OxLDL inhibits vascular endothelial growth factor (VEGF)-induced differentiation of EPCs via deactivation (dephosphorylation on Ser 473 ) of the serine/threonine kinase Akt pathway . Several potential mechanisms mediating this effect of oxLDL exist: activation of protein phosphatase-2A or lipid phosphatases, such as phosphatase and tensin homolog deleted on chromosome 10 (PTEN) or inositol-5 phosphatase-2 (SHIP-2) inactivating phospholipid products of PI-3 kinase. Many effects of proatherogenic factors on stem cells are mediated via ROS. Much less information is available on the role of proatherogenic factors in modulating HSCs and MSCs.
mTOR–Wnt Pathway
The canonical Wnt/β-catenin pathway is one of the key regulators of stem cell self-renewal . Consisting of 19 secretory cysteine-rich glycoproteins, Wnt signal via Frizzled receptors and members of low-density lipoprotein receptors LRP5 and LRP6 . Activation of this pathway plays a role in embryonic development, tissue maintenance and regeneration, with persistent activation inducing various tumors or cell senescence . Wnt3A and Wnt5A are essential in modulating self-renewal of HSCs . An inhibitor of Wnt signaling, Dickkopf-1, is necessary for HSC re-entry into the cell cycle . Recent findings in epidermal stem cells indicate that prolonged Wnt signaling results in a mammalian target of rapamycin (mTOR)-mediated exhaustion of stem cells and their premature senescence and mTOR inhibitor, rapamycin, protects stem cells against developing senescence. This mechanism of developing senescence is considered as epithelial protection against uncontrolled proliferation and tumorigenesis. Another inhibitor of mTOR, the regulated in development and DNA damage-1 (REDD-1), is a hypoxia-induced protein that may have relevance to diverse pathological conditions . Other potential targets of Wnt signaling include increased expression of HoxB4 and Notch1 genes . Extensively studied in embryogenesis, the role of Notch signaling is much less examined in adult stem cells. There is, however, evidence that it can restore regenerative capacity of skeletal muscle of old mice and its inhibition impairs it .
Adrenergic and GABA Receptor Stimulation
Hematopoietic Lin − Sca + progenitor cells (HPCs) express adrenergic receptors. Catecholamine stimulation of HPCs results in enhanced formation of ROS, activation of the p38/MAPK pathway and decreased capacity to form granulocyte/macrophage colonies, which is reversible upon antioxidant or p38 inhibition treatment . Adrenergic stimulation also leads to transcriptional activation of p16, invoking the possibility of cell cycle arrest and premature senescence of HPCs. This mechanism, prevalent in aging, congestive heart failure and a variety of other conditions, may have important implications for defective regenerative and immune defense processes.
Activation of γ-aminobutyric acid-A (GABA A ) receptors negatively controls embryonic and neuronal stem cell proliferation independent of differentiation, apoptosis or damage to DNA . The effects of GABA are transduced via S-phase checkpoint kinases and the histone H2AX. With the broadening specter of GABA receptor expression, its role in adult stem cells remains to be discovered.
Micro-RNAs as Potent Modifiers of Stem Cell Proliferation and Differentiation
The past decade has witnessed the emergence of micro-RNAs (miRNAs) as key regulators of stem cell proliferation and differentiation in embryonic development and in adult life . It is estimated that humans harbor about 1000 different miRNAs, 19–25 nucleotide-long non-coding RNA transcripts, and 700 of them had been confirmed at the time of writing (sequences deposited in http://microrna.sanger.ac.uk ). The complexities of their synthesis have been partially decoded. RNA polymerases II and III generate long primary miRNA transcripts, which undergo cleavage by nuclear RNase Drosha and DiGeorge syndrome critical region gene 8 (DGCR8) protein to produce an ≈70 nucleotide-long precursor, (pre)-miRNA, that is exported to the cytoplasm and employs another RNase, Dicer, to generate the mature miRNA. Mature miRNA in turn forms a complex with Argonaute proteins, a catalytic portion of the RNA-induced silencing complex (RISC), binds to the 3′ untranslated region (3′UTR) of target RNAs, and either accelerates their degradation (perfect complementation) or induces translational suppression (imperfect complementation). The predominant miRNAs expressed in embryonic stem cells are limited to four loci: miR-290–295 (most abundant), miR-15–16, miR-17–92, and miR-21 clusters. In Dicer1 or DGCR8 null mice, ESCs lack expression of the pluripotent marker Oct4, fail to proliferate, and embryonic lethality occurs on E7.5 . In contrast, conditional Dicer1 mutant mice are viable and although they express Oct4, cells fail to differentiate towards endodermal and mesodermal lineages upon stimulation , suggesting that one of the main functions of miRNAs is to permit differentiation by silencing pluripotency factors.
One of the silencing mechanisms is attributed to miR-134, which is induced by retinoic acid and targets two positive regulators of Oct4, Nanog and liver receptor homolog-1 (LRH-1) . In adult HSCs, miR-181 appears to be responsible for differentiation towards B-lymphoid lineage without affecting T-cell lineage, whereas overexpression of miR-223 and miR-142 is responsible for upregulation of the T-lymphoid lineage .
Cell cycle progression represents another target of miRNAs. Along these lines, the miR-290 cluster represses cyclin E-cdk2 inhibitors and the miR-302 cluster targets cyclins D1 and D2 , thus promoting rapid G1-S checkpoint transition characteristic of ESCs. A similar function is executed by miR-92b, which represses the cyclin-dependent kinase inhibitor p57 . In contrast, miR-24, which is upregulated during physiological terminal differentiation of HSCs, muscle and neuronal cells, inhibits cell cycle progression by repressing E2F2 , an example of its role in differentiation to the mature phenotype.
Regulation of miRNA biogenesis by cytokines is exemplified by TGF-β/BMP signaling that induces miR-21 expression with the subsequent downregulation of the programmed cell death 4 (PDCD4) . miR-221 and miR-222 inhibit normal erythopoiesis and modulate angiogenesis by targeting c-Kit receptor for stem cell factor .
So far, the role of miRNAs in developing stem cell incompetence has been underinvestigated. Expression profiling of miRNAs in senescing mouse embryo fibroblasts revealed that miR-290 is commonly upregulated and it correlates with other senescence-associated genes such as p19Arf , p16 and p21 . This pathway is also involved in stress-induced premature senescence triggered by nocodazole treatment. The subject is ripe for investigation of miRNAs in various forms of stem cell dysfunction.
In conclusion, these molecular mechanisms ( Fig. 18.3 ), solely or in combination, contribute to stem cell incompetence which manifests itself in the diverse dysfunctional states, as discussed below.

Impairment of Stem Cell Function
Mismatched Niche Capacity
The ability of stem cell niches to adjust their capacity to harbor stem cells in accord with physiological requirements and the loss of this capacity under diverse pathological conditions have been recently noticed. Zhang et al. and Calvi et al. have disclosed complex relations within the bone marrow osteoblastic–HSC niche intricately regulated by parathyroid hormone and bone morphogenetic protein leading to the increased niche capacity. The questions related to the capacity of niches have come into focus in investigations of cancer cells, which may create their own niche and/or hijack the pre-existing ones . Moreover, the first evidence linking stem cell niches with disease initiation and progression has arrived from studies of primary myelofibrosis, which is associated with an imbalance between endosteal and vascular niches resulting in clonal cell proliferation .
In an attempt to visualize potential sites of stem cell residence, MSC used as a bait were used presented to ex vivo control and postischemic kidney sections, and stem cell adhesion was examined as a surrogate marker of niche capacity . Specificity of MSC adhesion was confirmed by demonstrating that (i) 3T3 cells displayed 10-fold lower adhesion, and (ii) MSC adhesion was CXC chemokine receptor-4 (CXCR4)/stromal cell-derived factor-1 (SDF-1) dependent. MSC adhesion was asymmetrical, with postischemic sections exhibiting greater than two-fold higher adhesion than controls, and showed a preference for perivascular areas. Pretreatment of kidney sections with cRGD resulted in increased MSC adhesion (through displacement of resident cells), whereas blockade of CXCR4 (with AMD3100) or inhibition of α 4 β 1 (VLA4) integrin or its counterpart, vascular cell adhesion molecule-1 (VCAM-1), reduced adhesion. The difference between adhered cells under cRGD and control conditions reflected prior occupancy of the binding sites with endogenous cells. AMD3100-inhibitable fraction of adhesion reflected CXCR4-dependent adhesion, whereas maximal adhesion was interpreted as the MSC-lodging capacity of the kidney. The data demonstrated that (i) CXCR4/SDF-1-dependent adhesion increases in ischemia, (ii) preoccupancy of MSC binding sites is decreased, and (iii) the capacity of MSC binding sites is expanded in postischemic kidneys. A similar technique was used to study MSC adhesion to myocardium obtained from control and Adriamycin-treated mice, which revealed that Adriamycin cardiomyopathy is associated with increased niche capacity and preoccupancy, with only a minor CXCR4/SDF-1 dependence of adhesion (unpublished observations). This field remains in its infancy and requires further exploration.
Impaired Mobilization
Impaired stem cell mobilization can occur owing to the inadequacy of mobilizing signals, their shielding or impaired detachment from their niches. Hematopoietic stem and progenitor cells, akin to lymphocytes, exhibit constitutive trafficking routes from the bone marrow to various extramedullary organs, where they reside for several days and then return to the bloodstream via lymphatics . Sphingosine-1 phosphate receptors govern the egress of stem cells from the tissues to lymphatic capillaries. This sentinel cycle allows for replenishment of organ-resident stem cells and for their extramedullary proliferation upon local activation by TLR agonists. Along this route, sphingosine-1 phosphate and receptors and TLRs can be potentially targeted by disease processes, resulting in either impaired drainage or excessive local proliferation.
A classical mobilization agent, granulocyte colony-stimulating factor (G-CSF), executes dislodgment of stem cells from the bone marrow niche by activating neutrophil elastase, which in turn cleaves SDF-1 . A closely related granulocyte/macrophage CSF mobilizes 5–100-fold higher numbers of HSCs, MSCs and EPCs compared with baseline levels . Caveolin-1 deficiency blocks efficient dimerization of CXCR4, thus facilitating stem cell mobilization, but negatively affecting stem cell engraftment , whereas its overexpression may result in enhanced SDF-1/CXCR4 binding in the bone marrow and defective mobilization.
Mobilization of EPCs from their respective niches can be accomplished by mechanical injury and ischemic stress through generation of hypoxia-inducible factor-1 (HIF-1)-regulated release of vascular endothelial growth factor, erythropoietin and SDF-1, as well as by placental growth factor, G-CSF and granulocyte–macrophage colony-stimulating factors . Some proinflammatory cytokines, such as IL-8, are potent stimulators of EPC and HSC mobilization, thus linking this response to stressors with proinflammatory conditions . An additional mechanism for EPC mobilization has been attributed to apoptotic endothelial microparticles , a feedback mechanism of a well-timed surge in stem cells when injury occurs. Microparticles (microvesicles) shed from the live cells have been implicated in the bidirectional transfer of genetic information between stem cells and injured somatic cells . In relation to the kidney, microvesicles derived from injured tubular epithelia may induce phenotypical changes in MSCs through expression of some tubular cell markers .
Several investigators have addressed the question of whether stem cells can be efficiently delivered to areas of tissue ischemia to preserve or restore end-organ function by participating in vasculogenesis. In a model of myocardial infarction in bone marrow-transplanted mice, histological analysis showed donor-derived endothelial cells in areas of neovascularization at the border zone of the infarct . These experimental data have been strongly supported by clinical observations. Adams et al. found increased levels of circulating EPCs in patients with CAD after exercise-induced myocardial ischemia. Lambiase et al. showed an inverse correlation between the density of coronary collaterals and numbers of peripheral EPCs in CAD. Such an inverse correlation between the number and function of circulating EPCs and the severity of various chronic cardiovascular and renal diseases is a rather general rule .
Pharmacological mobilization of EPCs can be achieved using statins, VEGF, erythropoietin, angiotensin-converting enzyme (ACE) inhibitors and estrogens, to name a few . These agents exert their effects at least in part via activation of endothelial nitric oxide synthase (eNOS), making nitric oxide (NO) production an obligatory part of the response to mobilizing agents. Mice deficient in eNOS showed reduced mobilization . Considering the fact that eNOS is usually uncoupled (the state of dissociation of C-terminal reductase domain from the N-terminal oxygenase domain resulting in the reduced generation of NO, enhanced generation of the superoxide anion and peroxynitrite) in many diseases, and in view of the fact that mobilization of EPCs is dependent on functional eNOS, it is not surprising that this function may become impaired.
Acute ischemia is one of the potent signals to mobilize EPCs; this has been unequivocally documented in humans and in experimental animals with myocardial ischemia, ischemic stroke and renal ischemia . Despite the apparent universality of this response to ischemic insult, the precise molecular mechanisms responsible for it remain uncertain.
What are stress signaling (SOS) molecules, produced by and discharged from the ischemic tissue, which are capable of downstream mobilization and recruitment of stem and endothelial progenitor cells? Uric acid and high-mobility group protein (HMGP) are prototypical alarm signals activating the innate immune system, which exhibit a short-lived surge after ischemia–reperfusion injury. Previous studies demonstrated that exogenous uric acid leads to a rapid mobilization of EPCs and HSCs and protection of the kidney against ischemic injury . Kuo et al. demonstrated that monosodium urate (MSU) in vitro and in vivo resulted in exocytosis of Weibel–Palade bodies with the release of IL-8, von Willebrand factor (vWF) and angiopoietin-2 into the culture medium and circulation, respectively. In TLR-4-deficient mice, acute elevation of uric acid level by injection of MSU did not result in the release of vWF and angiopoietin-2 to the circulation, suggesting that uric acid affected exocytosis of Weibel–Palade bodies via this receptor. The release of IL-8 in response to elevated uric acid level required both TLR-2 and TLR-4. These findings outline a novel paradigm linking postischemic repair and inflammation via the release of the constituents of Weibel–Palade bodies and further broaden the spectrum of alarm signaling to establish constituents of Weibel–Palade bodies as potential second messengers not only for proinflammatory responses but also for mobilization of stem cells. The important feature of this response is that it is only a brief transient surge of uric acid that has this signaling function, whereas chronic elevation of uric acid not only lacks this type of alarm signaling, but inhibits ischemia-induced mobilization .
Interactions between HSCs and stromal cells are mediated in part through α 4 β 1 (VLA4)/VCAM-1 interaction . Accordingly, inducible ablation of α 4 β 1 (VLA4) or conditional ablation of VCAM-1 is associated with the enhancement of G-CSF-induced mobilization of HSCs . An additional mechanism of leptin-induced upregulation of αVβ 5 and α 4 integrins may explain enhanced adhesion to neointimal lesions , but the same phenomenon would appear to inhibit EPC mobilization, thus requiring further analysis. The issue of adhesion molecules participating in stem cell adhesion to respective niches has been comprehensively reviewed elsewhere . There remain multiple unanswered questions related to the efficacy of stem cell mobilization by intrinsic or pharmacological means in CKD, which need to be addressed in future studies.
Impaired Engraftment
The process of engraftment is just as complex as is a similar process of leukocyte egress; it includes tethering, adhesion, transmigration and final lodging of stem cells from the circulatory bed to the tissues. Adhesion and transmigration are initiated in part by integrins. The pathways involved in homing of bone marrow-derived stem cells have been identified as α 4 β 1 -integrin and thymosin β 4 .
Many ligands participate in the recruitment of stem cells to specific tissues. Homing of HSCs, EPCs and a subpopulation of MSCs which expresses CXCR4 is governed mainly by the α-chemokine SDF-1 . Both CXCR4 and SDF-1 are expressed in the normal kidney and their expression is already enhanced 24 h after ischemia . Impaired engraftment can occur owing to reduced CXCR4/SDF-1 expression or function.
There are many factors affecting binding of SDF-1 to its cognate G-protein-coupled seven-transmembrane domain receptor CXCR4 . SDF-1/CXCR4 binding activates several pathways, such as FAK/Paxillin, PI-3K/Akt, MEK, Jak/STAT, nuclear factor-κB (NF-κB), matrix metalloproteinases (MMPs) and phosphatases Ship 1, 2 and CD45, thus regulating cell motility and chemotaxis, adhesion, survival and secretion. Upon receptor activation, CXCR4 undergoes oligomerization/dimerization, a process that takes place in lipid-rich domains and requires caveolin-1 expression . Other potential ligands engaged in homing of stem cells, albeit significantly less explored, include chemokines MCP-3 (homes MSCs) and GRO-1 (homes bone marrow-derived EPCs, especially in tumors).
The knowledge of where to home may be also affected by impaired niche capacity, as discussed above.
Impaired Signaling and Transformation
Impaired signaling and transformation of stem cells can occur as a result of either perturbed paracrine secretion or differentiation into a mature cell. The capacity of multipotent stem cells to differentiate towards mature cells of diverse lineages has a stochastic and an instructive component. Usually, it is most probably the latter that is in error under pathological conditions. Mesenchymal stem cells undergo osteogenic differentiation when stimulated by epidermal growth factor (EGF), but not by the closely related platelet-derived growth factor (PDGF). Proteome-based comparison of tyrosine phosphorylated proteins in MSCs showed that more than 90% overlapped upon stimulation with either agonist, whereas the phosphatidylinositol–protein kinase B pathway was activated exclusively by PDGF , suggesting that this kinase represents a checkpoint for MSC differentiation. Blocking this pathway converts the effect typical of PDGF to that resembling EGF. It remains to be seen whether this mechanism may participate in the development of ectopic calcification.
The ability of circulating progenitors to differentiate towards endothelial or smooth muscle cell lineages has been furthered by studies of a parabiotic model in which a wild-type mouse and a transgenic mouse expressing green fluorescent protein (GFP) are conjoined subcutaneously via anastamosing circulations. Tanaka et al. demonstrated that mechanical injury to femoral arteries of wild-type mice resulted within 4 weeks in a chimerism of cells comprising developing neointima: 15% and 31% of parabiotic partner-derived GFP-positive cells were detected in the intimal and medial layers, respectively, with some cells expressing α-smooth muscle actin (α-SMA), others CD31. Studies using adoptive transfer of MSCs to mice with acute renal ischemia showed that in this in vivo model the majority of transplanted cells differentiated towards α-SMA-expressing cells and a smaller portion towards CD31-expressing cells, whereas in vitro the proportions were reversed .
A dramatic example of the inappropriate TGF-β signaling resulting in hypercellular lesions of different podocytopathies has been described by Smeets et al. , demonstrating that the population of CD133 + CD24 + renal progenitors proliferated and deposited extracellular matrix, thus contributing to the development of the so-called tip lesion and crescent formation.
Mechanical stress may be another important instructive signal for stem cell differentiation decision. When bone marrow MSCs were subjected to flow- or pressure-induced stress, as opposed to static culture conditions, a 12-fold higher proportion of the cells acquired smooth muscle/myofibroblastic characteristics . These observations may have far-reaching significance for fibrotic processes where distorted or enhanced mechanical forces exerted by fibrogenic transformation of the parenchyma could bias the differentiation decisions.
Impaired Self-protection
The loss of stem cell viability and resistance to ongoing stress may occur in various disease states associated with oxidative stress. Ito et al. convincingly demonstrated that chronic states of oxidative stress induced either by an inhibitor of glutathione synthesis or by deletion of Atm (ataxia telangiectasia mutated) gene, shorten the lifespan of HSCs through an increase in proliferation, stem cell exhaustion and bone marrow failure, expressed as defective bone marrow reconstitution. This is an example of ROS inducing an accelerated type of replicative senescence. This author’s group demonstrated that oxidative stress in type 2 diabetic db/db mice is associated with an increased proportion of apoptotic EPCs under basal conditions and reduced viability under conditions of stress . Similar findings were described in type 2 diabetic patients: EPCs exhibited impaired proliferation, adhesion and incorporation into vascular structures . Evidence was provided that pharmacological restoration of stem cell competence, both ex vivo and in vivo using a seleno-organic antioxidant and peroxynitrite scavenger, is possible . Holmen et al. described a most intriguing phenomenon whereby circulating inflammatory endothelial cells expressing two inflammation-associated markers, vascular adhesion protein-1 and major histocompatibility complex (MHC) class I-related chain A, in addition to high levels of inducible NOS and neutrophil-activating chemokines, contribute to the impairment of proliferation, migration and clonogenicity of EPCs in patients with Wegener granulomatosis. This mechanism may have much broader pathological implications.
To draw a preliminary conclusion, stem/progenitor cells are differentially affected in different models of CKD. For instance, in Adriamycin nephropathy the prevailing defect could be traced to the inability of stem/progenitor cell homing to the kidney owing to the cytotoxic effect of this anthracycline antibiotic; in diabetic nephropathy the defect may be explained predominantly by oxidative stress and reduced viability of stem/progenitor cells; in unilateral ureteral obstruction, pathology of stem/progenitor cell predilection towards myofibroblastic differentiation may be palpably responsible for the ensuing tubulointerstitial fibrosis and scarring. These hypothetical pathogenic mechanisms require in-depth investigation, as the delineation of the causes of stem/progenitor cell incompetence is a prerequisite to designing effective therapies.
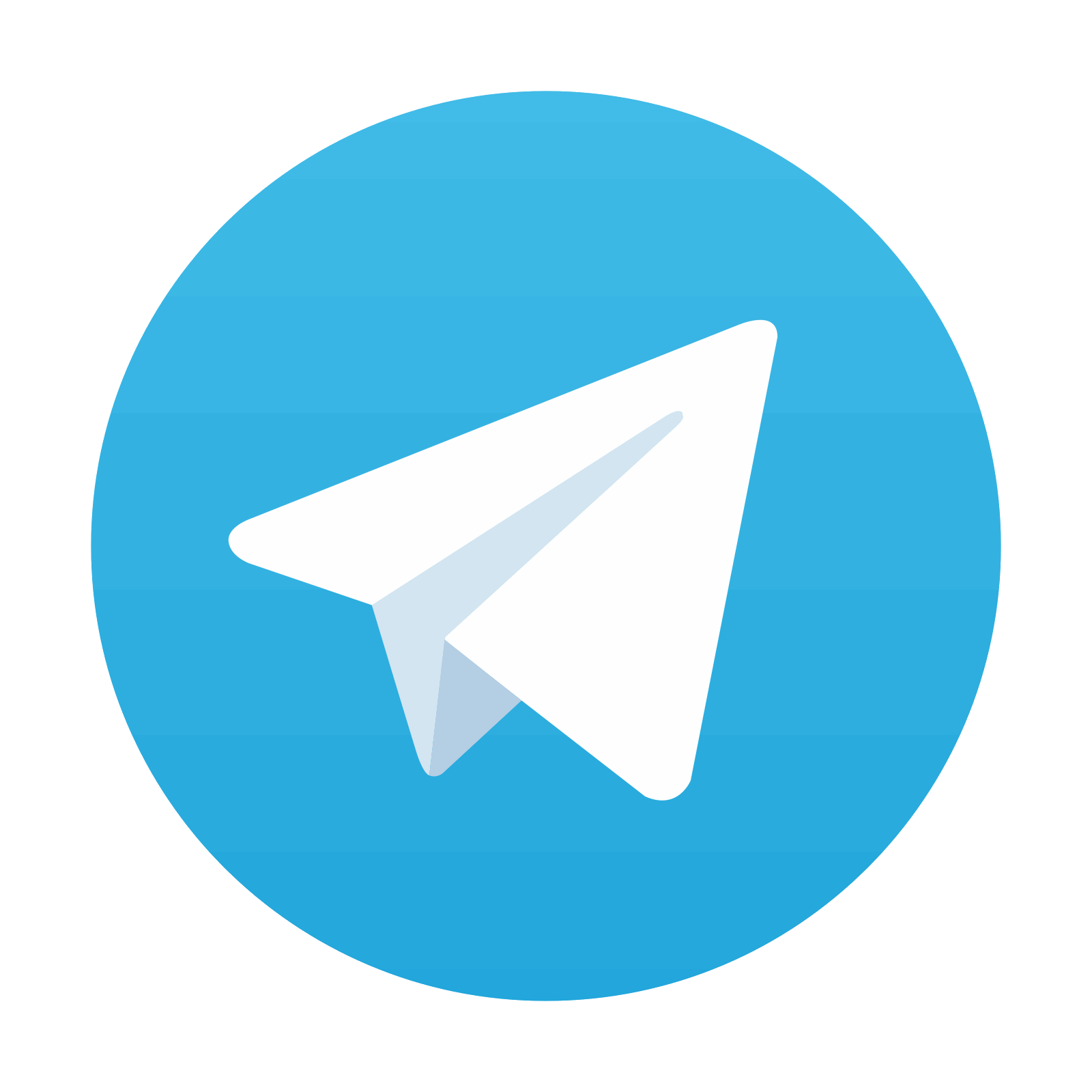
Stay updated, free articles. Join our Telegram channel

Full access? Get Clinical Tree
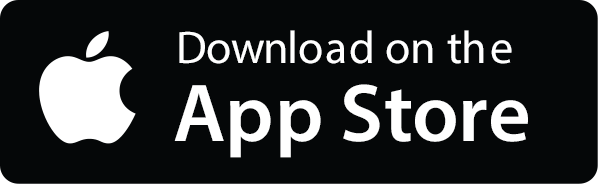
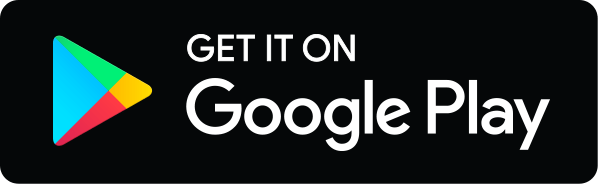