Requirements for Protein, Calories, and Fat in the Predialysis Patient
Tahsin Masud
William E. Mitch
GOALS OF NUTRITIONAL THERAPY
The goals of dietary therapy for patients with chronic kidney disease (CKD) are: (i) to diminish the accumulation of nitrogenous wastes and limit the metabolic disturbances characteristic of uremia; (ii) to prevent loss of protein stores; and (iii) to slow the progression of CKD. Protein-restricted diets improve uremic symptoms because they reduce the levels of uremic toxins, most of which result from the metabolism of protein. A low-protein diet also ameliorates specific complications of CKD, including metabolic acidosis, renal osteodystrophy, hyperkalemia, and hypertension. This occurs because a diet that is restricted in protein is invariably restricted in the quantities of sulfates, phosphates, potassium, and sodium eaten each day. Contrariwise, when the diet exceeds the daily protein requirement, the excess protein is degraded to urea and other nitrogenous wastes, and these products accumulate (Fig. 6-1). These considerations explain why dietary protein restriction has been used for decades to treat chronically uremic patients.
ASSESSING PROTEIN REQUIREMENTS
Nitrogen Balance and Protein Requirements
Nitrogen balance (Bn) is the criterion standard for assessing dietary protein requirements, because a neutral or positive Bn indicates that the body’s protein stores are being maintained or increased. Although measuring Bn is time consuming and technically demanding, no readily acceptable alternative methods are available for assessing amino acid or protein requirements.
On the basis of Bn measurements, the average protein requirement for healthy adults who perform a moderate amount of physical activity and consume sufficient (but not excessive) calories is approximately 0.6 g protein/kg body weight/day (b.w/d). This level of dietary protein was based on Bn results from subjects who had been fed variable amounts of protein. By an extrapolation process, the World Health Organization determined that the average protein intake required to maintain neutral Bn was 0.6 g protein/kg b.w./day, and this value plus 2 standard deviations (approximately 0.75 g protein/kg b.w./day) was designated as the “safe level of intake.” This amount meets the protein requirements of 97.5% or more for healthy adults. Dietary protein above these levels is converted to waste products that must be excreted by the kidney.
MECHANISMS RESPONSIBLE FOR NEUTRAL NITROGEN BALANCE
Healthy Subjects
For adults in Western societies, the protein intake usually exceeds the requirement. But consuming more protein does not increase muscle mass; instead, the body adapts by oxidizing any excess of amino acids from dietary protein. The nitrogen liberated by amino acid oxidation is converted to waste nitrogen (principally urea) and excreted by the kidney. When the intake of protein decreases, amino acid oxidation is reduced, allowing amino acids to be recycled and yielding more efficient use of dietary essential amino acids (EAA) (see Chapter 1).
Besides amino acid oxidation, protein synthesis and degradation determine the level of Bn. Note that the daily rates of protein synthesis and degradation are much larger than the rate of amino acid oxidation (Fig. 6-2); approximately 280 g of body protein are synthesized and degraded in a 70-kg adult each day. With fasting, however, body protein stores (principally skeletal muscle) are degraded to amino acids, which are used in the liver for gluconeogenesis. With feeding, protein degradation declines sharply, and protein synthesis repletes body protein stores. The net daily result is a neutral (or positive) Bn, and protein stores will be preserved as long as the diet is nutritionally adequate. A principal modulator of these changes in protein turnover is insulin, the principal anabolic hormone. In adult subjects, insulin mainly suppresses protein degradation. In summary, healthy adults successfully adapt to dietary protein restriction by: (i) suppressing catabolism
of EAA, and (ii) inhibiting protein degradation and increasing protein synthesis with feeding.
of EAA, and (ii) inhibiting protein degradation and increasing protein synthesis with feeding.
Patients with Chronic Kidney Disease
Patients with advanced but uncomplicated CKD (glomerular filtration rate [GFR] approximately 5 to 15 mL per minute depending on the etiology of CKD) are remarkably efficient in adapting to dietary protein restriction. These patients reduce rates of amino acid oxidation and protein degradation in the same fashion as normal adults when their protein diet is restricted from 1.0 to 0.6 g/kg/day. The same adaptive metabolic responses are activated when the diet is restricted to only 0.3 g/kg/day and a supplement of essential amino acids or their nitrogen-free analogues (ketoacids) is given. Such a dietary regimen maintains both neutral Bn and indices of adequate nutrition during more than 1 year of observation. This happens because patients with CKD activate the same adaptive responses to dietary protein restriction as healthy adults. First, amino acid oxidation is decreased, resulting in both a lower requirement for and more efficient utilization of essential amino acids. Second, if protein or amino acid intake is barely sufficient, neutral Bn is achieved by a postprandial suppression of whole body protein degradation, with or without an accompanying increase in protein synthesis. But when protein (or amino acid) intake is inadequate, these compensatory response(s) are incomplete, leading to negative Bn and loss of lean body mass.
Dietary Protein Restriction and Nephrotic Syndrome
Patients with nephrotic syndrome (i.e., more than 3 g proteinuria per day) could have an increased risk for protein wasting. This
issue is very important, because patients who are given a well-designed low-protein diet (LPD) have decreased proteinuria and increased serum albumin concentrations compared with patients fed excessive amounts of protein. Because the degree of proteinuria is closely related to the risk for progressive kidney and cardiovascular diseases, LPD could help prevent these problems. Fortunately, restricting dietary protein also activates adaptive responses in patients with nephrotic syndrome as determined by positive results in measurements of Bn and the components of protein turnover when they consumed a diet containing 0.8 or 1.6 g/kg b.w./day protein (plus 1 g/kg b.w./day dietary protein for each g proteinuria) and 35 kcal/kg b.w./day energy. In nephrotic and age- and gender-matched adults, a low-protein diet suppressed amino acid oxidation, and protein degradation decreased while protein synthesis increased during feeding. These responses resulted in neutral or positive Bn. The increase in amino acid oxidation induced by feeding was also blunted, suggesting that the urinary protein losses were closely associated with suppression of amino acid oxidation. Thus, proteinuria in CKD does not block the adaptive responses that are required to improve or maintain protein homeostasis. Evidence also suggests that diets containing less protein (<0.6 g/kg b.w./day) do not increase the risk for protein wasting in patients with the nephrotic syndrome.
issue is very important, because patients who are given a well-designed low-protein diet (LPD) have decreased proteinuria and increased serum albumin concentrations compared with patients fed excessive amounts of protein. Because the degree of proteinuria is closely related to the risk for progressive kidney and cardiovascular diseases, LPD could help prevent these problems. Fortunately, restricting dietary protein also activates adaptive responses in patients with nephrotic syndrome as determined by positive results in measurements of Bn and the components of protein turnover when they consumed a diet containing 0.8 or 1.6 g/kg b.w./day protein (plus 1 g/kg b.w./day dietary protein for each g proteinuria) and 35 kcal/kg b.w./day energy. In nephrotic and age- and gender-matched adults, a low-protein diet suppressed amino acid oxidation, and protein degradation decreased while protein synthesis increased during feeding. These responses resulted in neutral or positive Bn. The increase in amino acid oxidation induced by feeding was also blunted, suggesting that the urinary protein losses were closely associated with suppression of amino acid oxidation. Thus, proteinuria in CKD does not block the adaptive responses that are required to improve or maintain protein homeostasis. Evidence also suggests that diets containing less protein (<0.6 g/kg b.w./day) do not increase the risk for protein wasting in patients with the nephrotic syndrome.
In summary, patients with uncomplicated CKD, including those with nephrotic range proteinuria, activate normal compensatory responses to dietary protein restrictions by suppressing EAA oxidation and reducing protein degradation. Consequently, Bn is neutral and lean body mass is maintained during long-term dietary therapy.
Complications that Impair Adaptive Responses in Protein Turnover
Metabolic acidosis and insufficient insulin are complications of CKD, and both limit adaptive nutritional responses to an LPD. In muscle, acidosis and acute insulin deprivation stimulate the oxidation of EAA and accelerate the degradation of protein. Metabolic acidosis, in addition to causing negative Bn in adults and impairment of growth in children and infants, also causes insulin resistance in healthy adults. Fortunately, the risks for metabolic acidosis and insulin resistance are substantially reduced with an LPD.
The branched-chain amino acids, valine, leucine, and isoleucine, are catabolized by branched-chain keto acid dehydrogenase (BCKAD), and acidosis increases the activity of this enzyme in skeletal muscle. The activation includes an increase in the muscle levels of mRNAs that encode BCKAD enzyme complex, suggesting there is an increased capacity to degrade these amino acids. Acidosis also accelerates protein degradation by increasing the activity of the adenosine triphosphate (ATP)-dependent, ubiquitin-proteasome proteolytic pathway. As with BCKAD, the mRNAs that encode components of the pathway are increased. These cellular responses explain why metabolic acidosis counteracts the adaptive responses activated by a LPD.
Acid is generated during metabolism of animal-derived proteins, and there is a strong inverse relationship between a higher protein intake and reduced predialysis serum bicarbonate concentration. Similarly, a significant increase in serum bicarbonate level occurred in the Modification of Diet in Renal Disease (MDRD) study in patients with both stage 3 and 4 CKD when protein intake decreased by 0.2 g/kg b.w/day. As a general rule, the serum HCO3 should be kept at 24 mmol/L or more to prevent the loss of muscle mass and to treat the bone, hormonal, and other complications of acidosis. Usually, two or three 650-mg sodium bicarbonate tablets (approximately 8 mEq of sodium and bicarbonate per tablet), given two to three times daily, are well tolerated and effective.
Amelioration of Insulin Resistance
Subjects with advanced CKD develop glucose intolerance, with fasting hyperglycemia and hyperinsulinemia, especially when metabolic acidosis complicates CKD. The major metabolic abnormality resides in peripheral tissues, including decreased glucose uptake by skeletal muscle and adipose tissue, both without and with insulin. In contrast, hepatic glucose production is normal and is appropriately suppressed in response to insulin. The abnormality involves defects after insulin interacts with its receptor (i.e., a postreceptor defect) apparently caused by suppression of phosphatidyl-inositol 3 kinase activity. Interestingly, diabetic patients with CKD treated with a low-protein diet displayed improvements in insulin sensitivity, fasting serum insulin levels, their need for daily insulin support, lower blood glucose levels, and a lower rate of endogenous glucose production.
OTHER ADVANTAGES OF LOW PROTEIN INTAKE
Amelioration of Hyperfiltration and Proteinuria
Both in experimental models and humans, high protein load acutely increases GFR, potentially contributing to the severity of microalbuminuria and, over the long term, glomerulosclerosis. A linear relationship between reduction in protein intake and decrease in proteinuria was first demonstrated in animals with CKD and was confirmed in several clinical trials. Levels of profibrotic compounds, such as transforming growth factor α (TGFα) and plasminogen activator inhibitor, can be reduced by an LPD, resulting in less severe renal injury and proteinuria compared with results with an uncontrolled diet.
Improvement of Lipid Profile
LPDs prescribed for patients with CKD are commonly designed by limiting animal sources of protein (e.g., meat and dairy products). As such, the intake of saturated lipids associated with animal proteins is decreased, resulting in an improved serum lipid profile. Reducing protein intake from 1.1 to 0.7 g/kg b.w/day for 3 months led to increased levels of serum lipoprotein A-1 and the apolipoprotein A-1:apoprotein B ratio.
Effect on Mineral and Bone Disorder
Phosphorus is an integral constituent of animal-derived proteins (1 g of protein contains about 13 mg of phosphorus), so reducing
protein intake automatically reduces phosphate intake. Reduced phosphate accumulation will lower serum levels of parathyroid hormone and improve renal osteodystrophy. In fact, renal osteomalacia and osteofibrosis do improve after 12 months of an LPD supplemented with ketoanalogs. VLPD was also associated with a decrease in the urinary excretion and serum levels of urea nitrogen and phosphate.
protein intake automatically reduces phosphate intake. Reduced phosphate accumulation will lower serum levels of parathyroid hormone and improve renal osteodystrophy. In fact, renal osteomalacia and osteofibrosis do improve after 12 months of an LPD supplemented with ketoanalogs. VLPD was also associated with a decrease in the urinary excretion and serum levels of urea nitrogen and phosphate.
Improvement in Blood Pressure
Another unexpected advantage of low protein intake is the improvement in blood pressure (BP); blood pressure decreased markedly (in the order of 15 mm Hg in systolic BP and 6 mm Hg in diastolic BP) in patients with CKD treated with a VLPD supplemented with ketoanalogs. Decreased protein intake of about 30% was associated with a 28% drop in sodium intake, but there was no change in BP or sodium intake in the two groups of patients whose protein intakes were not modified.
Amelioration of Erythropoietin Unresponsiveness
The relationship between the uremic state and erythropoiesis in patients with advance CKD (creatinine clearance ≤25 ml/mim/1.73m2) was evaluated after randomization to either LPD (0.6 g/kg b.w/day) or VLPD (0.3 g/kg b.w/day) supplemented with ketoanalogs. The erythropoietin (EPO) dose to maintain hemoglobin was unchanged in patients treated with a LPD but progressively decreased in those treated with the VLPD.
CONCERNS ABOUT LOW-PROTEIN INTAKE DURING THE COURSE OF PROGRESSIVE CHRONIC KIDNEY DISEASE
Two observations have raised concerns about using LPDs. First, an association was observed in patients receiving hemodialysis therapy between hypoalbuminemia and increased mortality; second, patients with CKD starting dialysis with advanced CKD had worse outcome. Let’s examine both of these issues in detail. The finding that dialysis patients often have low levels of serum proteins has led some to suggest that low-protein diets should be used cautiously or avoided in patients with CKD and that an “early start” of dialysis therapy should be considered. If patients with CKD are not properly instructed and supervised, a spontaneous decrease in protein intake and deterioration of some nutritional indices may indeed occur. Conversely, hypoalbuminemia in these patients is linked more closely to other factors such as inflammation and fluid status than to dietary inadequacy alone. In fact, patients with CKD who are not on maintenance dialysis treated with LPDs are shown to have increased serum protein concentrations at the initiation of maintenance dialysis therapy. An LPD is also associated with improved survival of patients with CKD who subsequently began maintenance dialysis. Further, there is abundant evidence that with proper implementation, an LPD yields neutral nitrogen balance and the maintenance of normal serum proteins and anthropometric indices during long-term therapy. After beginning dialysis, patients treated with supplemented very low-protein diet (SVLPD) were found to rapidly increase their protein intake with a gain in lean body mass in a study that followed patients with CKD treated with SVLPD for
5 years after beginning renal replacement therapy; the mortality was low and did not correlate with nutritional parameters measured at the end of SVLPD therapy. Finally, the conclusion that there is a negative impact of low residual renal function on survival are flawed by a failure to take into account “lead-time bias.” This bias refers to the influence of measuring survival from the start of dialysis. This seems to increase the apparent survival of those who started with more residual renal function (i.e., earlier in the course of the disease) compared with those who start dialysis with less residual renal function. When lead-time bias was considered in patients with CKD followed from an estimated creatinine clearance (eCcr) of 20 mL/minute to one of 8.3 mL/minute after being divided into early and late start groups, there was no survival benefit of survival from an earlier initiation of dialysis. More recently, a study examining data from the dialysis Morbidity Mortality Study Wave II to evaluate if beginning dialysis at higher levels of creatinine clearance or GFR (estimated from the MDRD formula) concluded that there is insufficient evidence to advocate early initiation of dialysis.
5 years after beginning renal replacement therapy; the mortality was low and did not correlate with nutritional parameters measured at the end of SVLPD therapy. Finally, the conclusion that there is a negative impact of low residual renal function on survival are flawed by a failure to take into account “lead-time bias.” This bias refers to the influence of measuring survival from the start of dialysis. This seems to increase the apparent survival of those who started with more residual renal function (i.e., earlier in the course of the disease) compared with those who start dialysis with less residual renal function. When lead-time bias was considered in patients with CKD followed from an estimated creatinine clearance (eCcr) of 20 mL/minute to one of 8.3 mL/minute after being divided into early and late start groups, there was no survival benefit of survival from an earlier initiation of dialysis. More recently, a study examining data from the dialysis Morbidity Mortality Study Wave II to evaluate if beginning dialysis at higher levels of creatinine clearance or GFR (estimated from the MDRD formula) concluded that there is insufficient evidence to advocate early initiation of dialysis.
The greatest challenge for physicians and dieticians is compliance of patients with LPD. In MDRD study, the compliance rate for patients assigned to 0.6 g/kg b.w/day diet, did not exceed 35% in study A and 46% in study B. Clearly, a skilled dietician must be involved in the treatment of patients with CKD. The nutritionist must be familiar with the problems specific to patients with CKD and must have knowledge about the use of seasonings that can make meals appetizing and pleasant.
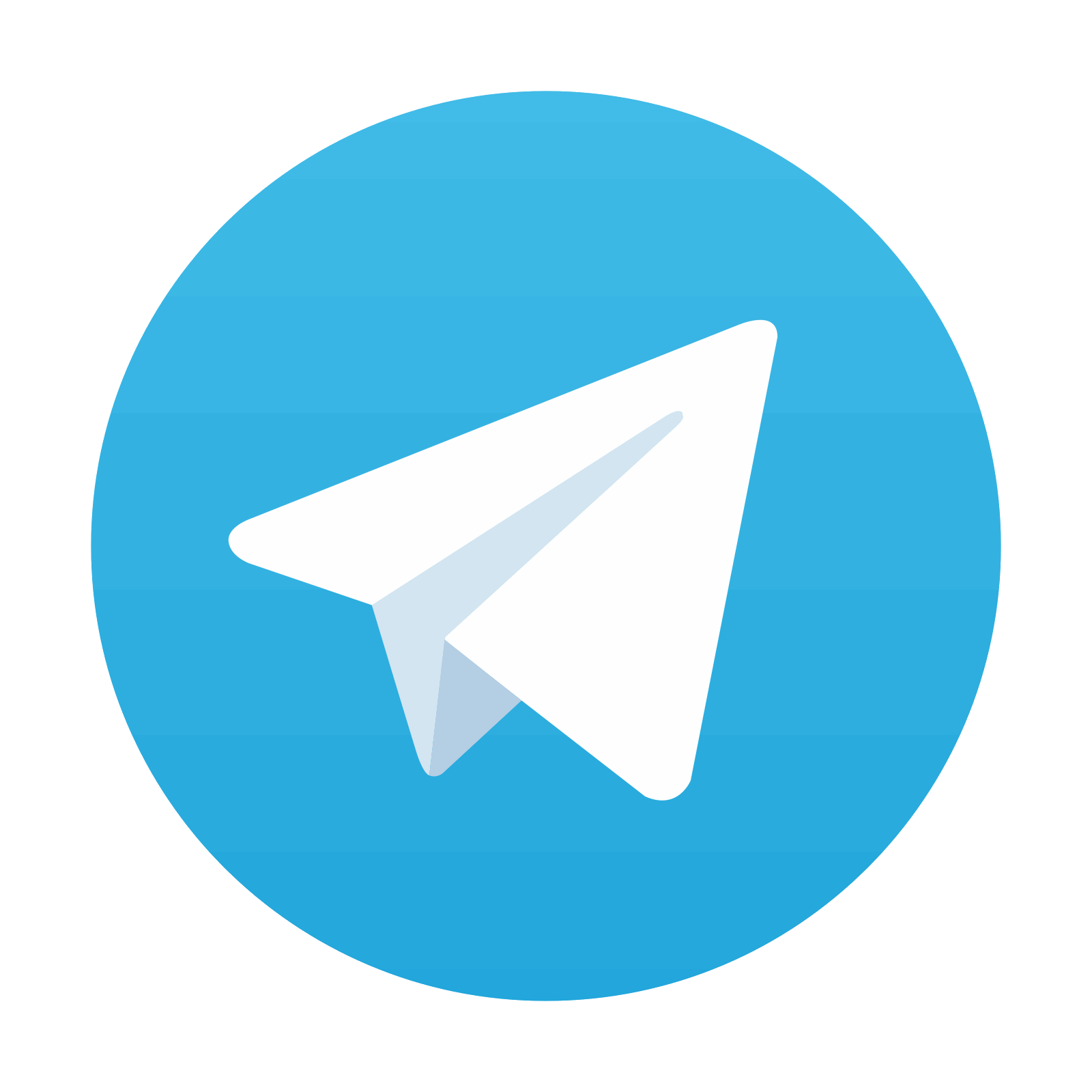
Stay updated, free articles. Join our Telegram channel

Full access? Get Clinical Tree
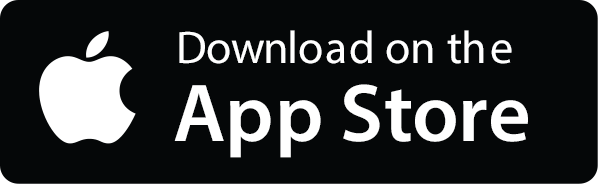
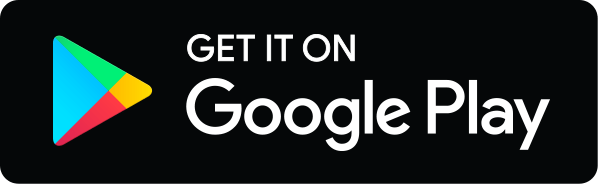