Outline
Introduction 125
Injury, Repair and Regeneration of the Kidney 125
Macrophage Phenotype 126
Macrophages in Different Disease Models 127
Classically Activated Macrophages in Adriamycin Nephropathy 127
Wound-healing (IL-4/IL-13-stimulated, M2a) Macrophages in Adriamycin Nephropathy 129
Regulatory (IL-10/TGF-β-stimulated, M2c) Macrophages in Adriamycin Nephropathy 131
Macrophages in Unilateral Ureteral Obstruction 133
Wound-healing (M2a) Macrophages in Diabetic Nephropathy 134
Macrophages in Acute Kidney Injury 134
Alternative Methods for Stimulating Macrophages 135
Adenovirus-transfected Macrophages 135
Alternative Sources of Macrophages 135
Bone Marrow-derived Macrophages 135
Regenerative Potential of Macrophages in Kidney Disease 136
Current Limitations in the Use of Macrophages as Cellular Therapy in Kidney Disease 136
Conclusion 136
Macrophages are key mediators in the pathogenesis of most types of primary and secondary human kidney diseases. There is emerging evidence that macrophages are pivotal players in the repair of tissue injury. Various strategies have been used to examine the role of macrophages in experimental renal injury, including adoptive transfer of macrophages, the systemic depletion of macrophages, blockade of molecules involved in monocyte recruitment into the inflamed kidney and gene modification of macrophages. This chapter will review the role of macrophages in renal injury and repair, and after a discussion about the different phenotypes of macrophages, show how experiments using adoptive transfer of different types of macrophages have increased our understanding of the biology of these cells.
Introduction
Macrophages are key mediators in the pathogenesis of most types of primary and secondary human kidney diseases. Many studies in both immune and non-immune-initiated models of kidney disease have shown a clear association between macrophage accumulation and the development of renal injury . However, there is emerging evidence that macrophages are pivotal players in the repair of tissue injury. Various strategies have been used to examine the role of macrophages in experimental renal injury including adoptive transfer of macrophages, the systemic depletion of macrophages, blockade of molecules involved in monocyte recruitment into the inflamed kidney and gene modification of macrophages . This chapter will review the role of macrophages in renal injury and repair, and after a discussion about the different phenotypes of macrophages, show how experiments using adoptive transfer of different types of macrophages have increased our understanding of the biology of these cells ( Table 7.1 ). Studies using stem cell progenitors of macrophages such as mesenchymal stem cells will not be included in this discussion (this is covered in Chapter 9 ).
Type of macrophage | Model | Method of delivery | Outcome on renal injury | Ref. |
---|---|---|---|---|
Classically activated macrophages | Adriamycin nephropathy | Intravenous | ↑ | |
IFN-γ and/or dexamethasone-stimulated macrophages | Nephrotoxic nephritis | Intravenous | ↑ | |
Wound healing (IL-4/IL-13-stimulated) macrophages | Adriamycin nephropathy | Intravenous | ↓ | |
Regulatory (TGF-β/IL-10-stimulated) macrophages | Adriamycin nephropathy | Intravenous | ↓ | |
Bone marrow-derived IL-4/IL-13-stimulated macrophages | Adriamycin nephropathy | Intravenous | No change | |
Wound-healing macrophages | Diabetic nephropathy (STZ in eNOS knockout C57BL/6 mice) | Intravenous | ↓ | |
Wound-healing macrophages | Diabetic nephropathy (STZ in wild-type BALB/c mice) | Intravenous | ↓ | |
Bone marrow-derived CD11b + CD18 + cells transfected with adenovirus containing IL-1 receptor antagonist | Anti-GBM glomerulonephritis | Intravenous | ↓ | |
Bone marrow-derived macrophages with adenovirus expressing I-κB | Nephrotoxic nephritis | Renal artery | ↓ | |
Bone marrow-derived macrophages with adenovirus expressing IL-10 | Nephrotoxic nephritis | Renal artery | ↓ | |
Alveolar macrophage cell line transfected with adenovirus expressing IL-4 | Nephrotoxic nephritis | Renal artery | ↓ | |
Bone marrow-derived mononuclear cells expressing endothelial cell markers | Thy1.1 glomerulonephritis | Renal artery | ↓ | |
Bone marrow-derived macrophages | Unilateral ureteral obstruction | Intravenous | ↓ |
Injury, Repair and Regeneration of the Kidney
Kidney disease, particularly in animal models, is characterized by an initial phase of injury, followed by a reparative phase and in some cases regeneration of kidney tissue. The injurious phase is characterized by tissue inflammation, while the reparative phase features the development of fibrosis. The regenerative phase involves proliferation of new cells, derived from either resident cells and/or bone marrow-derived (BMD) cells. This time-course model of renal disease has been elegantly demonstrated by Ricardo and colleagues , who studied mice that underwent unilateral ureteral obstruction (UUO) followed by reversal of UUO. Male C57BL/6J mice underwent UUO (using a vascular clamp) for 10 days followed by reversal of UUO (by release of clamp), and were then followed for 1, 2, 4 or 6 weeks. UUO was associated with tubulointerstitial fibrosis (increased collagen and hydroxyproline), tubular cell atrophy and dilatation, and macrophage accumulation. Reversal of UUO was associated with reduced macrophage numbers, decreased hydroxyproline and collagen expression, and restoration of glomerular filtration rate, as well as an increase in the number of viable tubules. This study showed the regenerative capacity of the kidney when the initial injury is removed.
Another study described a model of renal repair whereby mice underwent UUO for different lengths of time before ureteral reimplantation and contralateral nephrectomy. Seven weeks after reimplantation and contralateral nephrectomy, mean blood urea nitrogen levels and proteinuria were increased with longer duration of UUO. In contrast to the previous study, interstitial expansion, fibrosis and macrophage infiltration were similar in kidneys harvested after 10 days of UUO or following 10 weeks of ureter reimplantation, suggesting that the inflammatory process persisted despite relief of obstruction .
The vast majority of studies of macrophages in kidney disease have investigated the role of macrophages in the initial phases of injury and repair. Modulation of macrophage function or number before the initiation of renal injury allows the investigation of the ability of macrophages to abrogate renal injury. Several studies show that the initial degree of macrophage infiltration correlates with subsequent fibrosis, and that blockade of macrophage infiltration (by either depletion or reduction of recruitment) prevents the development of fibrosis . Ideally, investigating the capacity of macrophages to facilitate renal repair (rather than reduce injury) requires modulation of macrophage function and/or number after the injurious insult is removed. Therefore, animal models of kidney disease that use a toxic insult are ideally suited as the inciting event does not persist, unlike in immune models such as nephrotoxic nephritis. Models such as Adriamycin nephropathy, or UUO with reversal of UUO, are more suitable for this purpose. Therefore, interventions targeting infiltrating macrophages timed to occur after the toxic insult may be used to address the effect of macrophages on renal repair. Although studies using macrophages specifically for regeneration of kidney tissue are currently limited to BMD macrophages (discussed in this chapter and Chapter 9 ), this field is a highly active area of research .
Macrophage Phenotype
Macrophages are a diverse and dynamic population of cells that has the capacity to perform a wide range of critical functions. Macrophages can secrete a wide range of inflammatory factors, and thus are considered active participants rather than passive bystanders in mediating renal injury. The importance of macrophages in the pathogenesis of various kidney diseases has been investigated in human and animal studies. In human studies, macrophage infiltration is one of the hallmarks of severe and progressive renal disease, and the severity of injury correlates with the intensity of the macrophage infiltrate in patients with glomerulonephritis . Evidence of the pathogenic role of macrophages in various experimental renal diseases has been derived from studies demonstrating that depletion of macrophages or blockade of monocyte recruitment to the kidney reduces renal injury, and that repletion of macrophages restores renal injury. In sites of renal tissue injury, macrophages are activated towards a proinflammatory (M1) phenotype with the high expression of tumor necrosis factor-α (TNF-α), inducible nitric oxide synthase (iNOS) and interferon-γ (IFN-γ). These M1 cells directly damage resident cells through the generation of reactive oxygen species (ROS), nitric oxide (NO), complement factors and proinflammatory cytokines. M1 can also increase the production of extracellular matrix and vasculature through the expression of metalloproteinases and vasoactive peptides. Furthermore, M1 macrophages produce profibrogenic cytokines such as transforming growth factor-β (TGF-β) which promote interstitial fibroblast and myofibroblast proliferation, and their secretion of extracellular matrix (ECM) proteins that accumulate to form a scar.
However, macrophages are a diverse group of cells that also includes subpopulations that have a beneficial rather than harmful role in resolution and repair of tissue injury. Originally, activated macrophages were considered as cells that secreted inflammatory mediators and killed intracellular pathogens . These macrophages were also defined as classically activated macrophages (M1) following stimulation by lipopolysaccharide (LPS) or interferon (IFN-γ) in vitro. Now increasing evidence indicates that macrophages are also able to modulate immune responses through anti-inflammatory cytokines or regulation of T-cell function . For example, decidual macrophages have been shown to possess an immunoinhibitory function at the maternal–fetal interface. These cells express indoleamine 2,3-dioxygenase (IDO), which is able to reduce the ability of maternal T cells to mount an immune response against cells of the fetus . In vitro, macrophages incubated with interleukin-4 (IL-4), IL-13 or glucocorticoids can develop a regulatory phenotype (also known as alternatively activated or M2 macrophages) and produce anti-inflammatory cytokines and inhibit T-cell proliferation.
The anti-inflammatory phenotype described above includes a spectrum of macrophages which are heterogeneous in their mode of stimulation and expression of chemokines and surface molecules. Mosser and Edwards recently categorized anti-inflammatory macrophages into wound-healing macrophages (also known as M2a), which are activated by IL-4, and regulatory macrophages (also known as M2c), which are activated by Toll-like receptors (TLRs) in combination with other stimuli. However, macrophages associated with tumors can express characteristics of both wound-healing and regulatory macrophages, while macrophages in adipose tissue in obese individuals can convert from a wound-healing phenotype to a classically activated phenotype in response to adipocyte necrosis. Therefore, the paradigm of macrophage activation is changing. Instead of macrophages being regarded as fixed in phenotype, they are now regarded as responsive to changes in their environment, demonstrating plasticity in their nature. In Mosser and Edwards’ model, macrophages may be classified into classically activated (M1), wound-healing (M2a) or regulatory (M2c). Their phenotype may fall into a blend of any two of the above types (e.g. tumor-associated and obesity-associated macrophages) and may switch from one to another.
A key issue is what happens to macrophage phenotype during the course of kidney disease. Whereas depletion of macrophages early in disease may reduce fibrosis , depletion of macrophages later in disease may exacerbate it . In the late stages of kidney disease, the degree of fibrosis correlates inversely with the degree of macrophage infiltration , suggesting that the macrophage infiltrate in later disease is antifibrotic rather than profibrotic. Evidence for a change in macrophage phenotype during the course of disease also comes from an animal model of hepatic fibrosis induced by carbon tetrachloride in a transgenic mouse (CD11b-DTR) in which macrophages can be ablated by administration of diphtheria toxin. Conditional macrophage depletion when liver fibrosis was advanced resulted in reduced scarring and fewer myofibroblasts. Macrophage depletion during recovery, by contrast, led to a failure of matrix degradation. These data provide further evidence that functionally distinct subpopulations of macrophages exist in the same tissue and that these macrophages play critical roles in both the injury and recovery phases of inflammatory scarring.
Injury, Repair and Regeneration of the Kidney
Kidney disease, particularly in animal models, is characterized by an initial phase of injury, followed by a reparative phase and in some cases regeneration of kidney tissue. The injurious phase is characterized by tissue inflammation, while the reparative phase features the development of fibrosis. The regenerative phase involves proliferation of new cells, derived from either resident cells and/or bone marrow-derived (BMD) cells. This time-course model of renal disease has been elegantly demonstrated by Ricardo and colleagues , who studied mice that underwent unilateral ureteral obstruction (UUO) followed by reversal of UUO. Male C57BL/6J mice underwent UUO (using a vascular clamp) for 10 days followed by reversal of UUO (by release of clamp), and were then followed for 1, 2, 4 or 6 weeks. UUO was associated with tubulointerstitial fibrosis (increased collagen and hydroxyproline), tubular cell atrophy and dilatation, and macrophage accumulation. Reversal of UUO was associated with reduced macrophage numbers, decreased hydroxyproline and collagen expression, and restoration of glomerular filtration rate, as well as an increase in the number of viable tubules. This study showed the regenerative capacity of the kidney when the initial injury is removed.
Another study described a model of renal repair whereby mice underwent UUO for different lengths of time before ureteral reimplantation and contralateral nephrectomy. Seven weeks after reimplantation and contralateral nephrectomy, mean blood urea nitrogen levels and proteinuria were increased with longer duration of UUO. In contrast to the previous study, interstitial expansion, fibrosis and macrophage infiltration were similar in kidneys harvested after 10 days of UUO or following 10 weeks of ureter reimplantation, suggesting that the inflammatory process persisted despite relief of obstruction .
The vast majority of studies of macrophages in kidney disease have investigated the role of macrophages in the initial phases of injury and repair. Modulation of macrophage function or number before the initiation of renal injury allows the investigation of the ability of macrophages to abrogate renal injury. Several studies show that the initial degree of macrophage infiltration correlates with subsequent fibrosis, and that blockade of macrophage infiltration (by either depletion or reduction of recruitment) prevents the development of fibrosis . Ideally, investigating the capacity of macrophages to facilitate renal repair (rather than reduce injury) requires modulation of macrophage function and/or number after the injurious insult is removed. Therefore, animal models of kidney disease that use a toxic insult are ideally suited as the inciting event does not persist, unlike in immune models such as nephrotoxic nephritis. Models such as Adriamycin nephropathy, or UUO with reversal of UUO, are more suitable for this purpose. Therefore, interventions targeting infiltrating macrophages timed to occur after the toxic insult may be used to address the effect of macrophages on renal repair. Although studies using macrophages specifically for regeneration of kidney tissue are currently limited to BMD macrophages (discussed in this chapter and Chapter 9 ), this field is a highly active area of research .
Macrophage Phenotype
Macrophages are a diverse and dynamic population of cells that has the capacity to perform a wide range of critical functions. Macrophages can secrete a wide range of inflammatory factors, and thus are considered active participants rather than passive bystanders in mediating renal injury. The importance of macrophages in the pathogenesis of various kidney diseases has been investigated in human and animal studies. In human studies, macrophage infiltration is one of the hallmarks of severe and progressive renal disease, and the severity of injury correlates with the intensity of the macrophage infiltrate in patients with glomerulonephritis . Evidence of the pathogenic role of macrophages in various experimental renal diseases has been derived from studies demonstrating that depletion of macrophages or blockade of monocyte recruitment to the kidney reduces renal injury, and that repletion of macrophages restores renal injury. In sites of renal tissue injury, macrophages are activated towards a proinflammatory (M1) phenotype with the high expression of tumor necrosis factor-α (TNF-α), inducible nitric oxide synthase (iNOS) and interferon-γ (IFN-γ). These M1 cells directly damage resident cells through the generation of reactive oxygen species (ROS), nitric oxide (NO), complement factors and proinflammatory cytokines. M1 can also increase the production of extracellular matrix and vasculature through the expression of metalloproteinases and vasoactive peptides. Furthermore, M1 macrophages produce profibrogenic cytokines such as transforming growth factor-β (TGF-β) which promote interstitial fibroblast and myofibroblast proliferation, and their secretion of extracellular matrix (ECM) proteins that accumulate to form a scar.
However, macrophages are a diverse group of cells that also includes subpopulations that have a beneficial rather than harmful role in resolution and repair of tissue injury. Originally, activated macrophages were considered as cells that secreted inflammatory mediators and killed intracellular pathogens . These macrophages were also defined as classically activated macrophages (M1) following stimulation by lipopolysaccharide (LPS) or interferon (IFN-γ) in vitro. Now increasing evidence indicates that macrophages are also able to modulate immune responses through anti-inflammatory cytokines or regulation of T-cell function . For example, decidual macrophages have been shown to possess an immunoinhibitory function at the maternal–fetal interface. These cells express indoleamine 2,3-dioxygenase (IDO), which is able to reduce the ability of maternal T cells to mount an immune response against cells of the fetus . In vitro, macrophages incubated with interleukin-4 (IL-4), IL-13 or glucocorticoids can develop a regulatory phenotype (also known as alternatively activated or M2 macrophages) and produce anti-inflammatory cytokines and inhibit T-cell proliferation.
The anti-inflammatory phenotype described above includes a spectrum of macrophages which are heterogeneous in their mode of stimulation and expression of chemokines and surface molecules. Mosser and Edwards recently categorized anti-inflammatory macrophages into wound-healing macrophages (also known as M2a), which are activated by IL-4, and regulatory macrophages (also known as M2c), which are activated by Toll-like receptors (TLRs) in combination with other stimuli. However, macrophages associated with tumors can express characteristics of both wound-healing and regulatory macrophages, while macrophages in adipose tissue in obese individuals can convert from a wound-healing phenotype to a classically activated phenotype in response to adipocyte necrosis. Therefore, the paradigm of macrophage activation is changing. Instead of macrophages being regarded as fixed in phenotype, they are now regarded as responsive to changes in their environment, demonstrating plasticity in their nature. In Mosser and Edwards’ model, macrophages may be classified into classically activated (M1), wound-healing (M2a) or regulatory (M2c). Their phenotype may fall into a blend of any two of the above types (e.g. tumor-associated and obesity-associated macrophages) and may switch from one to another.
A key issue is what happens to macrophage phenotype during the course of kidney disease. Whereas depletion of macrophages early in disease may reduce fibrosis , depletion of macrophages later in disease may exacerbate it . In the late stages of kidney disease, the degree of fibrosis correlates inversely with the degree of macrophage infiltration , suggesting that the macrophage infiltrate in later disease is antifibrotic rather than profibrotic. Evidence for a change in macrophage phenotype during the course of disease also comes from an animal model of hepatic fibrosis induced by carbon tetrachloride in a transgenic mouse (CD11b-DTR) in which macrophages can be ablated by administration of diphtheria toxin. Conditional macrophage depletion when liver fibrosis was advanced resulted in reduced scarring and fewer myofibroblasts. Macrophage depletion during recovery, by contrast, led to a failure of matrix degradation. These data provide further evidence that functionally distinct subpopulations of macrophages exist in the same tissue and that these macrophages play critical roles in both the injury and recovery phases of inflammatory scarring.
Macrophages in Different Disease Models
Classically Activated Macrophages in Adriamycin Nephropathy
Although the expression and behavior of classically activated macrophages have been studied extensively, the pathogenic importance of both macrophage number and activation status in kidney disease is not well understood. Significantly, classically activated macrophages can incite and exacerbate inflammation and fibrosis and thus have the potential to impair renal tissue repair.
A study conducted using adoptive transfer of macrophages by the present authors’ research group explored the effect of activation status of macrophages in inducing renal injury . This study was carried out using the Adriamycin (doxorubicin) nephrosis (AN) model of kidney disease in severe combined immunodeficient (SCID) mice. AN is a robust experimental model of human focal segmental glomerulosclerosis, characterized by changes in both tubulointerstitial and glomerular compartments. It can be induced in immunocompetent mice , and also in SCID mice that are homozygous for an autosomal recessive mutation that leads to an absence of lymphocytes . The use of SCID mice allows the study of the role of macrophages in an in vivo kidney disease model without other potential interactions due to lymphocytes.
In Wang’s study , SCID mice with AN were treated 6 days after Adriamycin injection with either resting (1 × 10 6 to 5 × 10 6 ) or activated (1 × 10 3 to 1 × 10 6 ) macrophages (M1) (injected intravenously), and the effects on kidney injury were examined after 4 weeks. Transfusion with resting macrophages (M0) in doses between 1 and 5 million cells per mouse did not exacerbate renal injury and fibrosis in comparison with control AN mice. However, transfusion with as few as 10 4 activated macrophages per mouse worsened renal injury and fibrosis. The injury caused by 10 5 and 10 6 activated macrophages was not different from that caused by 10 4 activated macrophages. Similarly, both serum creatinine and urinary protein excretion were significantly worse following transfusion with activated macrophages in doses as low as 1 × 10 4 (but not 10 3 ) cells per mouse, but were unaffected by resting macrophages in doses up to 5 × 10 6 cells per mouse in normal or AN mice. This finding emphasizes the importance of macrophage activation status in causing or exacerbating kidney injury . Similar potency has been shown of IL-4/IL-13-activated macrophages (M2a), which were protective against injury in AN in SCID mice in doses as low as 10 4 per mouse .
Activation status of macrophages has also been examined by Nikolic-Paterson’s group . Exposure of macrophages to IFN-γ for 18 h (but not 3 h) before adoptive transfer caused a two-fold increase in the degree of proteinuria and glomerular cell proliferation compared with unstimulated cells. This was due to an increase in the number of transferred macrophages within the glomerulus and a significant increase in degree of renal injury per transferred glomerular macrophage .
An interesting finding in Wang’s study was that transfusion of resting macrophages did not exacerbate kidney injury in comparison to that of untransfused AN mice, even at doses 500 times higher than those at which activated macrophages exacerbated injury. This may be related to the properties of resting macrophages. One study examined the properties and responsiveness to cytokines of macrophages purified from normal and nephritic glomeruli and found that macrophages from normal glomeruli did not generate nitric oxide spontaneously but did so only after treatment with IFN-γ and TNF-α . Another reason may be related to the impaired ability of resting macrophages (M0) to accumulate in sites of (renal) inflammation. However, this result contrasts with that of Nikolic-Paterson’s group, who reported that transfusion of resting macrophages did increase proteinuria and mesangial cell proliferation in anti-glomerular basement membrane (GBM) nephritis. The explanation for these contrasting results may relate to differences in the models, with AN being primarily a model of innate immunity and anti-GBM nephritis of adaptive immunity in which macrophages act as an antigen-presenting cell to T cells. Moreover, under appropriate conditions, resting macrophages may serve as a reservoir for activated macrophages. This has been demonstrated in a two-shot model of Thy1.1 nephritis, in which resting macrophages become activated by interferon only after a second dose of anti-Thy1.1 antibody .
The mechanisms underlying the exacerbation of renal injury by activated macrophages have been examined. First, activated macrophages express high levels of major histocompatibility complex (MHC) II and CD86, which are important molecules in the initiation of adaptive immune responses. Second, activated macrophages express inflammatory mediators including nitric oxide and TNF-α, which are known to directly cause renal injury. It is noteworthy that macrophages isolated from AN kidney more than 3 weeks after adoptive transfer still exhibit upregulated inflammatory cytokine expression similar to that of the macrophages immediately after initial activation . Studies from Rees’ group demonstrated that macrophages separated from a focus of immunologically mediated inflammation in nephrotoxic nephritis were programmed in vivo, and were unresponsive to activating cytokines such as IL-4, TGF-β and TNF-α. Their studies raise the question of whether macrophage function alters with time during the resolution of inflammation. Thus, transfused macrophages appear to maintain their activation status within the inflammatory focus during the progression of renal injury .
To determine the fate of adoptively transferred macrophages, cells were labeled with the fluorescent tracking dye DiI. Fluorescently labeled cells were distributed to kidney, spleen and liver of AN mice 24 h after transfer. Very few of these cells were seen in spleen and liver at day 14 (8 days post-transfusion) and none were detectable at day 28 in AN mice transfused with activated macrophages, whereas in AN mice transfused with resting macrophages, their number in liver and spleen remained constant. In contrast, transfused activated macrophages from a dose of 1 × 10 4 accumulated progressively in kidneys up to day 28 after Adriamycin. The absolute number of transfused resting macrophages found in kidneys of AN mice, even with transfusion of 5 × 10 6 cells, was much less than the number of transfused activated macrophages, even with transfusion of as few as 1 × 10 4 cells. Macrophage recruitment to the kidney is an important step in initiation of inflammation and tissue destruction. Therefore, activated macrophages are more easily recruited into damaged kidney than are resting macrophages. In addition, transfused activated macrophages accumulate progressively at the site of injury [the kidney], rather than in other unaffected organs.
To clarify why activated macrophages accumulated more effectively in sites of injury than resting macrophages, macrophage mobility in response to CCL2 was examined . Activated macrophages demonstrated greater mobility in response to CCL2 than resting macrophages. These data suggest that enhanced responsiveness of activated macrophages to chemotactic stimuli may be a key factor determining their accumulation at sites of injury. Moreover, in that study, activated macrophages expressed higher levels of CCR2, the receptor for CCL2, than resting macrophages. Izikson et al. reported that CCR2 expression is consistent with the ability of monocytes to traffic to sites of inflammation. Furthermore, Xu et al. also showed that inflammatory monocyte recruitment to inflamed tissue was coincident with a reduction in CCR2 + cells in circulation after adoptive transfer of BMD macrophages.
In conclusion, activated macrophages, but not resting macrophages, exacerbate renal injury in murine AN. Activation status of macrophages determines whether and to what extent macrophages cause or exacerbate renal injury. Therapeutic targeting of pathogenic activated macrophages (M1 phenotype) rather than all macrophages is likely to be an effective approach to treating progressive renal disease.
Wound-healing (IL-4/IL-13-stimulated, M2a) Macrophages in Adriamycin Nephropathy
The precedent from other tissues such as lung and skin provides a convincing reason for believing that macrophages play an important role in tissue remodeling and repair. A subset of macrophages that secrete anti-inflammatory cytokines, promote angiogenesis and play a positive role in wound healing and tissue remodeling have been generally referred to as alternatively activated macrophages (M2). M2 cells induced by exposure to IL-4 and IL-13 (M2a) and deactivating cytokines such as IL-10 and TGF-β (M2c) are thought to suppress immune responses and promote tissue remodeling. M2 macrophages have been studied extensively in vitro with respect to their suppressive activity, secretion of anti-inflammatory cytokines and ability to modulate wound healing and angiogenesis. However, whether or not M2 can be potent immune regulators in vivo and used as therapy for kidney disease has not been clear until recently.
The adoptive transfer of both non-activated and LPS/IFN-γ activated macrophages can worsen inflammation in animal models of acute renal injury . In contrast, inhibition of nuclear factor-κB (NF-κB) by adenoviral I-κB (I-κB) in macrophages leads to an anti-inflammatory phenotype, which has been shown to ameliorate renal injury in an animal model of glomerulonephritis . In addition, macrophages transfected with anti-inflammatory cytokines have been shown to reduce renal injury in animal models of glomerulonephritis (see below).
Adoptive transfer of cytokine-programmed wound-healing macrophages has the therapeutic potential to reduce renal injury . To determine the effects of wound-healing macrophages in renal disease models independent of endogenous T and B cells, adoptive transfer of macrophages into SCID mice was performed. In this study, macrophages were isolated from spleens of BALB/c mice and stimulated with IL-4 and IL-13 to induce a wound-healing phenotype. These macrophages were then transfused into SCID mice with AN. In these studies, macrophages provided marked protection against both structural and functional damage in AN . Renal injury in AN was marked and characterized by glomerular sclerosis, tubular atrophy and interstitial fibrosis with substantial mononuclear cell infiltration. Renal injury and fibrosis were significantly reduced in mice transfused with wound-healing macrophages compared with control AN mice ( Fig. 7.1 ). Similarly, serum creatinine and urine protein were significantly improved with M2a macrophage transfusion compared with untransfused AN mice. This study provides direct evidence that ex vivo modulation of macrophages by cytokines to an anti-inflammatory phenotype can be an effective strategy for treating experimental chronic inflammatory renal disease. In comparison to other macrophage strategies for ameliorating renal injury in animal models such as genetic modification or chemokine blockade, cytokine manipulation of macrophages to the M2 phenotype provides many of the advantages without the associated risks. The advantages of this method include the simplicity of ex vivo macrophage modulation by cytokines, and the effectiveness of intravenous administration without the need for specialized delivery. Most important is the fact that it avoids limitations of gene therapy which include technical difficulties of gene delivery and risks of infection associated with the viral vector.

The potency of M2a is underscored by the observation that as few as 1 × 10 4 M2 are required to protect against renal injury . These experiments delineate a lymphocyte-independent mechanism of protection by M2a. Injected M2a homed to kidneys rather than secondary lymphoid organs and retained their phenotype beyond 4 weeks postinjection, similar to the behavior of adoptively transfused M1 macrophages in AN. The protective effect of M2a was associated with reduced accumulation and downregulated chemokine and inflammatory cytokine expression of the infiltrating host macrophages. This study provides direct evidence that the regulatory effects of M2a are target specific, robust and effective for treating experimental chronic inflammatory renal disease .
Another relevant issue is the stability of macrophage phenotype after initial cytokine exposure and transfusion in vivo, an important consideration in the reliability of M2a as a therapeutic approach for kidney diseases. One study has suggested that the microenvironment can reprogram the functional phenotype of macrophages . However, M2a phenotype (expression of CCL17 and mannose receptor), once produced ex vivo, can be maintained for up to 4 weeks in in vitro culture ( Fig. 7.2 ). M2 phenotype and function can also persist in the kidney in vivo, as shown by the following data: (i) M2 macrophages trafficked to inflamed kidney rather than to other unaffected organs, and accumulated progressively in inflamed kidney; (ii) markers of M2 phenotype including CCL17 and mannose receptor were also maintained in transfused IL-4/IL-13-stimulated macrophages for at least 4 weeks after transfusion; and (iii) in inflamed kidney, transfused M2a decreased the number of host macrophages and their expression of inflammatory cytokines including TNFα and CCL2 .

In conclusion, adoptive transfer of M2a macrophages in the AN model results in strong protection against renal structural and functional injury. M2a preferentially target the inflamed kidney, and the regulatory effects of M2a are sustained in vitro and in vivo. Thus, macrophage modulation ex vivo to M2a is an effective and reliable strategy for treating experimental chronic kidney disease.
Regulatory (IL-10/TGF-β-stimulated, M2c) Macrophages in Adriamycin Nephropathy
The phenotype of alternatively activated macrophages (M2) is diverse and characterized by anti-inflammatory and regulatory properties. Recently, M2 have been subdivided into M2a, which develop in vitro in response to IL-4 or IL-13, M2b in response to immune complexes and IL-1 or LPS, and M2c in response to IL-10, TGF-β or glucocorticoids . M2c are usually regarded as deactivating macrophages because of their ability to downregulate proinflammatory cytokines and remodel tissue. The primary role of M2c appears to be modulation of the immune response through secretion of regulatory cytokines such as IL-10 and TGF-β.
IL-10 plays a critical role in limiting the duration and intensity of immune and inflammatory reactions. In macrophages, IL-10 inhibits production of proinflammatory cytokines such as TNFα, IL-6 and IL-12, and impairs antigen presentation by monocytes or macrophages via downregulation of MHC II and costimulatory molecules.
TGF-β is a pleiotropic cytokine which mediates a wide variety of effects on cellular differentiation, activation and proliferation. In macrophages and monocytes TGF-β regulates activation, cytokine production, host defense and chemotaxis. TGF-β acts as a negative regulator of CD163-expressing macrophages and inhibits LPS-induced macrophage production of the proinflammatory cytokines TNFα, IL-1α and IL-18 .
M1, M2a and M2b have been defined by a number of specific biomarkers: iNOS, Cox2 and proinflammatory cytokines for M1; mannose receptor, arginase, CCL17, YM1 and FIZZ1 for M2a; and IL-10 and other TLR-related molecules for M2b. However, there is less certainty about specific biomarkers for M2c. Recently, this group has identified B7-H4 as a specific functional biomarker of M2c. B7-H4, an inhibitory molecule, is a recently discovered member of the B7 family. It has been reported that B7-H4 is expressed in tumor-associated macrophages in human ovarian carcinoma . It has also been shown that regulatory T lymphocytes (Tregs) can convey suppressive activity to macrophages by stimulating B7-H4 expression through IL-10 . A striking finding in this study was that M2c, but not M2a, could convey suppressive activity to T cells via B7-H4, resulting in induction of Tregs. Thus, B7-H4 is potentially a specific biomarker and functional marker of M2c .
The effect of transfused M2c was examined in an immunocompetent model of AN. To examine the protective effects of M2c in murine AN, macrophages were separated from spleen and cultured for 48 h with TGF-β and IL-10. M2c cells (1 × 10 6 /mouse) were infused at day 5 after Adriamycin administration. Age-matched normal mice and mice injected with Adriamycin alone were used as normal and disease controls. In vivo, adoptive transfer of M2c significantly reduced tubular atrophy, interstitial expansion and glomerulosclerosis in AN. M2c also reduced proteinuria and increased creatinine clearance significantly. This study demonstrated that M2c were able to diminish inflammatory infiltrates and renal structural and functional injury, as shown for M2a in SCID mice with AN. Moreover, it appeared that M2c were more effective than M2a in reducing renal histological and functional injury . M2c not only deactivated and reduced inflammatory immune cells including CD4 + T cells, CD8 + T cells and renal macrophages, but also reduced tubular damage and glomerulosclerosis. These data demonstrate that macrophages polarized ex vivo to protective (wound-healing and regulatory) phenotypes may be a useful therapeutic strategy for chronic renal inflammatory disease .
M2c were found to express high levels of B7-H4, whereas M2a did not. B7-H4 is a member of the B7 family of costimulatory molecules. In the B7 family, the classic costimulatory molecules B7-1 and B7-2 provide critical positive costimulatory signals on interaction with CD28 on resting T cells, whereas B7-H4 is a negative regulator of T-cell responses in vitro that inhibits T-cell proliferation, cell cycle progression and cytokine production. Although mouse B7-H4 ligation of T cells has an inhibitory effect on T-cell activation, the regulatory mechanisms of B7-H4 remain to be defined. A striking finding was that M2c could convey suppressive activity to T cells via B7-H4, resulting in the inhibition of T-cell proliferation. Furthermore, M2c were also able to induce Tregs. This function of M2c was demonstrated both in vitro (partially via B7-H4) and in vivo (in renal draining lymph nodes).
The ability to promote differentiation of Tregs by macrophages has also been reported for lamina propria macrophages . Denning et al. described a population of CD11b + F4/80 + CD11c − macrophages in the lamina propria that express several anti-inflammatory molecules, including IL-10, but few if any proinflammatory cytokines. These macrophages can induce Foxp3 + Tregs in the lamina propria and inhibit IL-17 production by lamina propria CD11b + dendritic cells. Recently, Murai et al. showed that IL-10 secreted by a unique subset of macrophages in the lamina propria is responsible for maintaining Foxp3 expression in Tregs in inflammatory conditions. Lamina propria macrophages produce IL-10, which signals via IL-10 receptor-β on Tregs to maintain Foxp3 expression and Treg suppressive function. These IL-10-derived signals are essential for the maintenance of Foxp3 expression in inflamed but not steady-state conditions, perhaps because of the suppressive effects of proinflammatory cytokines on Foxp3 expression. It is plausible that unique bacterial flora, perhaps through TLR signaling, are responsible for the induction of IL-10 in these macrophages.
This group found that M2c are able to deactivate renal inflammatory (M1) macrophages. This ability of M2c has been shown in coculture with M1, and was further demonstrated in the AN model by their deactivation of endogenous renal macrophages. This novel finding of deactivation of inflammatory macrophages in kidney by M2c may explain their protective effects against renal injury .
An interesting question for further study is whether transfused macrophages interact with renal proinflammatory dendritic cells (DCs). Renal dendritic cells (rDCs) were first described as MHC class II-positive cells with stellate and “mononuclear phagocyte” morphology in the rat renal interstitium, and have been isolated by using their membrane expression of CD11c. Furthermore, in the human kidney, there are two different DC subsets in the tubulointerstitium, CD68 + BDCA-1 + DC-SIGN + / − HLA-DR + myeloid DCs and BDCA-2 + HLA-DR + lymphoid DCs. Soos et al. demonstrated that CX3CR1 rDCs form an organ-spanning network within the entire interstitial and mesangial space. rDCs have been shown to accumulate in peritubular and periglomerular interstitium in human lupus nephritis and glomerulonephritis and rat crescentic glomerulonephritis , indicating that DCs are recruited from the circulation into the site of renal injury. Dong et al. showed that rDCs are a source of proinflammatory mediators in acute urinary obstruction and facilitate accumulation of IL-17 expressing T cells in the kidney. There is also evidence that recruited kidney DCs provide a mechanistic link between glomerular injury and the spread of damage to the tubulointerstitium . Moreover, it has been shown that interstitial macrophages are capable of altering DC function in lung and intestinal disease . Therefore, the interactions between macrophages and DC have potential importance in renal inflammatory disease.
A major consideration in evaluating M2c as a therapeutic approach is the potential for phenotype switch in vivo. Macrophages, unlike T cells which undergo irreversible differentiation upon antigen stimulation, can retain their plasticity and respond to different environmental signals. Numerous studies have examined the stability and longevity of activated macrophages within the host. Several studies have indicated that the phenotype of macrophages can change in vivo over time; for example, it was reported that macrophages in the earliest stages of cancer resembled classically activated macrophages, yet, with tumor growth, macrophages developed a regulatory phenotype . Another example is the observation that macrophages in adipose tissue of non-obese humans have a wound-healing phenotype, yet can switch to a proinflammatory phenotype in obese individuals . It is unclear whether phenotypic deviation can occur in transfused anti-inflammatory macrophages used to treat various diseases. This is very important as transfused macrophages may cause more harm than benefit if they switch from an anti-inflammatory to a proinflammatory phenotype. Importantly, it was previously shown that macrophage phenotype after initial ex vivo cytokine modulation was stable in vitro and little changed in vivo despite microenvironmental changes during the course of disease . However, that study was performed in SCID mice, which are deficient in T and B cells, and the stability of the M2 phenotype could possibly be explained by the lack of cognate immune cells and their secreted proinflammatory cytokines. A more recent study examined the effect of transfused macrophages into an immunocompetent model of AN and found that the phenotype of transfused macrophages did drift, but not towards a distinct M1 or M2 phenotype. The suppressive features of transfused M2c were partially lost, as shown by their reduced expression of anti-inflammatory genes, such as TGF-β, arginase and mannose receptor. The expression of proinflammatory genes by M2c was elevated at weeks 1 and 2, but diminished at week 3. This phenomenon indicates that transfused M2c do not convert to a typical M1 phenotype, and that their phenotype drifts across different stages of disease .
Another concern in using M2c as a therapeutic approach is their potential profibrotic effect. M2c express high levels of TGF-β , a growth factor linked to renal fibrosis. TGF-β acts on a transmembrane receptor through Smad proteins to regulate key profibrotic genes such as collagen. Macrophage-derived TGF-β may also promote fibrosis by paracrine activation of matrix-producing myofibroblasts and promotion of epithelial–mesenchymal transition of tubular epithelial cells (TECs) into myofibroblasts . However, current studies by the present authors in AN mice treated with M2c showed a reduction rather than promotion of renal fibrosis, arguing against this concern. Possible reasons underlying the reduction of fibrosis by M2c include their deactivation of host macrophages and reduction of other profibrotic stimuli. IL-10 and TGF-β secreted by M2c suppress immune responses, dampen inflammation and promote tissue remodeling in early stages of disease, and thereby can further reduce fibrosis. Another possible explanation for the observed reduction in fibrosis is that transfused M2c, after executing their anti-inflammatory effects, decrease their secretion of TGF-β. This group demonstrated that TGF-β expression by transfused M2c in inflamed kidney did reduce progressively from week 1, towards levels seen in resting macrophages. However, IL-10 expression of transfused M2c remained at high levels throughout the observed course of disease. These results suggest that transfused M2c may have a considerable ability to limit inflammation and reduce profibrotic effect as the disease progresses.
In vivo proliferation is another potential concern in the therapeutic use of transfused M2c. It has been reported that fetal macrophages have a high proliferative capacity, but that the proliferative capacity of macrophages declines once permanent hematopoiesis is established . The present authors demonstrated that M2c isolated from the spleen do not proliferate in vitro for up to 3 weeks, nor do transfused M2c isolated from kidneys of AN mice.
A key question is whether M2c exist in various kidney diseases. Several studies have shown some clues to the existence of M2c in vivo. Tumor-associated macrophages (TAMs) are a paradigm for polarized M2 mononuclear phagocytes. In the tumor microenvironment, IL-10, TGF-β and PGE 2 are produced by a variety of tumor cells and by TAM themselves. IL-10 promotes the differentiation of monocytes to mature macrophages and blocks their differentiation to DCs. In addition, IL-10 induces TAM to express M2c-related functions, such as scavenging debris, angiogenesis, tissue remodeling and repair. TAMs express high levels of the mannose receptor (MR) and are poor at presenting antigen. They also have an IL-10 high IL-12 low cytokine repertoire. In addition, CD11b + F4/80 + macrophages in the lamina propria express several anti-inflammatory molecules, including IL-10 and TGF-β, which express an M2c-like phenotype. These M2c-like macrophages can induce Foxp3 + regulatory T cells in the lamina propria.
In conclusion, M2c, also known as wound-healing macrophages, are capable of repair of renal injury, and show potential as a means of treating kidney disease.
Macrophages in Unilateral Ureteral Obstruction
UUO is a well-described model that enables study of the factors that contribute to renal fibrosis. The importance of macrophages in UUO was first described by Schreiner in 1988. However, several studies demonstrate an inverse correlation between macrophage number and the degree of fibrosis , suggesting that there is a subpopulation of macrophages that is antifibrotic. Depletion of macrophages by cyclophosphamide increased fibrosis when administered late (day 14 post-UUO) but not when administered early (day 5 post-UUO). Studies in UUO have shown that BMD macrophages skewed towards an anti-inflammatory phenotype can promote fibrosis via their production of galectin-3 .
Data from UUO studies suggest that macrophages tend to be profibrotic/anti-inflammatory or antifibrotic/proinflammatory. In addition to their production of TNF-α and nitric oxide, classically activated macrophages produce matrix metalloproteinases that degrade extracellular matrix and induce TEC (and potentially myofibroblast) apoptosis, thereby reducing TEC production of TGF-β. In contrast, regulatory (IL-10/TGF-β-stimulated) macrophages generate anti-inflammatory cytokines such as TGF-β and galectin-3 which favor myofibroblast activation and extracellular matrix production . As noted previously, macrophages have the capacity to drift between different phenotypes, presumably in response to their microenvironment, and can share properties of any of the recognized phenotypes. Therefore, a challenge for future studies is to find macrophages with an anti-inflammatory antifibrotic phenotype that can be used therapeutically.
Wound-healing (M2a) Macrophages in Diabetic Nephropathy
Macrophages are an important orchestrator of the immune response in diabetic kidney disease. Tubulointerstitial inflammation is a common feature in human diabetic nephropathy, in which macrophages are major components . In addition, both macrophages and lymphocytes (CD4 + and CD8 + ) are present within the glomeruli of diabetic subjects with kidney disease.
This laboratory has investigated the effect of adoptive transfer of M2a in experimental diabetes. Studies were performed in streptozotocin (STZ)-induced type 1 diabetic wild-type C57BL/6 mice. Kidneys from diabetic mice infused with M2a had less tubular damage, glomerular hypertrophy and interstitial expansion than diabetic mice without M2a. M2a also reduced pancreatic inflammatory cell infiltration and plasma levels of glycosylated hemoglobin A1c. These findings demonstrated that macrophages that have been modified ex vivo by IL-4 and IL-13 to a regulatory phenotype may reduce the severity of both diabetes and diabetic nephropathy. Since glycemic control and islet cell damage were improved in the macrophage-treated group, these cells may reduce renal injury by improving the diabetic milieu .
This group also examined the effect of M2a in a murine model of diabetic nephropathy associated with more severe renal injury. Croker and Johnson’s laboratory recently described a model of diabetic nephropathy using the endothelial nitric oxide synthase knockout (eNOS −/−) mouse. Streptozotocin-injected eNOS −/− mice developed severe glomerulosclerosis, mesangiolysis and arteriolar hyalinosis associated with hypertension reflecting many of the renal pathological changes in type 1 diabetes. Similar changes were also observed in eNOS knockout mice cross-bred with db/db mice, a model of nephropathy in type 2 diabetes . The present group examined the therapeutic efficacy of IL-4/IL-13-stimulated macrophages (M2a) in a murine model of diabetic nephropathy. Diabetic renal injury was induced by STZ injection in eNOS −/− mice. Diabetic mice treated with M2a had significantly less arteriolar hyalinosis, glomerulosclerosis and tubular damage than control diabetic mice, while functional injury was similar. Glycemic control was similar in both groups. Adoptive transfer of M2a significantly reduced the amount of renal injury due to diabetes. These data suggest that M2a treatment protects against diabetic renal injury by inhibiting arteriolar hyalinosis and subsequent glomerular damage [Lee and Harris, unpublished and ].
Much more work needs to be done before results of these studies can be translated to the clinic. Questions such as the fate of M2a traffic in diabetic kidney disease, the length of time that M2a last in vivo, effectiveness of other M2 phenotypes such as M2c, and their potency remain unanswered. It will also be important to discover whether M2a can ameliorate renal injury in established diabetic nephropathy, a circumstance which more closely resembles that seen in the clinic.
Macrophages in Acute Kidney Injury
Acute kidney injury (AKI) is characterized by infiltration of macrophages, neutrophils, lymphocytes and natural killer (NK) cells soon after the initiation of injury. In the most common model of AKI, ischemia–reperfusion injury (IRI), interruption of blood flow to the kidney is followed within minutes by injury to renal parenchymal cells, particularly TECs. TEC injury (necrosis, as opposed to apoptosis) is followed by release of cytokines, complement factors and chemokines that recruit immune cells into the region of damage. After several weeks of IRI recovery phase, fibrosis may occur. The macrophage is the dominant cell type within the cellular infiltrate after IRI, localizes to the outer medulla of the postischemic kidney and persists well into the recovery phase . Depletion studies using clodronate have demonstrated that macrophages promote tissue injury in AKI following the initial phase and fibrosis in the recovery phase . Similarly, inhibition of macrophage recruitment in osteopontin-knockout mice inhibits fibrosis in the recovery phase of IRI , suggesting a role of macrophages in the development of fibrosis after the initial phase of injury.
However, it is possible that these depletion studies affect both proinflammatory and regulatory/anti-inflammatory macrophages. Other studies have suggested that macrophages may be involved in the prevention and repair of injury following AKI. Macrophages mediate protection against early renal injury in IRI by the production of heme oxygenase-1, an enzyme that prevents free radical formation . Vinuesa’s group investigated the effect of macrophages in the late phase of IRI. Clodronate depletion before induction of IRI was followed by adoptive transfer of RAW 264.7 cells and led to greater regeneration of cells within the kidney compared to non-transfused mice, as shown by positive staining for the proliferation markers, stathmin and proliferating cell nuclear antigen (PCNA), and increased expression of the anti-inflammatory cytokine IL-10 . Further evidence comes from Kelley’s group, who showed that colony stimulating factor-1 derived from macrophages and tubular epithelial cells promotes kidney repair in the recovery phase after IRI .
Therefore, the role of macrophages in AKI is still not clear. Current data suggest that macrophages may promote renal injury in the immediate postischemic period, but are also involved in repair in the late recovery phase. These data are consistent with the paradigm that macrophages are phenotypically diverse and play opposing roles in tissue injury and repair.
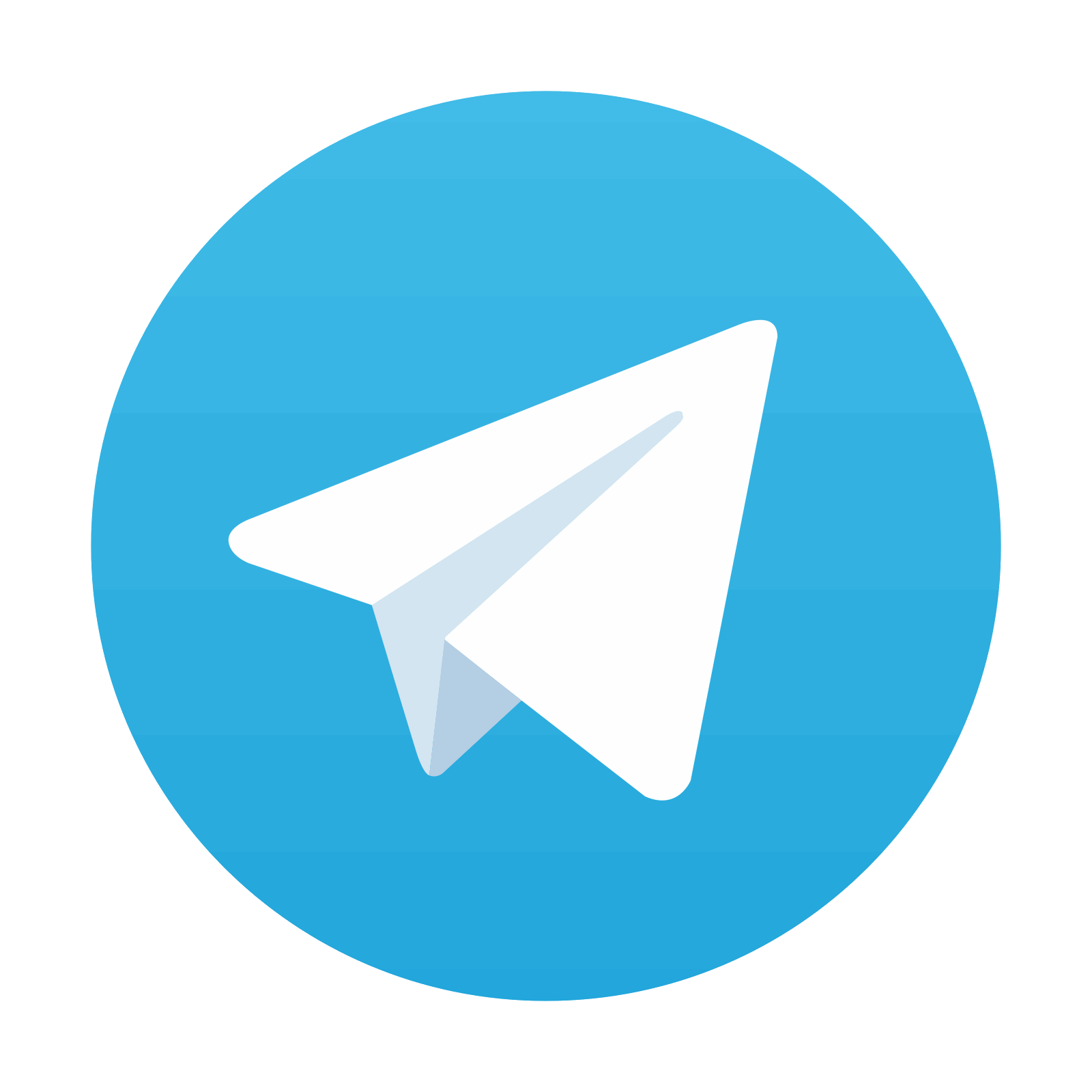
Stay updated, free articles. Join our Telegram channel

Full access? Get Clinical Tree
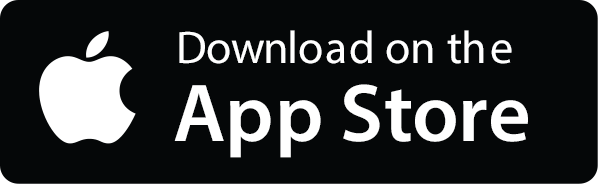
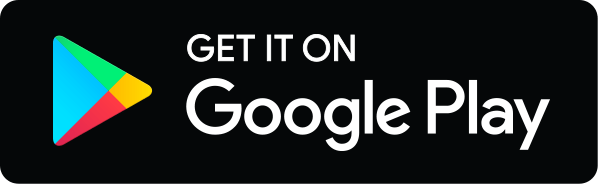