Age
Hemoglobin (g/dL
Mean corpuscular volume (fL)
Mean
Lower limita
Mean
Lower limita
Birth (cord blood)
16.5
13.5
108
98
1–3 days (capillary)
18.5
14.5
108
95
1 week
17.5
13.5
107
88
2 weeks
16.5
12.5
105
86
1 month
14.0
10.0
104
85
2 months
11.5
9.0
96
77
3–6 months
11.5
9.5
91
74
0.5–2 years
12.0
11.0
78
70
2–6 years
12.5
11.5
81
75
6–12 years
13.5
11.5
86
77
12–18 years female
14.0
12.0
90
78
12–18 years male
14.5
13.0
88
78
18–49 years female
14.0
12.0
90
80
18–49 years male
15.5
13.5
90
80
Etiology
The causes of anemia in children can be classified by the physiological process of red blood cell (RBC) production and loss or by morphology based on the size of the RBCs. The most common causes based on this classification are listed in Table 12.2. The physiological causes of anemia consist of decreased RBC production, bone marrow failure, dyserythropoiesis, hemolytic anemias, and blood loss. Morphological classification of anemia is divided into three major groups based on the mean corpuscular volume (MCV) of the RBC as microcytic (MCV below the lower limit for age), macrocytic (>100 fL), and normocytic (78–100 fL). However, these categories are not mutually exclusive as may occur when there is more than one mechanism causing anemia. For example, anemia associated with chronic kidney disease could be related to decreased erythropoietin production, iron deficiency, effect of uremia on red blood cell membranes, and/or chronic inflammation. Macrocytic RBCs are frequently seen in hemolytic anemias due to either an increased number of macrocytic reticulocytes or a relative deficiency of folic acid due to increased RBC turnover. Likewise, a microcytic, hypochromic (decrease in mean corpuscular hemoglobin) anemia can occur due to the coexistence of iron deficiency and lead poisoning. Finally, the classification of anemia can change during the course of the disease from one category to another. For example, RBCs are typically normocytic during the initial stages of chronic disease but can become microcytic in the later stage due to associated iron deficiency.
Table 12.2
Classification of anemia
Physiologic |
I. Impaired red blood cell production |
A. Nutritional deficiencies |
a. Iron deficiency |
b. Folic acid deficiency |
c. Vitamin B12 deficiency |
d. Protein calorie malnutrition |
i. Marasmus, |
ii. Kwarshiorkar |
e. Vitamin B6 deficiency |
f. Scurvy |
B. Bone marrow failure |
a. Pure red cell aplasia |
i. Diamond Blackfan anemia |
ii. Transient erythroblastopenia of childhood |
b. Aplastic anemia |
c. Inherited bone marrow failure syndromes |
d. Marrow infiltration |
i. Malignancies |
ii. Osteopetrosis |
iii. Storage disorders |
C. Dyserythropoiesis |
a. Thalassemia syndromes |
b. Lead poisoning |
c. Sideroblastic anemia |
d. Congenital dyserythropoietic anemia |
e. Erythropoietic protoporphyria |
D. Miscellaneous |
a. Anemia of chronic disease |
b. Hypothyroidism |
c. Anemia due to chronic kidney disease |
d. Connective tissue disorders |
e. Hemoglobin mutants with decreased affinity for oxygen |
II. Hemolytic anemia (increased red blood cell destruction) |
A. Defects of hemoglobin |
a. Quantitative disorder (Thalassemias) |
b. Qualitative disorder (sickle cell disease) |
B. RBC membrane defects |
a. Hereditary spherocytosis |
b. Hereditary elliptocytosis |
c. Hereditary stomatocytosis |
C. Enzyme deficiencies |
a. Glucose 6-phosphate dehydrogenase deficiency |
b. Pyruvate kinase deficiency |
D. Immune |
a. Isoimmune |
i. Rh incompatibility |
ii. ABO incompatibility |
b. Autoimmune |
i. Primary or idiopathic |
ii. Secondary |
1. Lupus |
2. Other connective disorders |
E. Miscellaneous |
a. Microangiopathic hemolytic anemia |
i. Thrombotic thrombocytopenic purpura |
ii. Hemolytic uremic syndrome |
b. Paroxysmal nocturnal hemoglobinuria |
c. Burns |
d. Hypersplenism |
III. Blood loss |
a. Acute |
i. Fetomaternal hemorrhage |
ii. Trauma |
iii. Splenic sequestration |
b. Chronic |
i. Gastrointestinal blood loss |
Morphologic |
I. Microcytic |
a. Iron deficiency anemia |
b. Thalassemia: Alpha thalassemia, Beta thalassemia |
c. Hemoglobinopathies |
d. Anemia of chronic disease (later stages) |
e. Chronic lead poisoning |
f. Sideroblastic anemia |
II. Macrocytic |
a. Folic acid deficiency |
b. Vitamin B12 deficiency |
c. Aplastic anemia |
d. Hypothyroidism |
e. Liver disease |
f. Diamond Blackfan anemia |
g. Inborn error of metabolism: Hereditary orotic aciduria |
III. Normocytic |
a. Acute blood loss |
b. Hemolytic anemia |
c. Chronic renal disease |
d. Anemia of chronic disease (early stages) |
e. Splenic sequestration |
Diagnostic Approach
Clinical
Anemia in children can be identified through universal screening or based on signs and symptoms. The American Academy of Pediatrics recommends universal screening with a hemoglobin or hematocrit measurement for all infants at 12 months of age [6]. In addition, newborn screening is mandated and can identify patients with hemoglobinopathies (sickle cell disease, beta thalassemia). The signs and symptoms of anemia that may be present largely depend upon how abrupt the onset of anemia is. The onset can be very rapid (e.g., acute blood loss, hemolysis) or insidious (iron deficiency, anemia of chronic disease, lead poisoning). Family and medical history often provide valuable information in the diagnostic evaluation of an anemic patient. The majority of children with mild anemia of insidious onset are clinically asymptomatic. Infants and young children often present with non-specific symptoms such as poor feeding, irritability, excessive sleepiness, or tiredness. Older children and adolescents may have shortness of breath, palpitations, syncope, or exercise intolerance. Parents also often report pallor as a presenting symptom, which has poor sensitivity for predicting mild anemia, but correlates well with severe anemia [7, 8]. Physical examination may show pallor, jaundice, lymphadenopathy, hepatosplenomegaly, frontal bossing and congenital anomalies, and reflect a variety of causes of anemia. Vital signs (heart rate, respiratory rate, blood pressure, and oxygen saturation) should be evaluated in all anemic patients to ensure hemodynamic stability. In addition, patients may have signs and symptoms associated with a chronic condition such as systemic lupus erythematosus, rheumatoid arthritis, chronic kidney disease, or hepatic disease. Clinical clues for some causes of anemia are enumerated in Table 12.3.
Table 12.3
Historical clues in the evaluation of anemia in children
Clinical clue | Suspected cause of anemia |
---|---|
Excess intake of cow’s milk | Iron deficiency |
Chronic blood loss | Iron deficiency |
Exclusive intake of goat’s milk | Folic acid deficiency |
Strict veganism | Vitamin B12 deficiency |
Pica | Iron deficiency, lead poisoning |
Family history of splenectomy, cholecystectomy | Hereditary spherocytosis |
Family origin of Mediterranean and Middle Eastern ancestry | Beta thalassemia, glucose 6-phosphate dehydrogenase (G6PD) deficiency |
Family origin of South East Asian ancestry | Alpha thalassemia, HbE disease (Cambodia, Laos) |
Exposure to drugs, toxins | G6PD deficiency, folic acid deficiency, lead poisoning |
Ingestion of certain foods, e.g., Fava beans | G6PD deficiency |
Laboratory
The initial laboratory evaluation of anemia includes a complete blood count, reticulocyte count, and a peripheral smear. Most physicians use the morphological classification of anemia based on MCV (microcytic, macrocytic, or normocytic) to help guide the diagnostic process (Table 12.2). An elevated reticulocyte count (or percentage) suggests hemolysis or chronic blood loss as the cause of anemia, whereas a decreased count (or percentage) suggests decreased RBC production. A reticulocyte index (reticulocyte count x patient’s hematocrit/normal hematocrit) is a more accurate marker of erythropoietic activity. In general, a reticulocyte index ≥3 indicates chronic blood loss or a hemolytic process, while an index of <3 (often <1.5) is seen in patients with impaired RBC production [9]. Evaluation of a peripheral blood smear of a child may provide particularly valuable clues toward a particular etiology of anemia. For example, the presence of a large number of spherocytes strongly suggests the diagnosis of hereditary spherocytosis. Table 12.4 lists some of the important blood smear findings and their associated clinical significance. The use of special RBC stains is necessary to identify inclusion bodies such as Heinz bodies (RBC enzymopathies, chronic liver disease, asplenia), siderocytes (aplastic anemia, chronic infection), and intraerythrocytic parasites (malaria, babesiosis).
Table 12.4
Diagnostic significance of peripheral blood smear
Finding | Conditions seen |
---|---|
Target cells | Hemoglobin SC disease, HbE disease, thalassemias, severe iron deficiency |
Spherocytes | Hereditary spherocytosis, immune hemolytic anemia |
Elliptocytes or Ovalocytes | Hereditary elliptocytosis |
Stomatocytes | Hereditary stomatocytosis, Rh null blood group, cold hemolysis |
Irreversibly sickled RBCs | Sickle cell syndromes |
Howell-Jolly bodies (nuclear remnants) | Hyposplenism, asplenia, dyserythropoietic anemias, severe iron deficiency |
Basophilic stippling (ribosomal aggregation) | Lead poisoning, thalassemias, severe iron deficiency |
Cabot’s rings | Lead poisoning, pernicious anemia |
Fragmented RBCs, Schistocytes | Microangiopathic hemolytic anemia, disseminated intravascular coagulation |
Crenated RBCs | Uremia, acute hepatic necrosis |
After the initial laboratory evaluation has been conducted, additional studies may be helpful to identify the physiological process resulting in anemia (Table 12.2). Reduced serum ferritin (<12 ng/mL) levels with an elevated total iron binding capacity (TIBC > 450 μg/mL) suggests iron deficiency anemia. An elevated lactate dehydrogenase level, a low haptoglobin level, and indirect hyperbilirubinemia point toward a hemolytic process. Other tests required for diagnostic confirmation include the osmotic fragility test (hereditary spherocytosis), hemoglobin electrophoresis (sickle cell disease, thalassemia, and other hemoglobin variants), RBC enzyme panel (G6PD and pyruvate kinase deficiency), direct and indirect antiglobulin test (autoimmune hemolytic anemia), and CD55/59 flow cytometric assay (Paroxysmal nocturnal hemoglobinuria).
Specific Causes of Anemia in Children
Anemia in the Newborn
Anemia in the neonate can be classified into three major categories: anemia due to blood loss, hemolysis, or decreased RBC production. Anemia as a result of blood loss can occur due to twin-to-twin transfusion, internal hemorrhage that occurs with a cephalohematoma or splenic rupture from a traumatic birth, an intraventricular hemorrhage that occurs in premature, low birth weight babies, or fetomaternal hemorrhage. Fetomaternal hemorrhage is diagnosed by the Kleihauer-Betke acid elution test which is based on the resistance of fetal hemoglobin to elution from the intact cell in an acid medium [10]. Fetal–maternal transfusion occurs in approximately 50% of pregnancies, but does not usually result in significant blood loss [11]. Additional risk factors for substantial blood loss in the neonate include rupture of the umbilical cord, placental abruption, placenta previa, placental tumors, and amniocentesis during the third trimester of pregnancy.
Immune hemolytic anemia occurs as a result of blood group incompatibility [Rh (D antigen), ABO, and minor blood group incompatibilities] between the mother and the fetus. Newborns with immune hemolytic anemia usually present with anemia, jaundice, and a positive Coomb’s test within the first 24 h of life. These infants need to be monitored closely with appropriate interventions (phototherapy, exchange transfusion) as indirect bilirubin levels can rise rapidly and lead to kernicterus. Although hemolytic anemia due to Rh incompatibility is usually severe, its incidence has decreased dramatically thanks to antenatal screening and the use of Rh immunoglobulin (RhoGam) in Rh-negative mothers. In contrast, hemolytic anemia secondary to ABO incompatibility is usually milder and occurs in the setting of a mother with blood Group O and an infant who has either Group A or B blood type. Other conditions that may manifest as hemolytic anemia in the newborn period include G6PD deficiency, hereditary spherocytosis, and thalassemia syndromes.
Anemia as a result of decreased RBC production in the neonate can occur due to physiological anemia of infancy or anemia of prematurity (AOP), Diamond-Blackfan anemia (discussed later in the chapter), and congenital infections. After birth, there is initially a decrease in RBC mass due to transition from a hypoxic state in utero to a hyperoxic state with increased tissue oxygenation. This process continues until oxygen delivery is inadequate which in turn stimulates erythropoiesis again. Due to this physiological adaptation to postnatal life, all term infants develop a normocytic and asymptomatic anemia (hemoglobin 9–11 g/dL) at 8–12 weeks of age. AOP, on the other hand, is an exaggerated physiological response, characterized by a normocytic, normochromic anemia with a decreased reticulocyte count and decreased serum erythropoietin (EPO) levels. The low hemoglobin concentration in these premature infants is due to a lack of third-trimester hematopoiesis and iron transport, suboptimal EPO level and response, shorter RBC life span and iatrogenic blood loss in relation to their body weight. The nadir of hemoglobin (7–9 g/dL) typically occurs by 4–8 weeks after birth and usually improves by 3–6 months of age [12]. The management of AOP includes optimal nutrition with iron supplementation and judicious use of blood sampling and blood transfusion. Routine use of recombinant EPO for the treatment of AOP is not recommended due to its limited benefit in reducing blood transfusions and exposure to blood donors, and because its early use (first 8 days after birth) can increase the risk of retinopathy of prematurity [13].
Pure Red Cell Aplasia
Diamond-Blackfan anemia (DBA) is a rare congenital pure red cell aplasia that results from defective ribosomal biosynthesis in bone marrow erythrocytic progenitors that leads to early apoptosis of RBCs [14]. Mutation in the RPS19 gene is the most common genetic defect and is present in 25% of patients with DBA . Most patients present during the first year of life with progressive severe macrocytic anemia, reticulocytopenia, elevated fetal hemoglobin (HbF) and adenosine deaminase (ADA) levels and a normocellular marrow with a paucity of erythroid precursors. About 30% of patients have associated anomalies such as short stature, dysmorphic facies, skeletal abnormalities (e.g., triphalangeal or bifid thumb), or congenital heart disease. Patients with DBA are at risk to develop secondary malignancies such as acute myeloid leukemia and osteosarcoma. Treatment options for DBA include glucocorticoids, chronic blood transfusions, and bone marrow transplantation for patients with severe anemia who are transfusion-dependent.
Transient erythroblastopenia of childhood (TEC) is an acquired form of pure red cell aplasia characterized by anemia with reticulocytopenia [15]. In contrast to DBA, patients with TEC are usually older (mean age 2–3 years), have normocytic anemia, normal HbF and ADA levels and do not have associated physical anomalies. Parvovirus B19 has been implicated in the pathogenesis of TEC , especially in the setting of underlying chronic hemolytic anemia such as hereditary spherocytosis or sickle cell disease. Most cases resolve spontaneously without any specific interventions and almost never recur. Blood transfusion may be required in patients who present with severe symptomatic anemia.
Iron Deficiency Anemia
Iron deficiency is the most common cause of anemia in children. Despite the increased efforts promoting breastfeeding and the use of iron-fortified formula, iron deficiency anemia (IDA) still occurs in approximately 3–7% of young children and 9% of adolescent females [16, 17]. Increased demand due to rapid growth coupled with excess intake of cow’s milk (low iron content) and heavy menstrual bleeding with inadequate iron intake are the major reasons for IDA in these populations, respectively. Since dietary deficiency of iron is uncommon in school age children and adolescent males, the presence of IDA in these patient groups warrants additional investigations to rule out sources of chronic blood loss (e.g., Meckel’s diverticulum). IDA is also the most common cause of microcytic, hypochromic anemia. Other important causes of microcytic anemia include alpha or beta thalassemia trait and lead poisoning.
IDA in children is associated with a variety of clinical manifestations including pica (irresistible craving for ice, paper, clay), restless leg syndrome, breath-holding spells, and poor neurodevelopmental outcome [18]. In addition to the microcytic, hypochromic appearance of the RBCs, IDA is characterized by a low reticulocyte count, an increased RBC distribution width (RDW) and a Mentzer index >13 [Mentzer index = MCV (fL)/RBC count (×106 per mm3)] [19]. In contrast, microcytic anemia in beta thalassemia trait is associated with a normal or slightly increased reticulocyte count, normal RDW, and a Mentzer index <13. An elevation of the reticulocyte count (after 1 week) and hemoglobin value (after 1 month) subsequent to the initiation of oral iron therapy confirms the diagnosis of IDA in most cases. Additional laboratory results such as a low serum ferritin level, increased total iron binding capacity, and low transferrin saturation percentage confirms iron deficiency and may be needed in certain situations. Standard oral iron therapy consists of the provision of 3–6 mg/kg/day of elemental iron in 2–3 divided doses for 1–2 months to correct the anemia, followed by an additional 2–3 months of therapy to increase hepatic iron stores. Ferrous sulfate is the most commonly used iron formulation used to treat IDA, but may cause gastrointestinal side effects. Newer iron preparations such as NovaFerrum™ are associated with fewer side effects and improved palatability, but may be expensive [18]. Intravenous iron therapy can be useful for patients with poor adherence or those who are intolerant to oral iron preparations.
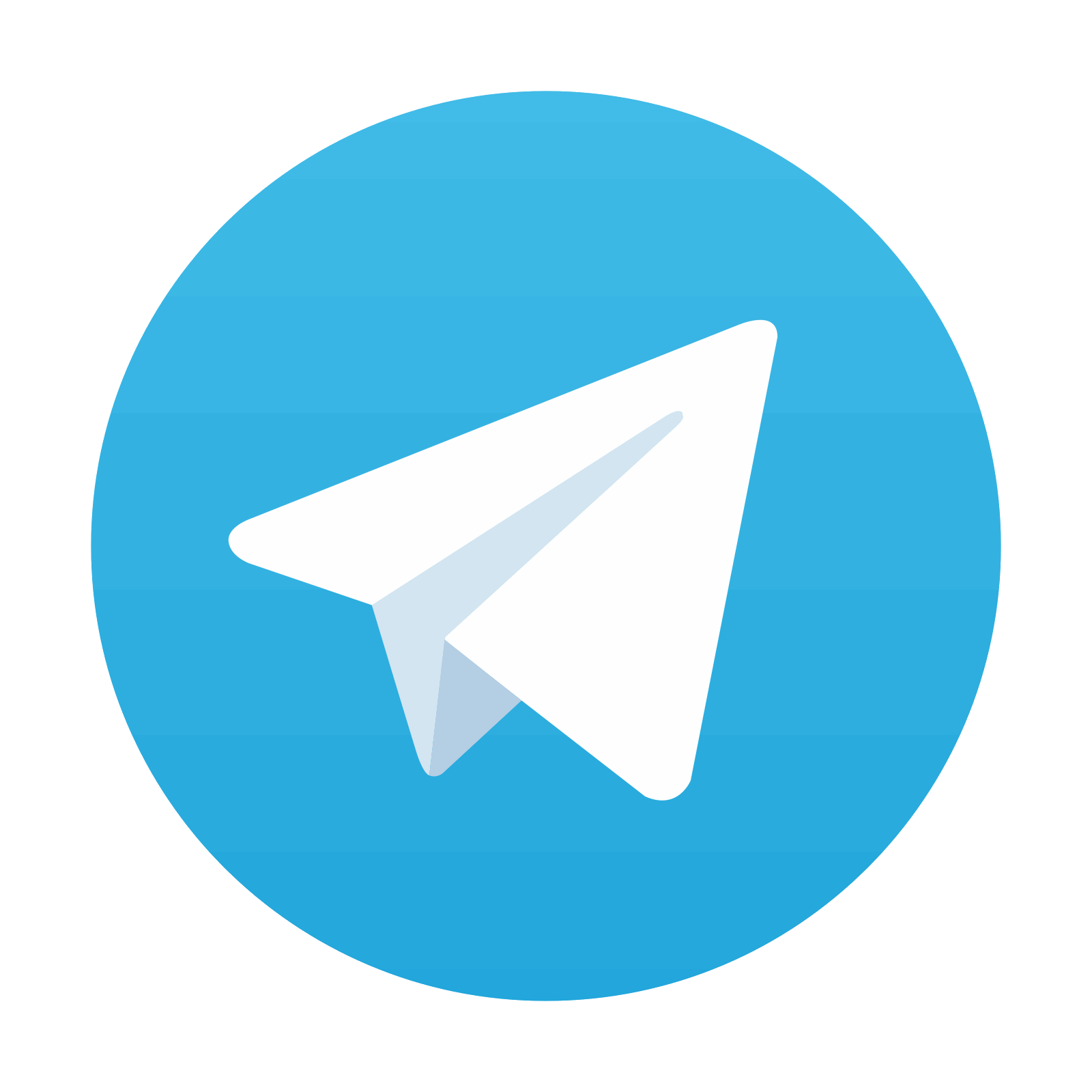
Stay updated, free articles. Join our Telegram channel

Full access? Get Clinical Tree
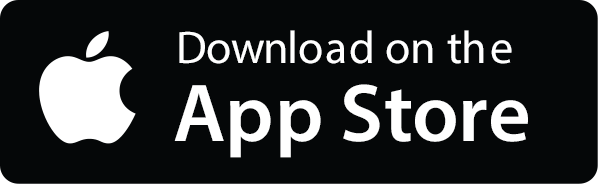
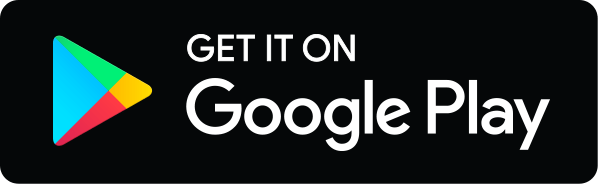