8 Oliver W. Hakenberg Department of Urology, University Hospital Rostock, Rostock, Germany Allogenic renal transplantation is the best treatment for many patients with chronic renal failure because transplantation restores all functions of the kidney. Health‐related quality of life (QoL) markedly improves after renal transplantation and the side effects of dialysis treatment as well as the progression of renal failure–related organ and tissue deterioration, which are not treated effectively by dialysis, are much reduced. To achieve good results of renal transplantation, however, good preoperative and peri‐ and postoperative conditions have to be established. Patients on waiting lists need to be well prepared. Interdisciplinary patient care before and after renal transplantation is needed to achieve durable and long‐term success. Keywords: kidney transplantation; allogenic; immunosuppression; graft function; graft survival Renal transplantation and chronic dialysis are the two treatment options for chronic renal failure (end‐stage renal disease [ESRD]). ESRD is defined as the need for chronic dialysis, which is the filtration of water and metabolic waste products. Other renal functions (i.e. regulation of blood pressure, of Ca2+/phosphate homeostasis and bone metabolism, and of red blood cell production), which maintain physiological homeostasis, cannot be corrected by dialysis. Over the years, patients on dialysis develop secondary hypertension, peripheral vascular disease, hyperparathyroidism, anaemia, infertility, and erectile dysfunction and have a much reduced life‐expectancy. Renal transplantation can restore all the deficient functions of a normal kidney and is a significantly better treatment modality for ESRD than chronic dialysis. The life expectancy of transplant recipients is much higher than that of patients on dialysis and approaches that of healthy individuals, depending on the number of years spent on dialysis treatment. Conditions following from ESRD will be cured or improved (e.g. hypertension). Existing pathology such as atherosclerotic disease cannot be reverted; however, further progression can be halted or slowed down. In children, normal growth is restored. Today, average five‐year graft survival after renal transplantation is about 70% and average patient five‐year survival is higher than 80%. The results and outcomes of renal transplantation differ amongst countries [1]. Most patients who undergo renal transplantation have ESRD as a result of chronic interstitial nephritis, diabetes, nephrotic syndromes, hypertension, and polycystic disease. In many cases, the exact cause is renal failure unknown. Theoretically, most patients with ESRD are suitable for renal transplantation. In practice, this is not the case. Patients with long‐standing ESRD and complicating factors such as obesity, hypertension, peripheral vascular disease or cardiac dysfunction, and advanced age are often poor candidates for transplantation, although none of these conditions on its own is a contraindication. Only few causes of ESRD are absolute contraindications because these have a high chance of recurrence and will destroy the graft (Table 8.1). Table 8.1 Approximate risk of recurrence of renal disease in the transplanted kidney. Organs from brain‐dead donors are the main source for renal transplantation. Although it would be desirable to have all patients with ESRD undergo transplantation, this is not possible because of the shortage of donor organs. There are a number of ethical and regulatory guidelines and legislations surrounding organ donations. These are highly dependent on cultural and historical attitudes of each country. These vary from organ retrieval from patients who are brain dead without explicit consent to the need for formal written declaration of either the deceased or the next of kin. The number of organ donations varies amongst different countries depending on attitudes and legislation [2] (Table 8.2). Table 8.2 Organ donation rates in different countries (per million inhabitants/per year in 2012 [2]). As the need for donor kidneys is much greater than actual donations, measures aimed at increasing the organ pool have been implemented, such as less stringent criteria regarding donor age and comorbidities. This has led to the use of kidneys from much older donors and of so‐called ‘marginal organs’. Experience has shown that reasonable results can still be obtained. An alternative to the use of cadaver donor organs is life donation. Again, legislation differs amongst countries. Some allow it for unrelated and even unconnected persons (e.g. the US and the UK), whereas others restrict it to relatives and closely connected persons. Commercial life donation is illegal in European countries but allowed in some other countries (e.g. Iran). Some Asian countries have programs of using organs from donors who undergo the death penalty (e.g. Singapore and China). ‘Cross‐over’ live donor transplantation between couples with an incompatible ABO constellation is legal in only some countries (i.e. Netherlands, UK, US). The US and the UK also allow for so‐called ‘altruistic kidney donation’, which is a completely unrelated and anonymous live kidney donations [3]. The procurement, allocation, and transplantation of human organs is regulated by law and special authorities oversee this process. In the UK, this is the Human Tissue Authority (HTA). Organ allocation can be on a purely national basis (e.g. UK, Norway, Switzerland, and France), whereas other European countries (Austria, Belgium, Croatia, Germany, Hungary, Luxembourg, Netherlands, and Slovenia) have formed a multinational institution, Eurotransplant, to increase the donor pool and improve matching. Allocation is based on age, waiting time, blood group, human leucocyte antigen (HLA) matching, and in some countries (e.g. the UK), on body size of donor and recipient. Children are given priority. The waiting time varies between different programs. In the UK, on average it is three years and in Germany five. Patients with rare blood groups and rare HLA‐constellations have a longer wait time. Putting a patient on a waiting list for kidney transplantation requires the diagnosis of ESRD. The day on which chronic dialysis begins is counted as the beginning of the wait time. ‘Preemptive renal tranplantation’ before a patient has actually become dependent on dialysis is only possible with a live donation. Contraindications against renal transplantation are conditions which endanger the success of the surgical procedure, risk severe complications of immunosuppression, or put doubt on the necessary compliance required for successful immunosuppressive treatment [3, 4]. A history of malignancy requires several years of recurrence‐free survival before immunosuppression can be considered safe (Table 8.3). Table 8.3 Recommended minimum recurrence‐free survival (years) after curative treatment for cancer before renal transplantation should be considered. Investigations need to assess cardiovascular fitness for anaesthesia and surgery, to exclude common malignancies, to examine the state of the large pelvic vessels and the degree of renal anaemia, hypertension, and secondary hyperparathyroidism. Chronic or latent infections of the urinary tract should be treated. Bladder capacity and residual diuresis have to be assessed. In severe bladder disorders, preparations for urinary diversion with renal transplantation must be considered. Pretransplant nephrectomy is indicated for nonfunctioning kidneys that are a source of repeated infections, bleeding, or the cause of medically refractory hypertension. In patients with polycystic kidneys, it is usually not necessary to remove a kidney to make room for a transplant. It is better to leave both native kidneys unless they cause problems because the residual water diuresis increases the quality of life (QoL) under dialysis considerably. All potential recipients require blood group and HLA typing as well as the assessment of the presence of preformed antibodies. For a successful transplantation, the risk of rejection needs to be minimised. ABO blood group compatibility and as much HLA compatibility as possible is aimed for. Preformed antibodies may exist as a result of previous exposure to HLA‐antigens by blood transfusion, pregnancies, or may be have been induced by autoimmune diseases. A high level of preformed antibodies does not preclude successful transplantation, but immunosuppression needs to be more intensive. Potential donors who are brain have to be screened for infections and cancer, so that these are not transmitted to the immunocompromised transplant recipient. Contraindications for organ donation in general are listed in Table 8.4. Table 8.4 Contraindications for organ donation. CMV, cytomegalovirus; EBV, Epstein‐Barr virus; HIV, human immunodeficiency virus; TB, tuberculosis. Brain death implies that there is irreversible loss of brain stem function, and this is based on neurological examination, electroencephalogram (EEG), or cerebral angiography. Usually, these investigations have to be repeated to document irreversibility. Diagnostic criteria for brain death is strict and should be made by physicians not involved with organ procurement and transplantation (Table 8.5). It is the duty of the explanting surgeon to make sure that the appropriate criteria for the diagnosis of brain death have been used. Table 8.5 Diagnostic criteria for brain death. Concern for the feelings of bereaved relatives as well as the need to release scarce intensive care resources require that surgery for organ retrieval should be performed soon [5]. If consent from the next of kin is required, a trained transplant coordinator is likely to have the necessary skills to present organ donation as an opportunity for good amidst tragedy. Organs from living donors could potentially make up for the shortage of cadaveric kidneys. There are several advantages of live‐donor renal transplantations: the donor and recipient operations can be planned and performed almost simultaneously so that ischaemia time is minimised. Secondly, persons selected for live kidney donation are healthy, and therefore, live donor kidneys are perfect kidneys in contrast to cadaver kidneys, which are often of comparatively poor quality. In addition to the contraindications that apply to all donor kidneys (Table 8.4), the potential life donor must not be under emotional, social, or even financial pressures for the donation. The surgery for kidney retrieval has some morbidity, and a small but undeniable risk of mortality. There can be untoward psychological sequelae regardless of whether or not the transplantation is successful. Overall life expectancy is not impaired after kidney donation, but there seems to be a slightly increased risk of developing ESRD. Potential donors, therefore, have to be adequately counselled and need to understand all aspects of the sacrifice they are making. The donor’s medical work‐up has to include split renal function studies, an intravenous urogram or computed tomography (CT), and renal arteriograms as well as a investigations aimed at identifying contraindications. The donor who is brain dead needs to be conditioned for safe procurement of organs in good condition. Blood volume has to be increased by intravenous fluids and diuresis and cardiovascular stability need to be maintained (e.g. mannitol, diuretics). Anaesthesia is not required for organ removal in donors who are brain dead. However, occasionally there are spinal reflex movements which can be disturbing to the staff and are not helpful for promoting organ donation. It is better to avoid this altogether by relaxation. Donor kidneys need to be removed with long renal vessels, intact hilar fat, and the fine vessels that supply the ureter. In a heart‐beating donor, there is usually no need for haste, unless there is circulatory instability. A long midline incision is made from the xiphoid process to the symphysis, for multiorgan removal, the thoracic cavity needs to be opened as well. All organs should be inspected for malignancy or infection. The ascending colon and small bowel are mobilised by incising their peritoneal reflections and placed on the chest (Figure 8.1). The superior mesenteric and coeliac arteries are divided for access to the aorta well above the renal vessels (Figure 8.2). Aorta and vena cava are taped just above the bifurcations, the aorta is clamped at the level of the coeliac artery, and a tube is inserted (Figure 8.3). Cooled preservation solution (4 °C) is run in, usually 4 l, until the effluent from the vena cava is quite clear. The perfusion cools the organs and reduces their metabolism as well as oxygen demand. Secondly, the organs need to be flushed free of all blood which would otherwise clot in all small vessels. Figure 8.1 The bowel is mobilised completely and placed on the chest. Figure 8.2 (a and b) The superior mesenteric and (c) coeliac arteries are divided between ligatures. Figure 8.3 Perfusion of the kidneys through a Foley catheter in the aorta. The aorta and vena cava can be removed en bloc with the kidneys, or they can be split open in the midline and divided posteriorly before removal (Figure 8.4). The latter approach allows for close inspection of additional renal vessels because it is of utmost importance to secure all renal arteries and veins if there are several. The upper two‐thirds of the ureters are dissected, preserving their blood supply (Figure 8.5). The kidneys with their patch of aorta and vena cava are then removed and placed in a bowl of cooled perfusion fluid. A slice of splenic tissue is removed for tissue typing. The kidneys are then placed in sterile bags with perfusion fluid and packed into an ice‐cooled container for transportation. Figure 8.4 The aorta and vena cava are removed en bloc with the kidneys and upper two‐thirds of the ureters. Figure 8.5 (a and b) The aorta and vena cava are slit open in the midline, carefully noting if more than one renal artery is present on either side. As the feelings of relatives who may wish to see the deceased donor later need to be respected, wound closure should be diligent, including wound dressing, and furthermore, the body should be clean at the end of surgery. Every living donor undergoes extensive and careful investigations to make sure that the remaining kidney is perfect, and that there is no unsuspected serious disorder that would put the donor at risk. There are few procedures as stressful for a surgeon as removing a good kidney from a healthy donor and transplanting it to another individual. The expectations of all involved that nothing whatsoever can go wrong are extremely high. It should be explained to the donor that the operation is safe and that there is a normal life expectancy with only one kidney. Mortality as a result of live kidney donation is low (about 0.05%, most of them from pulmonary embolism) [6]. However, there are data suggesting that live donors have a slightly increased risk of later developing ESRD themselves [7]. The choice of which kidney to use from a living donor depends on split renal function and angiography. If one kidney is better than the other, the better kidney should be left to the donor. If there are multiple vessels on one side, it is preferable to use the kidney with single vessels because this makes the transplant surgery easier and safer. If there are no differences between the left and the right side, it is preferable to take the left kidney because of its longer renal vein. There are different ways of performing live donor nephrectomy: open, laparoscopic, or hand‐assisted laparoscopic [8]. Whichever method is used, the main features are that dissection is meticulous, and the kidney removed with long vessel stumps and without any injuries. With open surgery, the incision should be as short as possible. Adequate exposure can be provided by a 12th rib bed approach but also by a subcostal incision, which tends to cause less postoperative pain. The renal vessels are dissected and taped (Figure 8.6). On the left side, care should be taken for the adrenal vein and a large lumbar vein usually entering the left renal vein posteriorly. The hilar fat should be left intact, and injury of the small ureteral vessel must be avoided. Then, the ureter is dissected distally and cut. Finally, first the renal artery is clamped, then the renal vein, and then both are cut. The kidney is placed in cooled preservation solution and organ perfusion is started (Figure 8.7). Figure 8.6 Living donor nephrectomy. On the left side beware of a circumaortic renal vein, or a large lumbar vein entering the renal vein at this point. Figure 8.7 The donor kidney is irrigated with ice‐cold preserving fluid until the fluid emerging from the renal vein is seen to be clear. It is advisable to have a scrubbed assistant ready to commence organ perfusion immediately because the warm ischemia time is crucial. Only then are the stumps of the renal vessels ligated. Great care must be taken for vascular control as donor complications must be avoided. The scar should be cosmetically acceptable. Perioperative antibiotics for the donor are advisable. Warm ischemia time consists of the time between clamping of the renal vessels and starting cold organ perfusion plus the time later needed for suturing the vascular anastomoses when the organ is already in the recipient’s body. Total warm ischemia time should be as short as possible. Whether both donor and recipient are operated on at the same time in two theatres or one after the other is not of importance. For graft survival, organ conservation by perfusion and storage in a cooled solution is vital. The composition of the perfusate is aimed at reducing cellular oedema and potassium loss and minimising cellular oxygen demand and metabolic activity. There are three different commonly used solutions (Euro‐Collins, University of Wisconsin, and histidine‐tryptophan‐ketoglutarate [HTK]). These solutions differ in composition (Table 8.6) [9, 10]. The HTK solution is widely used in Europe, and the Wisconsin University solution includes a synthetic colloid (hydroxyethyl starch) introduced for livers and is not widely used for kidneys. Using these fluids and keeping the kidney in a sterile ice‐cold container can preserve them for many hours. Table 8.6 Possible causes and differential diagnosis of delayed graft function. The success of kidney transplantation is highly dependent on ischemic time. Although kidneys can be transplanted more than 24 hours after removal from the donor, positive results decrease with every hour. The surgical technique of renal transplantation is the same for kidneys from a cadaver or a living donor. The kidney is usually transplanted extraperitoneally into the contralateral iliac fossa. An oblique groin incision or a pararectal incision can be used. The inferior epigastric vessels can be preserved. The peritoneum is reflected medially, and the common iliac vessels and the bladder are exposed (Figure 8.8). Figure 8.8 Extraperitoneal exposure of the common and internal iliac vessels. Usually, an end‐to‐side anastomosis of the renal vessels to the external iliac vein and artery is performed (Figure 8.9). In case of recipient atherosclerosis, a patch with as little atheroma as possible should be selected. Sometimes the anastomosis has to be done on the common iliac or end‐to‐end onto the internal iliac artery (Figures 8.10 and 8.11). This latter option has the disadvantage of disrupting distal pelvic blood supply in patients who often already have peripheral vascular disease. Care should be taken to ligate the lymphatics in the connective tissue sheath of the vessels to prevent the subsequent formation of a lymphocele. Figure 8.9 (a) Preparation of the external iliac artery for end to side anastomosis of the donor renal artery to the external iliac artery. (b) External iliac vein prepared for anastomosis. Figure 8.10 Preparation of the internal iliac artery. Figure 8.11 End‐to‐end anastomosis of the internal iliac artery to the donor renal artery. If there are problems of space or extensive scarring because of a prior surgery, the renal vessels can also be anastomosed directly to the lower aorta and vena cava as is commonly done in paediatric transplantation. The venous anastomosis should be done first and should be wide to prevent outflow obstruction. A 4–0 or 5–0 monofilament suture (e.g. Prolene) is used after clamping the iliac vein with a Satinsky clamp, opening it with a scalpel and flushing it with a heparinized solution of physiological saline. Similarly, the arterial anastomosis is done with a 5–0 or 6–0 suture after spatulating the renal artery. The vessels are flushed with heparinized saline to expel the air before the sutures are tied. When there is more than one renal artery, it is preferable to have them on one aortic patch for a single anastomosis as this reduces anastomotic time, which is crucial because it represents warm ischemia (Figure 8.12). However, this is not always possible in which case it is necessary to anastomose each renal artery separately. If the recipient artery is found to be very atheromatous, endarterectomy can be done or a prosthetic vascular replacement may need to be inserted. Figure 8.12 Where there is more than one renal artery, both should be left on one aortic patch, which is sewn onto the side of the external iliac artery. When the vascular anastomoses have been done, bulldog clamps are put on the renal vessels before the clamps are taken off the iliac vessels. Only when both anastomoses are satisfactory are the bulldog clamps removed; the venous before the arterial clamp and graft reperfusion begun (Figure 8.13). The pulse of the renal artery should be clearly palpable and the filling of the renal vein visible. With good perfusion, the colour of the transplant turns from white to a healthy pink quickly. Figure 8.13 The vascular anastomoses are completed, and the submucosal tunnel is then prepared in the bladder for the ureter. For the ureterovesical anastomosis, standard techniques such that of Lich‐Gregoire can be used can be used. Because postoperative ureteral problems are more often those of stenosis rather than reflux, it is preferable to do a simple anastomosis with only a short submucosal tunnel on the anterior part of the bladder (Figure 8.14). The ureter should be spatulated and a short double‐J stent inserted. Figure 8.14 (a and b) A wide elliptical anastomosis is made between the ureter and bladder mucosa. (c) The terminal 5 cm of ureter are buried in a loose tunnel of bladder muscle. Graft function depends on good perfusion; therefore, a duplex ultrasound within the first 24 hours is done. If there are problems either with inadequate arterial perfusion or obstructed venous outflow, an early reoperation to correct the problem may be necessary. Venous obstruction often leads to renal vein thrombosis and graft loss. Problems with arterial perfusion can be the result of intimal lesions, dislodged atherosclerotic plaques, or more commonly, kinking of the renal artery. Kinking requires surgical revision and placing the kidney medially without re‐anastomosis. Anastomotic arterial stenosis can be managed by interventional dilatation [11]. Secondary bleeding with hematoma or urinoma or a lymphocele formation may occur. More often than in nontransplant surgery, these require revision surgery because the risk of infection is greater, but conservative treatment is preferable. Lymphoceles can compress vessels or the ureter. Ureteral anastomotic stenosis needs stenting, dilatation, or reimplantation. Spontaneous rupture of the graft is a life‐threatening complication leading to massive haemorrhage but has become rare with modern immunosuppression. Acute rejection can occur precipitated by failure to take the medication, infection or a blood transfusion. Early anuria of the transplanted kidney is a common complication in cadaver kidneys and is usually due to acute tubular necrosis (ATN) resulting from severe ischemic damage [5]. It is, however, necessary to exclude all other correctable causes (Table 8.7). Table 8.7 Common maintenance immunosuppressive combination regimens. CyA, cyclosporine; MMF, mycophenolate mofetil. The patient may be dehydrated. After years on dialysis, many patients find it difficult to change from a restricted fluid intake to copious intake. Intravenous infusions may need to be given. There may be complications causing urinary obstruction. The simplest problem is a misplaced or obstructed bladder catheter. There may be ureteral anastomotic stenosis or insufficiency. Ultrasound will show a full or an empty bladder, hydronephrosis of the transplant kidney, or a perivesical fluid collection (urinoma). Transplant hydronephrosis and urinoma will need a double‐J catheter or a percutaneous nephrostomy before revision surgery. Doppler ultrasound will show impaired graft perfusion by arterial stenosis or venous thrombosis. For arterial stenosis, interventional angiography may be required. Venous thrombosis leads to graft loss. Doppler sonography will also show the resistance index which if increased (> 0.8) indicates rejection [12]. Blood levels of nephrotoxic immunosuppressants may be too high, requiring dose adjustments. If these causes have been excluded, a biopsy will show either rejection or ATN. ATN is a completely reversible process and patience is needed. An organ transplanted from an identical twin is not rejected as demonstrated by the first kidney transplantation performed in Boston in 1955 [13]. All other organ transplantations from one individual to another of the same species (an allograft) undergo recognition and rejection by the recipient’s immune system. Unless the immune response is effectively suppressed, the recipient’s immune system will destroy the graft. Recognition of a transplanted organ as ‘non‐self’ is caused by the recipient’s immune system recognising antigens on the cell surface of donor cells. These antigens are found in vertebrates on most nucleated cells and are determined by a set of genes on chromosome 6, called the ‘major histocompatibility complex’ (MHC). There are also minor histocompatibility (miH) factors, which can lead to graft rejection between siblings, even when all the known antigens of the MHC have been correctly matched. The genes of the MHC system are divided into classes I, II, and III. MHC class I proteins are cell surface glycoproteins consisting of two chains; the heavy chain activates CD8 T‐cells, and the light chain CD4 T‐cells. MHC class II proteins are expressed only on B‐cells, dendritic cells (DCs), and some endothelial cells. MHC class III genes encode for mediators such as tumour necrosis factors (TNF‐α and ‐β). One‐half of the MHC genes come from the father, and the other half from the mother. Siblings and parents, therefore, have half their MHC antigens in common (Figure 8.15). With two alleles at each MHC locus, most individuals can express six different MHC class I proteins and eight different MHC class II proteins. Combined with the polymorphism at this locus, this means for unrelated individuals that MHC‐identical donors and recipients are extremely rare. Even if they are found, miH antigens are invariably different. Complete identity is only possible with monozygotic twins. Figure 8.15 Inheritance of class I and II human leucocyte antigens (HLAs). Patients who have been exposed to MHC antigens through previous transplantation, transfusions, or pregnancy often develop antibodies against those MHC antigens (preformed antibodies). In humans, the MHC is called the ‘HLA system’ because it was first studied on white blood cells. The HLA system is a complex multigene family of more than 10 loci. The genes and their products are classified into two types: HLA class I and HLA class II. HLA class I genes are situated at the telomeric end of the 6p21.3 region of chromosome 6 (Figure 8.16). This region encodes the classic transplantation antigens HLA‐A, HLA‐B, and HLA‐C, which are expressed on nucleated cells. There are many other class I HLA loci. Figure 8.16 Diagram of chromosome 6. The HLA class II region consists of three main loci (i.e. HLA‐DR, HLA‐DQ, and HLA‐DP). The gene products are expressed on cells with immune functions (i.e. B‐cells, activated T‐cells, and antigen‐presenting cells) and is induced during inflammatory processes. Graft survival correlates with the number of HLA haplotypes shared by the donor and recipient. Therefore, HLA matching is done for organ allocation. However, there is large polymorphism of HLA proteins expressed because of variations of several or even single amino acids. This means that HLA specificities can be further subclassified by serological methods and even more so by DNA‐based typing methods. With cadaver donor transplantation, donor and recipient must have the same ABO blood group. Antibodies to the ABO blood group antigens are preformed or natural antibodies. ABO incompatibility between donor and recipient leads to hyperacute rejection. According to the frequency of the different ABO blood groups in the population, there is a shortage of A donors, and patients with this blood group on average have a longer waiting time for a kidney, especially if they are rhesus negative. In ABO‐incompatible live‐donor transplantation, the recipient’s ABO antibodies can be removed by repeated immunoadsorption and the use of B‐cell depleting drugs (rituximab) before transplantation. The later reappearance of these antibodies is generally not associated with rejection (i.e. accommodation) [14]. The number of HLA‐specificities that can be routinely tested is limited. These are the three class I antigens HLA‐A, HLA‐B, and HLA‐C and three class II types HLA‐DR, HLA‐DQ, and HLA‐DP. Because of the polymorphy of HLA‐antigens, this typing with broad specificity may result in ‘good matching’, but at a more specific level, several mismatches may actually be present [15]. More specific matching is not feasible for several reasons. The logistics of matching for such a diverse polymorphic antigenic system are complex; the increased ischemia time associated with an extensive matching process would lead to poorer outcomes, and there is a diminishing effect of HLA matching on transplantation outcome with modern more potent immunosuppressive regimens. However, even with limited matching, the chance of finding an HLA identical recipient is small. It is higher if there is a large pool of recipients with their HLA status kept on a central register. The kidneys are sent to the best matched available recipient. Complete identity of the six HLA loci tested (a ‘full house’ organ) is desirable. In practice, for most renal transplantations, there are between one and three mismatches. Patients with donor‐specific sensitising have a high risk of hyperacute rejection, but this has been virtually eliminated by routine cross‐matching. This consists of testing donor lymphocytes and recipient serum for preformed antibodies. There are different techniques of cross‐matching, and there is an ongoing debate about which preformed antibodies are damaging and which are not. In most cases, IgG HLA class I and class II‐specific antibodies represent a contraindication to transplantation. Highly sensitised patients are those with more than 85% of preformed antibodies. To find acceptable donors for such patients, ‘acceptable mismatch programs’ have been instituted by many programs defining minimal criteria (e.g. for the HLA‐DR locus). Another approach is allowing for paired live donor transplantation [16]. The response to a transplantation occurs in a series of relatively well‐defined stages. The first stage results from the severe physical assault that the organ is subjected to during harvest from the donor including the haemodynamic and neuroendocrine responses to brainstem death and transplantation into the recipient including many hours of preservation under artificial conditions. All these events sensitise the organ to ‘reperfusion injury’ when it is warmed rapidly on revascularization in the recipient. During and shortly after the ischemia and reperfusion periods, a variety of genes become activated and inflammatory cells begin to infiltrate the graft. The effectors of this first nonadaptive and not antigen‐specific response are part of the innate immune system (i.e. activated endothelial cells, macrophages, and interleukins [IL‐1, IL‐6]) as well as complement factors. The inflammatory response also triggers the migration of bone‐marrow derived donor DCs out of the graft. This early response does not constitute graft rejection, but the severity of the initial injury and the subsequent inflammatory reaction are central in the stimulation of antigen‐specific immunity later. A much damaged organ generates a strong ‘danger signal’, which initiates stronger responses when donor and recipient are antigenically different. The next phase is characterised by presentation of donor antigens to infiltrating recipient T‐cells which then become activated, proliferate, and differentiate to a variety of other cells, which infiltrate the graft and destroy it unless effectively suppressed (Figure 8.17). The antigens that stimulate graft rejection are cell surface MHC (HLA) antigens and numerous miH systems. Incompatibility for either MHC or miH antigens lead to an immune response, but rejection is stronger with MHC incompatibilities [17]. Figure 8.17 The immune response. Antigens from the transplant are taken up by macrophages and dendritic cells which present them to the helper T cells which produce lymphokines such as interleukins two (Il‐2) and four (IL‐4) and gamma interferon. For a strong immune response, MHC antigens need to be presented to the recipient. Bone‐marrow derived DCs, which migrate from the graft into the recipient, are essential (direct antigen presentation). They present MHC class I and class II antigens, produce various costimulants and cytokines, and stimulate T cells, especially CD4+ and CD8+ cells. Recipient DCs, which infiltrate the graft, are also capable of recognising donor MHC antigens and presenting this antigenic information to immune system effectors (indirect antigen presentation). Activation of T‐cells requires several costimulatory signals for downstream intracellular activation, such as CD28. Following this, B‐cells, DCs, and monocytes are activated. A response develops, which is either predominantly cell‐mediated or humoral. Which of the two happens is largely cytokine‐regulated. Interferon‐γ (IFN‐γ) is the prototypical cytokine, and if it dominates, the rejection will be cell‐mediated leading to the appearance of activated macrophages and specific circulating T‐cells. If IL‐4, ‐5, and ‐6 predominate, this will lead to humoral rejection. Thus, the local cytokine milieu seems to be important for determining which type of rejection occurs. Activated leucocytes migrate into the graft by adhering to the graft endothelium. This requires complex leucocyte‐endothelial interactions involving specific proteins (i.e. selectins, integrins, and immunoglobulins). Selectins control the first loose attachment of leucocytes (‘rolling’) along endothelial surfaces, which leads to the endothelial cells expressing IL‐8 and platelet‐activating factor. These lead to stronger leucocyte adhesion and arrest of the rolling process. L‐selectin and other proteins produced by the leucocytes enables extravasation. Chemokines from the graft such as α‐chemokines (which attract neutrophils and T‐cells) and β‐chemokines (which attract monocytes/macrophages, DCs, and natural killer [NK] cells) support graft infiltration. The immune response mounted depends on antibodies, unspecific cellular reactions as well as specific cytotoxic cells and cytokines. Antibodies can fix complement, especially complement component 4d (C4d), which recruits macrophages and activates endothelial cells. The point of entry, and therefore, the site of the most severe inflammatory reaction and destruction is the endothelial structure of the graft. Destruction of the small vessels of the graft occurs primarily and can be seen histologically while parenchymal changes are secondary to destruction of the vasculature. There are three types of rejection: hyperacute, acute, and chronic. Hyperacture rejection is due to preformed antibodies (donor‐specific HLA class I antibodies) and can occur intraoperatively or within hours postoperatively. With hyperacute rejection, the graft kidney swells, takes on a dark colour, and infarcts within minutes of its circulation being restored. Recipient antibodies against donor HLA antigens bind to the vascular endothelium of the graft, disrupt intercellular junctions, and induce the release of mediators leading to rapid uncontrollable activation of intravascular thrombotic and complement activation [18]. This results in intravascular coagulation and interstitial haemorrhage and destruction of the graft within minutes. Acute rejection occurs any time after about one week up to three months. It is a result of cell‐mediated immunity in the majority of cases, whereby the graft is infiltrated by large numbers of activated T‐cells (cellular rejection). Clinically, the kidney swells, becomes tender, and its function declines. There may be systemic symptoms such as fever, malaise, or hypertension. Acute rejection can also, less commonly, be predominantly mediated by antibodies (humoral rejection). Acute rejection can be initiated by failure to take the drugs, infection, or a blood transfusion. Transplant biopsy is required, showing dense cellular infiltration and oedema and can determine the type of rejection and its severity (i.e. Banff classification) [19]. Chronic rejection can occur any at any time after transplantation and is a gradual deterioration in renal function which does not improve when the dose of immunosuppressants is increased. Biopsy shows interstitial fibrosis and tubular atrophy, which are the hallmarks of chronic rejection; there is also intimal fibrosis of arteries with hyalinization of glomeruli. Chronic rejection is a slow process which seems to occur in many renal transplants over the years and is held responsible for the slow but steady decline of transplant function starting many months or years after successful transplantation. It underlies the late graft loss seen in many patients [20]. Immunosuppressive treatment is always a combination of several drugs to maximise immune suppression; however, it increases the likelihood of side effects. Steroids reduce T‐cell proliferation and inhibit the production of IL‐2 and of Il‐1 and IL‐6 in macrophages. They also inhibit the migration of monocytes to areas of inflammation such as acute rejection. Prednisone and prednisolone are metabolised in the liver where prednisone is converted to prednisolone. Their half‐lives are increased in hepatic disease and shortened if taken together with drugs that induce hepatic enzymes. The sensitivity of lymphocytes to steroids can vary individually as well as under the influence of other immunosuppressants, accounting for the so‐called ‘steroid resistance’ seen in some patients. Side effects of steroids are numerous, and considerable and most protocols today aim at tapering the steroid dose shortly after transplantation or using a steroid‐free maintenance protocol. Large‐dose steroid treatment is used in acute rejection. Azathioprine was used until cyclosporine became available. It is a derivative of mercaptopurine, is activated by hepatic metabolism, and inhibits purine synthesis, blocking DNA and RNA synthesis in lymphocytes and IL‐2 production. It is catabolised by xanthine oxidase, and thus, there is an interaction with allopurinol. Azathioprine has been largely replaced by mycophenolate, but it is an inexpensive drug still used in many countries. Cyclosporine became available in the 1980s and revolutionised renal transplantation by markedly improving graft survival. It is a fungal product and inhibits CD4+ T‐helper cells, preventing IL‐2 and cytotoxic T‐cell induction. Cyclosporine binds to an intracellular protein (i.e. cyclophilin A), which inhibits a Ca2+‐dependent phosphatase (i.e. calcineurin). Calcineurin is part of an intracellular signalling pathway leading to the expression of IL‐2. Tacrolimus also binds to an intracellular protein (FK BP‐12), which inhibits calcineurin, blocking T‐cell activation and IL‐2 production. It is 10–100 times more potent than cyclosporine. Oral bioavailability is limited (25–30%) and 99% is plasma protein‐bound. Long‐term graft survival with tacrolimus is better than with cyclosporine [21]. Both calcineurin inhibitors are given orally, and drug levels have to be controlled. Hepatic clearance (cytochrome P450) leads to numerous drug interactions. Calcineurin inhibitors are nephrotoxic. This can occur early in kidneys with marked ischemic damage or within weeks associated with high serum levels, or there can be late, chronic nephrotoxicity, leading to progressive loss interstitial graft fibrosis. Mycophenolic acid (MPA), also a fungal product, inhibits the enzyme inosine monophosphate dehydrogenase (IMPDH) and thereby the synthesis of guanosine nucleotides in T‐ and B‐cells. A synthetic analogue of MPA, mycophenolate mofetil (MMF), has better oral bioavailability. MMF is rapidly metabolised to an inactive glucuronide, but has a long half‐life (17 hours), but plasma levels need to be monitored. Gastrointestinal side effects (diarrhoea, indigestion, and reflux) and bone marrow toxicity occur. With MMF, viral infections are more common. Sirolimus and everolimus block T‐cell proliferation by inhibiting the mammalian target of rapamycin (mTOR) [22]. Sirolimus (rapamycin) is the product of a microorganism first isolated from soil from the Easter Island (Rapa Nui), giving it its name, and everolimus is a synthetic analogue. Both bind to an intracellular immunophilin (FK506‐binding protein) which forms a complex with a serine‐threonine kinase (mTOR). This is a key point in the cell cycle regulatory pathway. Both drugs block lymphocyte proliferation and IL‐2 transcription and inhibit tumour cell growth. The incidence of posttransplant malignancy is reduced. Specific side effects are changes in lipid metabolism, delayed graft function, bone marrow suppression, and disturbed wound healing [23]. Sirolimus has a long half‐life (60 hours), but everolimus has a short half‐life (16–18 hours). Both drugs are given orally. Dosing should be concentration controlled and metabolism is also by cytochrome P450 (CYP3A4). Antibodies are used for the treatment of rejection. By immunising animals with human lymphocytes, heterogenous polyclonal sera can be obtained, which contain many antibodies with largely undefined specificities (i.e. antithymocyte globulin [ATG]). Polyclonal antibodies react with many different antigens and the animal proteins can cause allergic or anaphylactic responses. Fever, urticaria, rash, and headaches occur. The physiological half‐life of these antibodies is several weeks. With cell hybridization, monoclonal antibodies (mAb), which suppress T‐cells and their subsets, are available. MAb preparations contain a single specific antibody and their action is more predictable. OKT3 (muromumab, murine anti‐CD3) is an IgG2a mouse antibody binding human CD3. It mediates complement‐dependent cell lysis and rapidly clears T‐cells from the circulation. Polyclonal and monoclonal preparations with anti‐T‐cell antibodies are used for the treatment of steroid‐resistant rejection. Other specific mAbs are anti‐CD25 (daclizumab, basiliximab), anti‐CD25 (alemtuzumab), and anti‐CD20 (rituximab) which can be used for induction and rescue treatment. The B‐cell depleting effect of rituximab is used for ABO‐incompatible transplantation and for the treatment of humoral rejection. Belatacept is a fusion protein and acts as a selective blocker of T‐cell‐costimulation. It binds to CD80 and CD86 on antigen‐presenting cells. This blocks the CD28‐costimulation of T‐cell activation [24]. It is given as an induction treatment and during the first weeks after transplantation. Ebstein‐Barr virus infections and posttransplantation lymphoproliferative disorder (PTLD) seem to occur more frequently with belatacept. All immunosuppressive agents have side effects which occur with time even after years of treatment. Azathioprine causes bone marrow depression. Corticosteroids induce diabetes, osteoporosis, obesity, and other unwanted effects. MMF can cause leucopenia and thrombocytopenia. Tacrolimus is diabetogenic. Calcineurin inhibitors can provoke hypertension and are nephrotoxic. mTOR inhibitors cause gastrointestinal problems and disturbances in wound healing. Infections and the development of malignancies are more common with immunosuppression. Infections with ubiquitous viruses (i.e. cytomegaly, herpes simplex, zoster, and BK virus) are common after renal transplantation, especially with MMF treatment. Urinary tract infections can lead to pyelonephritis and graft dysfunction. Infectious agents not dangerous to immunocompetent hosts can be life‐threatening in transplant recipients (e.g. pneumocystis). The incidence of de novo malignancies is increased about 10‐fold with immunosuppression, the most common being skin cancers. The risk of malignancy is proportional to the immunosuppressive potency but lowest with mTOR inhibitors. A specific malignant disorder due to immunosuppression is the PTLD. Immunosuppressive treatment used can be characterised as induction, maintenance, or rescue therapies. Induction immunosuppression is intensive treatment used to suppress immune responsiveness at the time of transplantation. Maintenance treatment is less potent but is tolerable for long‐term use in a steady state. Rescue therapy is used with acute rejection and is – like induction therapy – intense and fairly toxic so that it cannot be given for prolonged periods of time. Induction treatment is started before the transplantation procedure and continued for the first week. It is used in patients at high risk of rejection (e.g. second transplantations, preformed antibodies, high number of HLA mismatches). Monoclonal or polyclonal antibodies can be used. Induction treatment should be an individualised decision because side effects can be severe. This consists of a combination with the early use of steroids tapered to a long‐term low‐dose or steroid‐free regimen. Combinations of two or three drugs of different classes are used. Most centres have their preferred maintenance regimen based on experience which is adjusted to individual circumstances (Table 8.6). The treatment of rejection requires biopsy, which will determine which type of rejection is present. First‐line treatment is by high dose steroids for three days. Failing this, humoral rejection requires plasmapheresis and perhaps rituximab. Second‐line treatment for cellular rejection is by antibodies (i.e. ATG, OKT3). In addition, the maintenance immunosuppressant may need to be changed [25]. Successful renal transplantation restores fertility and successful pregnancy is possible. Conception is possible after the first transplant year, provided immunosuppression is stable, and there have been no rejections. Hypertension during pregnancy is a common problem in transplant patients. Delivery can be vaginal, but obstetricians usually prefer caesarean section. There are very few data on any effects of immunosuppression on intrauterine development or during breast feeding. Patients who have had a transplant need close medical care. Immunosuppression and compliance with treatment, especially in children and adolescents, need to be supervised. Complications such as infections need early treatment. Bladder function is critical especially in patients who are older. Patients need to be regularly screened for de novo malignancies. Urothelial upper tract tumours of the native kidneys are relatively common. Maintenance immunosuppression requires blood level measurements at defined intervals.
Renal Transplant
Abstract
Focal glomerulosclerosis
30–50%
Membrano‐proliferative glomerulonephritis
30–50%
IgA nephropathy
40–60%
Hemolytic uremic syndrome (HUS)
50–75%
Diabetic nephropathy
80–100%
Oxalosis (primary hyperoxaluria)
80–100%
8.1 The Patients and the Kidneys
8.1.1 Organs for Transplantation
Croatia
Spain
Belgium
US
France
Portugal
Slovenia
Austria
Italy
Finland
Czech Republic
UK
Ireland
Poland
The Netherlands
Sweden
Canada
Hungary
Denmark
Germany
Switzerland
Luxembourg
Greece
36,5
35,1
32,9
25,6
24,9
24,2
23,0
22,5
22,4
19,9
19,8
18,3
17,0
16,1
15,8
15,6
14,7
14,3
13,4
12,8
12,0
7,9
7,0
8.1.2 Organ Allocation
8.1.3 Waiting Lists and Preparation of the Recipient
Malignancy
Cancer‐free years required
Renal
< 5 cm
> 5 cm
Wilms tumour
2
5
2
Prostate
2
Bladder
Non‐muscle invasive
Muscle‐invasive
2
5
Colorectal
2–5
Uterus
Cervical
Endometrium
2–5
2
Breast
2–5
Lymphoma
2–5
Skin
Basal cell
Squamous
Melanoma
None
None
5
8.1.4 Selecting Donors
Untreated bacterial sepsis
Hepatitis B and C positive
HIV, CMV, EBV, syphilis positive, TB
Chronic renal disease
Active systemic renal disease (e.g. systemic lupus erythematosus)
Potentially metastasizing malignancy
Severe hypertension
Severe diabetes
Significant cardiopulmonary or vascular disease
Acute renal failure with oliguria
Intravenous drug users or alcohol abuse
Loss of cerebral function
Absent brainstem function
No pupillary or corneal reflex
No tracheobronchial reflex
No oculocephalic reflex
No pain response in cranial nerve segments
No seizures or posturing
Apnoea in response to acidosis
Irreversible comatose state
No sedating, paralysing or toxic drugs
No profound hypothermia
No gross electrolyte or endocrine disturbance
8.1.5 Kidneys from Living Donors
8.2 Technique of Removal of Donor Kidneys
8.2.1 Cadaver Donor
8.2.2 Living Donor
8.2.2.1 Preservation of the Kidney
Dehydration
Arterial stenosis
Venous thrombosis
Urine leak
Ureteral obstruction
Catheter obstruction
Acute rejection
Acute tubular necrosis
Nephrotoxicity of immunosuppression
8.2.2.2 Inserting the Kidney
8.2.2.3 Postoperative Complications
8.2.2.4 Delayed Graft Function
CyA, MMF, and steroids
CyA, sirolimus, and steroids
Tacrolimus, sirolimus, and steroids
Tacrolimus, MMF, and steroids
8.3 Immunology of Organ Transplantation
8.3.1 The Major Histocompatibility Complex
8.3.2 The Human Leucocyte Antigen System
8.3.3 The ABO Blood Group System
8.3.4 HLA‐Typing in Renal Transplantation
8.3.5 Cross‐Matching and Preformed Antibodies
8.4 Graft Rejection
8.4.1 Reperfusion Injury
8.4.2 Adaptive Immunity
8.4.3 Antigen Presentation
8.4.4 Types of Immune Response
8.4.5 Migration of Activated Cells
8.4.6 Graft Destruction
8.4.7 Clinical Types of Rejection
8.4.8 Hyperacute Rejection
8.4.9 Acute Rejection
8.4.10 Chronic Rejection
8.5 Immunosuppression
8.5.1 Corticosteroids
8.5.2 Azathioprine
8.5.3 Calcineurin Inhibitors: Cyclosporine and Tacrolimus
8.5.3.1 Cyclosporine
8.5.3.2 Tacrolimus
8.5.4 Mycophenolate Mofetil (MMF)
8.5.5 mTOR Inhibitors
8.5.6 Antibodies
8.5.7 Belatacept
8.5.8 Side Effects of Immunosuppression
8.5.9 Immunosuppressive Treatment Regimens
8.5.9.1 Induction Treatment
8.5.9.2 Maintenance Treatment
8.5.10 Treatment of Rejection
8.5.11 Pregnancy after Renal Transplantation
8.5.12 Long‐Term Treatment of the Recipient after Renal Transplantation
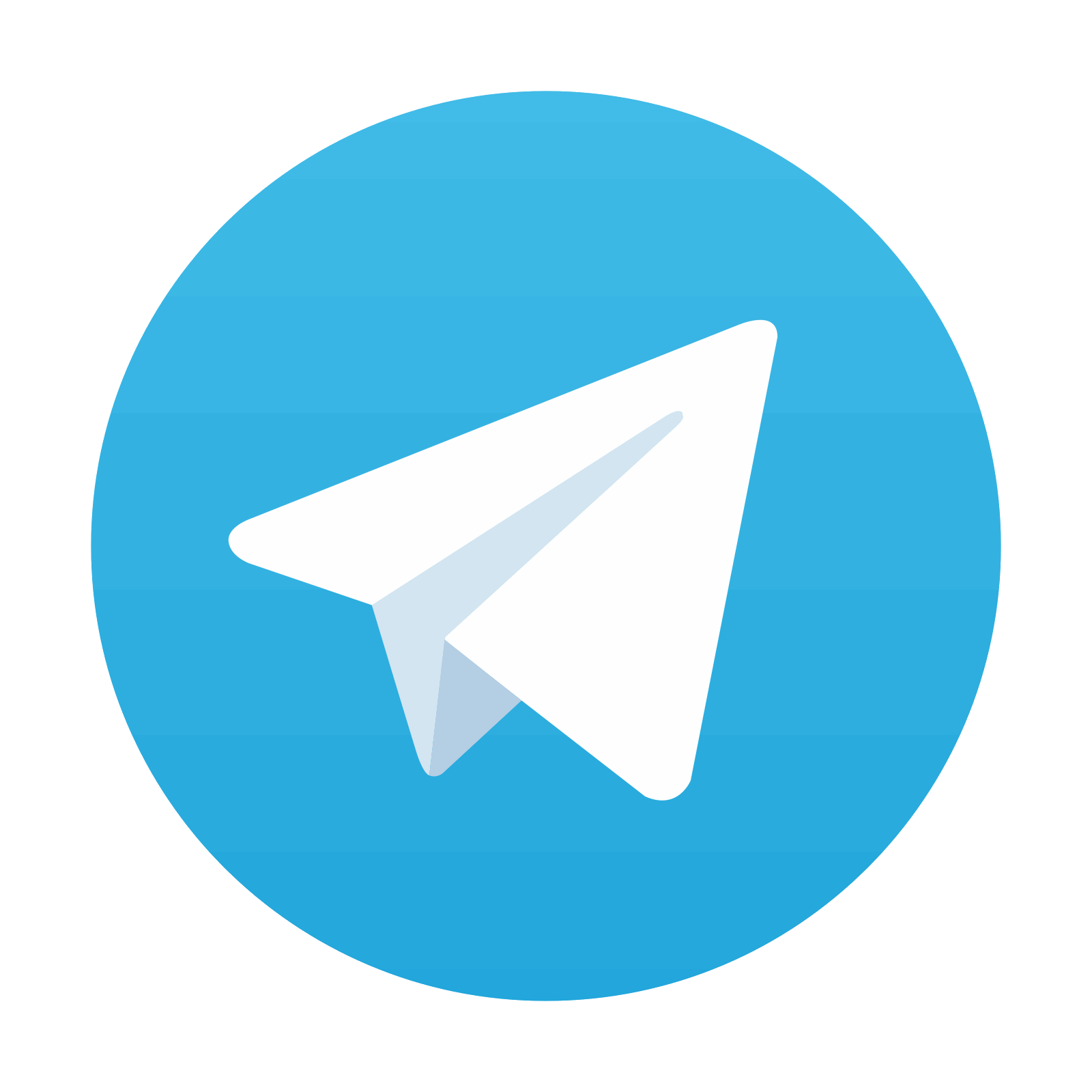
Stay updated, free articles. Join our Telegram channel

Full access? Get Clinical Tree
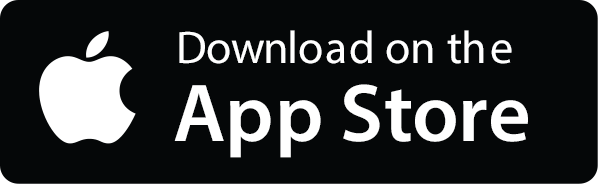
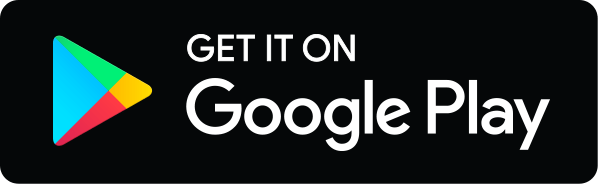