7 Electrosurgical Principles for Endoscopy
Louis M. Wong Kee Song and Michael B. Wallace
7.1 Introduction
Electrosurgery is an integral part of many therapeutic applications in gastrointestinal endoscopy. Electrosurgical units generate high-frequency alternating current (AC), which connects to accessories, such as polypectomy snares and sphincterotomes, for the purpose of cutting and/or coagulation. 1 An electrosurgical unit (ESU) converts low-frequency AC from a household electrical outlet (60 Hz in North America; 1 hertz [Hz] = 1 cycle per second) to > 300 KHz (▶Fig. 7.1). At these higher frequencies, there is insufficient time for cellular depolarization to occur before the current alternates again and undesirable neuromuscular stimulation (electric shock) is therefore avoided. Since the frequencies employed in electrosurgery are in the range of amplitude-modulated radio broadcasts, the term radiofrequency (RF) current is also used.

The amount and rate of heat produced at the cellular level through passage of RF current through tissue determine the end result. A high current concentration or density (measure of current applied per unit area) delivered by an electrosurgical knife or wire rapidly boils and vaporizes cells along the cleavage line, resulting in electrosurgical cutting. At low current density, tissue coagulation occurs since cells are heated more slowly and desiccate without any cutting. Several variables affect the current density and, consequently, the final tissue outcome (▶Fig. 7.2). Many of these variables are operator-dependent, such as selection of the ESU settings, type of accessory utilized, and technique and duration of application, so that the proportion of cells cut to those coagulated can be controlled to achieve the desired tissue effect. 2 , 3 An understanding of the basic electrosurgical properties and the interplay of these variables on the final tissue outcome are paramount for the safe and effective use of electrosurgery during endoscopy. Herein, the fundamental principles of electrosurgery and practical recommendations regarding utilization of electrosurgical devices for commonly performed procedures, such as polypectomy, sphincterotomy, and hemostasis, are highlighted.

The term cautery is often erroneously used during electrosurgery. 4 , 5 An example of a cautery device is the Heat Probe Unit (HPU-20, Olympus Corp., Tokyo, Japan), which uses an electrically heated probe that is then applied to tissue for coagulation (hemostasis) without any cutting (Video 7.1). The procedure is similar to hot iron branding and, unlike electrosurgery, there is no passage of electrical current through tissue.
7.2 Electrosurgical Principles
7.2.1 Electrical and Tissue Variables
The three interacting electrical properties of current (I), voltage (V), and resistance or impedance (R) affect temperature rise in tissue and are governed by Ohm’s law (▶Table 7.1). The terms resistance and impedance are applicable to direct current and AC, respectively.
Impedance is influenced by the water and electrolyte content of tissue. Tissues with high water content, such as blood vessels, pose less resistance to current flow than dehydrated tissues, such as bone and fat. Consequently, a lipomatous lesion is more difficult to transect with a snare relative to a nonfatty polyp at similar electrosurgical settings. Fibrosis and scarring also increase tissue impedance, which may necessitate adjustments in power and/or current waveform to achieve the desired effect. The buildup of charred tissue at the tip of a hemostatic probe or RF ablation catheter impedes current flow, hence the need to clean the electrodes intermittently during the procedure for efficient contact coagulation.
Voltage is the force that drives current through tissue. Voltage spikes must be greater than 200 peak volts (Vp) in order to generate adequate current density for electrosurgical cutting. Below 200 Vp, only tissue coagulation occurs regardless of the power setting. ESU outputs that maintain voltage constantly below the 200-Vp threshold are typical of bipolar applications for hemostasis, as well as the monopolar soft coagulation mode found in certain ESUs.
Current density ultimately determines the end result at the treated site and impacts the design or selection of the active electrode to suit a specific clinical purpose. Current density is lower when the RF energy extends over a greater volume of tissue, resulting in slower heating. Hence, the energy spread over the jaws of a flat hemostatic forceps (e.g., Coagrasper, Olympus Corp., Tokyo, Japan) or probe (e.g., TouchSoft, Genii Inc., St. Paul, MN) promotes coagulation as opposed to the high current density delivered along the thin wire of a sphincterotome that promotes cutting. Moreover, the operator can affect the current density by controlling the contact area between tissue and the active electrode.
Another operator-dependent variable is the power setting, which in turn impacts current density. As tissue heats, impedance rises, which decreases current flow and, consequently, power (▶Table 7.1). Through feedback tissue sensing, modern ESUs have outputs that are able to maintain power relatively constant over a range of measured impedances during current activation. These outputs with broad power-to-impedance curves are useful during certain procedures, such as polypectomy, where automatic sensing and power regulation reduce the potential for snare entrapment by providing adequate power during the entire resection process (▶Fig. 7.3). On the other hand, narrow power-to-impedance curves are characteristic of bipolar or monopolar outputs designed for contact hemostasis, with peak power delivered between 30 and 250 ohms (Ω) (▶Fig. 7.4). Tissues with resistances within this range, such as blood vessels, are targeted to receive the maximum power output that optimizes coagulation and, as tissue impedance increases with ongoing heating, the power output falls significantly so that the tissue does not overly desiccate and cause the accessory to stick. Although the manufacturer’s suggested power settings for specific procedures are a good starting point for a particular ESU, adjustments can be made based on the operator’s preference, technique, and desired outcome.


One variable that is entirely under the control of the operator is duration (time) of current application, which can impact the final outcome to a large degree. Taking into consideration that the total amount of energy (J) delivered equals power (W) ×time (s), how fast or slow the power is being deposited becomes important. The outcome of delivering 50 W of power for 2 seconds is quite different than that of delivering 20 W for 5 seconds, even though the total amount of heat energy delivered (100 J) is the same. Furthermore, if the time of application and power settings remain the same, either a continuous or modulated waveform will deliver an identical amount of total energy but with a very different outcome (see the section Electrosurgical Units and Waveforms).
7.2.2 Monopolar versus Bipolar Circuit
The terms monopolar and bipolar refer to the manner in which the electrosurgical circuit is completed. In a monopolar circuit, RF current oscillates from the active electrode (e.g., polypectomy snare) through the patient’s body via the path of least resistance to the inactive or dispersive electrode (electrosurgical pad), and returns to the ESU to complete the circuit (▶Fig. 7.5). The term dispersive electrode conveys an understanding that the energy exiting the patient through the pad is so much less concentrated that the risk of a burn injury at the pad site is reduced. Placement of the dispersive electrode close to the target site is recommended to keep the circuit as short as possible.

In a bipolar circuit, the active and return electrodes are in close proximity to each other, as illustrated by the bipolar hemostatic probe (e.g., Gold Probe, Boston Scientific Inc., Natick, MA) (▶Fig. 7.6). Current travels from the active to return electrodes through only a small volume of tissue in contact with the tip of the probe. An electrosurgical pad is not required for bipolar accessories. Except for hemostasis applications, bipolar devices are far less commercially available for endoscopic use than their monopolar counterparts.

7.3 Electrosurgical Units and Waveforms
ESUs enable the user to select from a menu of current waveforms a particular output, such as “Cut,” “Coag,” and “Blend.” An understanding of the waveform selected for a given ESU is important since manufacturer’s labeling of the outputs is not standardized and can be misleading (▶Table 7.2). For instance, an output labeled “Coag” with voltage spikes above 200 V is quite capable of electrosurgical cutting.
ESUs produce current outputs ranging from low-voltage, continuous sinusoidal waves to interrupted (modulated) waveforms with much higher voltages (▶Fig. 7.7). When voltage is maintained below 200 Vp, continuous waveforms produce superficial contact coagulation without electrosurgical cutting. These coagulation current outputs can be recognized by particular labels, such as “Soft Coag” or “TouchSoft” when used with monopolar devices and “BiCap” or “Bipolar” when used with bipolar accessories. With voltages between 200 and 600 Vp, continuous waveforms promote maximum cutting effects since they can rapidly produce high current densities along the active electrode to vaporize (cut) cells. Cells that are situated further away from the active electrode do not heat fast enough to vaporize, so they coagulate. Thus, even if a waveform is named “Pure Cut,” some coagulation will always be present along the margins of the cut. Although higher voltages deepen the coagulation spread along the cut margin, tissue charring becomes problematic when voltage is increased above 600 Vp for these continuous waves.

Modulated or interrupted waveforms heat tissue more slowly than continuous waves and are designed for outputs intended to produce varying amounts of coagulation, ablation, and hemostasis. By interrupting the current flow, tissue has a chance to cool, and the proportion of cells that desiccate without bursting increases. These modulated waveforms are recognized by particular labels, such as “Blend,” “Forced Coag,” and “Coag.” Peak voltages for modulated waveforms range from well over 200 Vp to 4,500 Vp, and even higher for noncontact fulguration modes. High-voltage modulated waveforms are used to ionize argon gas during noncontact argon plasma coagulation (APC).
One should not assume that a specific label, such as “Blend,” from one ESU corresponds to the same waveform output from another ESU with the same name. When reporting ESU settings in research studies and publications, quantitative descriptions of the waveforms based on the duty cycle and/or crest factor should be used to enable comparison and categorization of the qualitative waveform labels from different ESUs (▶Table 7.3). The duty cycle relates the percentage of time that the current is actually on during the activation period. For example, an output that is supplying current half the time and remains off the rest of the time has a 50% duty cycle. Continuous sine waves, often labeled as “Cut,” have a 100% duty cycle, whereas waveforms with duty cycles ranging from 20 to 80% often have labels such as “Swift Coag,” “Blend,” and “Blend Cut.” Waveforms named “Coag” typically have duty cycles of 6 to 12%, and although current is not flowing during much of the activation period, voltage spikes are well above 1,500 Vp when current does flow and therefore some electrosurgical cutting occurs especially with wire electrodes, such as polypectomy snares. Operators who utilize the “Coag” output and smoothly close a snare through polyp tissue are taking advantage of this cutting action along the snare wire, coupled with marked hemostasis promoted by the long rest periods in the cycle and the high voltage peaks that drive coagulation into tissue.
As an alternative to duty cycle, the crest factor can also be used to quantify waveforms. Crest factor takes into consideration average and peak voltages, as well as the frequency of the modulation, and becomes a better indicator of the expected depth and intensity of coagulation relative to the duty cycle for modulated waveforms. Continuous sinusoidal waveforms have a constant crest factor of 1.4 and in this instance the maximum peak voltage should also be stated. Highly modulated, high-voltage waveforms typical of “Coag” types (6% duty cycle) have crest factors in the range of 5 to 7. In general, high duty cycles correlate with low crest factors indicative of waveforms designed to produce more cutting with limited coagulation, whereas low duty cycles correlate with higher crest factors that predict increasing depth of coagulation and hemostasis (▶Table 7.3).
Specialized proprietary microprocessor outputs are available in some ESUs. For example, the “ENDO CUT” (Erbe Electromedizin, Tubingen, Germany) mode fractionates (pulses) the current output to promote controlled cutting of tissue and provide a well-defined and constant zone of coagulation during the entire cutting process. The “ENDO CUT” mode consists of an initial incision (cut) phase followed by phases of alternating cutting and coagulation current (▶Fig. 7.8), with the capability to adjust the duration (speed) of the cutting phase, the interval duration between cutting cycles, and the intensity of the coagulation effect. 6 , 7 Most of the ESUs listed in ▶Table 7.2 also have similar controlled pulse modes and all have various options for providing more or less coagulation along the cut zones.

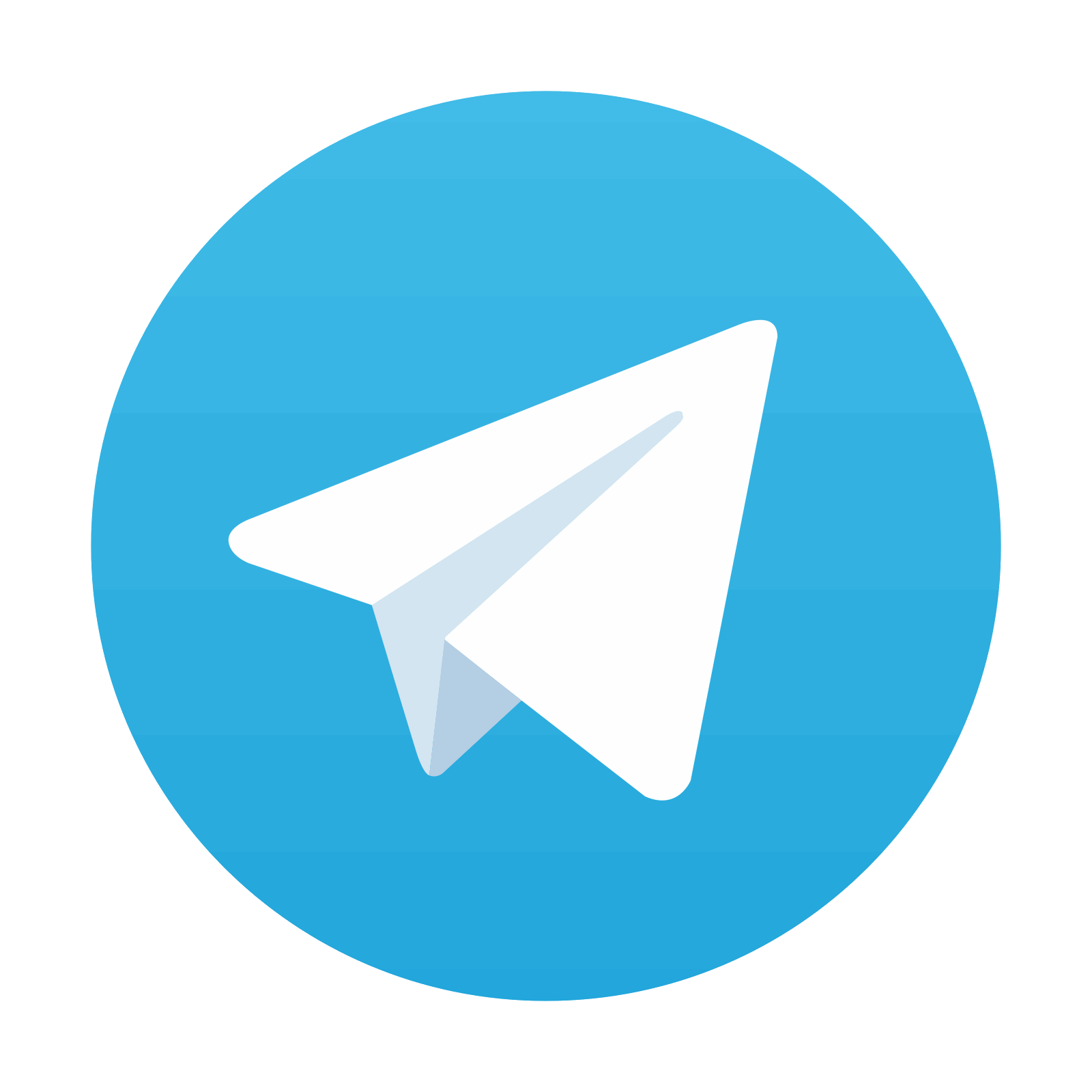
Stay updated, free articles. Join our Telegram channel

Full access? Get Clinical Tree
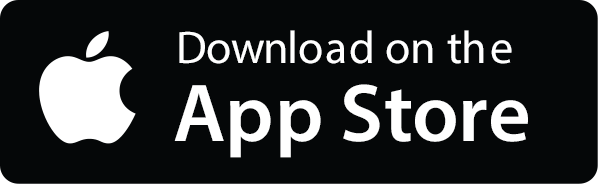
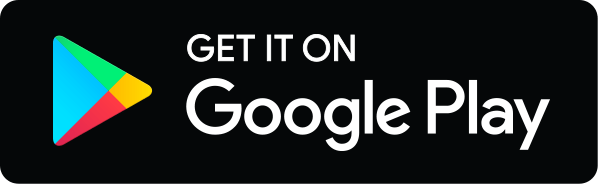