Renal Sodium Excretion, Edematous Disorders, and Diuretic Use
Robert W. Schrier
An understanding of body fluid volume regulation, as modulated by renal sodium and water excretion, is critical for the practice of clinical medicine. Knowledge of the intrarenal and extrarenal factors affecting renal sodium excretion is important to comprehend the mechanism of body fluid volume regulation in health and disease because the sodium ion is the primary determinant of extracellular fluid (ECF) volume. In this regard, the edematous disorders—cardiac failure, liver disease, and the nephrotic syndrome—present a particular challenge to our understanding of body fluid volume regulation. In normal humans, if the ECF volume is expanded by the administration of isotonic saline, then the kidney excretes the excess amount of sodium and water in the urine, thus returning the ECF volume to normal. However, in these edematous states, avid renal sodium and water retention persists despite expansion of ECF volume and the presence of total body sodium and water excess. In circumstances where advanced kidney disease is present and renal function and excretory capacity are diminished (e.g., acute or chronic intrinsic renal failure), it is obvious why the decreased glomerular filtration rate (GFR) may be associated with retention of sodium and water to the point of pulmonary and/or peripheral edema. However, it is clear that the integrity of the kidney as the ultimate effector organ of body fluid volume regulation is intact in patients with heart failure or liver disease and some patients with the nephrotic syndrome. Thus, the kidney must be responding to extrarenal signals from the afferent limb of a volume regulatory system in these edematous disorders. The study of these edematous disorders has led to our proposal of a unifying hypothesis of body fluid volume regulation that applies to both health and disease (1, 2, 3, 4, 5, 6, 7, 8). The purpose of this chapter is to review the afferent and efferent mechanisms that determine renal sodium and water handling, particularly in the context of the edematous disorders, and discuss the treatment of edema with diuretic agents.
Sodium Ion as Determinant of ECF Volume
Sodium ions reside primarily in the ECF compartment to which they are extruded from cells by active transport mechanisms. These transport processes result in an intracellular sodium concentration of 10 mEq/L and an ECF sodium concentration of 145 mEq/L. The sodium ion and its major anions, chloride and bicarbonate, constitute >90% of the total solute in the ECF space. Thus, total body sodium and its accompanying anions are the osmotically active solutes that are the major determinants of ECF volume. In turn, the regulation of sodium balance is determined by the relationship among sodium intake, extrarenal sodium loss, and renal sodium excretion. Practically, renal sodium excretion may be considered to be the primary determinant of sodium balance because the kidney is able to excrete virtually sodium-free urine as well as rapidly excrete large sodium loads in response to diminished or increased sodium intakes, respectively.
A positive sodium balance is associated with increased amounts of sodium, located predominantly in the ECF compartment. Because cellular membranes are freely permeable to water, the osmotic gradient created by the addition of ECF sodium causes water to move from cells into the ECF compartment, thus expanding ECF volume. In addition, an increase in ECF osmolality stimulates the hypothalamic thirst center and leads to increased fluid intake and also releases arginine vasopressin (AVP) from the posterior pituitary, which decreases renal water excretion by increasing the water permeability of collecting duct epithelium (9). The latter two effects of an increased ECF osmolality result in a positive water balance, and the combined influence of positive sodium and water balances leads to further expansion of ECF volume. If this expansion of ECF is of sufficient magnitude, then an alteration of the Starling forces that govern the transfer of fluid from the vascular compartment to the surrounding interstitial spaces occurs and edema results (10). Conversely, a negative sodium balance results in a depletion of ECF volume. A decrease in ECF volume may result in a parallel decline in plasma volume. Maintenance of ECF volume and plasma volume is necessary for adequate circulation and survival of the organism. Thus, renal sodium and water retention is clearly appropriate in situations of ECF volume depletion. However, in edematous disorders, continued renal sodium and water retention despite total body sodium and water excess defines a paradoxical clinical situation.
It is worth mentioning that the osmolality of ECF is regulated by the AVP-thirst-renal axis (as discussed in depth in Chapter 1). However, the osmolality of the ECF is not a reliable index of ECF volume. ECF volume and its determinant total body sodium are best assessed by physical examination and determination of urinary sodium concentration. For example, a finding of generalized edema suggests an expanded ECF volume and increased total body sodium. Conversely, orthostatic tachycardia and/or hypotension, flat neck veins, and decreased skin turgor suggest depletion of ECF volume and decreased total body sodium. In fact, alterations in the osmolality of the ECF can occur in association with normal, increased, or decreased ECF volume (Chapter 1).
In summary, the control of ECF volume is dependent on the regulation of sodium balance. The kidneys play the pivotal role in the regulation of sodium balance and therefore of ECF volume homeostasis. In certain edema-forming states associated with a normal GFR, the kidney retains sodium and water despite expansion of the ECF volume and total body sodium and water. A knowledge of the afferent (“sensor”) and efferent (“effector”) mechanisms of sodium and water retention associated with the edematous disorders forms the basis of our understanding of body fluid volume regulation.
Afferent Mechanisms Involved in Body Fluid Volume Regulation
THE CONCEPT OF “EFFECTIVE BLOOD VOLUME” OR WHAT COMPARTMENT IS SENSED?
If the afferent receptors of body fluid volume regulation primarily sense total blood volume, then the kidneys of edematous patients should increase their excretion of sodium and water since their total blood volumes are increased. However, as mentioned, this does not occur in patients with advanced cardiac failure, liver disease, or the nephrotic syndrome. Thus, there must be some body fluid compartment that is still “underfilled”—even in the presence of expansion of total ECF and blood volumes—and comprises the afferent limb of renal sodium and water retention in patients with edematous disorders. In 1948, Peters coined the enigmatic term effective blood volume as a reference to such an underfilled body fluid compartment (11). Accordingly, extrarenal signals must be initiated by this decrease in effective blood volume, which enhances tubular sodium and water reabsorption by the otherwise normal kidney. In this regard, it is clear that renal sodium and water retention can occur in patients with cardiac failure or cirrhosis and in some patients with the nephrotic syndrome before any diminution in GFR.
Borst and deVries (12) first suggested cardiac output as the primary modulator of renal sodium and water excretion. In this context, the level of cardiac output would constitute the effective blood volume and thus serve as the primary stimulus for renal sodium and water retention in patients with edematous disorders. Although this concept is appealing, substantial renal sodium and water retention may occur in the presence of an increase in cardiac output. For example, a significant elevation in cardiac output may occur in the presence of avid renal sodium and water retention and expansion of ECF volume in association with cirrhosis, pregnancy, arteriovenous (AV) fistulas, and other causes of high-output cardiac failure, such as thyrotoxicosis and beriberi. Consequently, there must exist some other or additional determinant(s) of effective blood volume.
PRIMACY OF THE ARTERIAL CIRCULATION IN VOLUME REGULATION
The unifying hypothesis of body fluid volume regulation in health and disease states that the fullness of the arterial vascular compartment or the so-called effective arterial blood volume (EABV) is the primary determinant of renal sodium and water excretion (1, 2, 3, 4, 5, 6, 7, 8). In a 70-kg man, total body water approximates 42 L, of which only 0.7 L (1.7% of total body water) resides in the arterial circulation. From a teleologic viewpoint, it is attractive to propose that the primacy for regulation of renal sodium and water excretion, and body fluid volume homeostasis, is modulated by the smallest body fluid compartment—thus endowing the system with exquisite sensitivity to relatively small changes in body fluid volume. Another advantage of the integrity of the arterial circulation constituting the main afferent sensing compartment for body fluid volume regulation is that perfusion of the vital organs is dependent on the arterial circulation. As a result, total ECF, interstitial fluid, or total intravascular volumes are not primary determinants of renal sodium and water excretion, and the venous component of intravascular volume likewise is excluded as the primary determinant of sodium and water excretion, because all of these body fluid compartments may be expanded while the renal sodium and water retention persists in edematous patients. It is acknowledged, however, that there are experimental and clinical circumstances in which selective rises in right and left atrial pressure stimulate the release of atrial natriuretic peptide (ANP) (13) or suppression of AVP (14), respectively, which may enhance sodium and water excretion. These events, however, must be subservient to the more potent determinants of the arterial circulation because the patient with advanced left or right ventricular dysfunction, or both, exhibits avid sodium and water retention despite markedly elevated atrial and ventricular pressures.
CARDIAC OUTPUT AND SYSTEMIC ARTERIAL RESISTANCE AS THE DETERMINANTS OF THE FULLNESS OF THE ARTERIAL CIRCULATION AND RENAL SODIUM AND WATER EXCRETION
The EABV is a measure of the adequacy of arterial blood volume to “fill” the capacity of the arterial circulation. Normal arterial filling exists when the ratio of cardiac output to systemic vascular resistance maintains venous return and cardiac output at normal levels. Thus, arterial underfilling may be initiated by either a decrease in cardiac output or a fall in systemic arterial resistance (i.e., arterial vasodilatation, which increases the holding capacity of the arterial vascular tree). Arterial underfilling results in unloading of high-pressure baroreceptors with subsequent activation of the three major neurohormonal vasoconstrictor systems—namely, the sympathetic nervous system, the renin-angiotensin-aldosterone system, and the nonosmotic release of AVP—which diminish renal hemodynamics and promote renal sodium and water retention. This hypothesis accounts for the initiation of sodium and water retention in low- and high-output cardiac failure, liver disease, and other states of arterial underfilling (Figs. 2-1 and 2-2).
AFFERENT VOLUME RECEPTORS
As mentioned, the afferent volume receptors for such a volume regulatory system must reside in the arterial vascular tree, such as the high-pressure baroreceptors in the carotid sinus, aortic arch, left ventricle, and juxtaglomerular apparatus. Although the low-pressure volume receptors of the thorax (cardiac atria, right ventricle, and pulmonary vessels) must be of some importance to the volume regulatory system (15, 16), there is considerable evidence that arterial receptors can predominate over low-pressure receptors in volume control in mammals.
High-Pressure Volume Receptors
In humans, the presence of volume-sensitive receptors in the arterial circulation was first suggested by Epstein et al. (17) based on observations made in patients with traumatic AV fistulas. Closure of traumatic AV fistulas was associated with an immediate increase in renal sodium excretion independent of concomitant changes in either GFR or renal blood flow (RBF) (17). Closure of AV fistulas is associated with a decreased rate of emptying of the arterial blood into the venous circulation, as demonstrated by closure-induced increases in diastolic arterial pressure and decreases in cardiac output (17). Further evidence implicating the relative “fullness” of the arterial vascular tree as being the major sensor in modulating renal sodium excretion can be found in denervation experiments. In these studies, surgical or pharmacologic interruption of sympathetic
efferent neural pathways emanating from high-pressure areas inhibited the natriuretic response to volume expansion (18, 19, 20). Moreover, reduction of pressure or stretch at the carotid sinus, similar to that produced by decreased cardiac output or arterial hypotension, has been shown to activate the sympathetic nervous system and promote renal sodium and water retention (21). High-pressure baroreceptors also appear to be important factors in regulating nonosmotic release of AVP and thus renal water excretion (22). One of the best-defined high-pressure receptors that are known to act in an appropriate manner to maintain constancy of the EABV is the renal afferent arteriolar baroreceptor (i.e., juxtaglomerular apparatus). This baroreceptor is an important factor in the control of renal renin secretion and consequently angiotensin II formation and aldosterone synthesis and release (23). The vasoconstrictor and sodium-retaining effects of angiotensin II and sodium-retaining effect of aldosterone then act to restore the fullness of the arterial circulation.
efferent neural pathways emanating from high-pressure areas inhibited the natriuretic response to volume expansion (18, 19, 20). Moreover, reduction of pressure or stretch at the carotid sinus, similar to that produced by decreased cardiac output or arterial hypotension, has been shown to activate the sympathetic nervous system and promote renal sodium and water retention (21). High-pressure baroreceptors also appear to be important factors in regulating nonosmotic release of AVP and thus renal water excretion (22). One of the best-defined high-pressure receptors that are known to act in an appropriate manner to maintain constancy of the EABV is the renal afferent arteriolar baroreceptor (i.e., juxtaglomerular apparatus). This baroreceptor is an important factor in the control of renal renin secretion and consequently angiotensin II formation and aldosterone synthesis and release (23). The vasoconstrictor and sodium-retaining effects of angiotensin II and sodium-retaining effect of aldosterone then act to restore the fullness of the arterial circulation.
Low-Pressure Volume Receptors
Low-pressure sensors also may have an important role to play in body fluid volume regulation because the more compliant venous side of the circulation contains up to 85% of the total blood volume at any given time (Table 2-1). In fact, a variety of maneuvers that decrease thoracic venous return, such as prolonged standing (24), lower-extremity tourniquets (25, 26), and positive pressure breathing (27), are associated with diminished renal sodium excretion. Conversely, maneuvers that augment venous filling, such as recumbency (28) and negative pressure breathing (29), are associated with increased renal sodium excretion. Moreover, a direct correlation between renal sodium excretion and left atrial pressure has been demonstrated in dogs, suggesting a role for an atrial receptor as one type of intrathoracic sensor (30). Immersion in water to the neck or so-called head-out water immersion results in a pressure gradient from 80 mm Hg at the foot to 0 mm Hg at water level. This maneuver increases venous return to the heart. In response to head-out water immersion, a profound increase in renal excretion of salt and water occurs independent of major changes in either GFR or renal hemodynamics (31). As first suggested by Gauer et al. (29) and Henry et al. (32), physiologically significant left atrial receptors have been shown to contribute to ECF volume regulation by exerting nonosmotic control over AVP secretion and thus over renal water excretion. In addition, the atria have been demonstrated to be the site for synthesis, storage, and release of vasoactive and natriuretic humoral agents (33, 34).
Thus, increased filling of the thoracic vascular and cardiac atria would be expected to signal the kidney to increase urinary sodium excretion in order to return the blood volume to normal. However, in the setting of chronic heart failure, renal sodium and water retention occur despite increased atrial pressure, which loads the low-pressure baroreceptors. Thus, in low-output chronic heart failure, diminished cardiac output must exert the predominant effect via unloading of high-pressure arterial baroreceptors. Chronic studies in animals employing experimental tricuspid insufficiency (35) further support this hypothesis. The increase in right atrial pressure was associated with avid renal sodium retention in these animal models. However, a concomitant fall in cardiac output likely explains the sodium retention.
Zucker et al. (36) have demonstrated that the inhibition of renal sympathetic nerve activity that is seen during acute left atrial distention is lost during chronic heart failure in dogs. Moreover, a decrease in cardiac preload fails to produce the expected parasympathetic withdrawal and sympathetic activation in humans with heart failure (37). These findings are also consistent with the observation of a strong positive correlation between left atrial pressure and coronary sinus norepinephrine, a marker of cardiac adrenergic activity, in
patients with chronic heart failure (38). Taken together, these findings suggest that the normal inhibitory control of sympathetic activation accompanying increased atrial pressures is lost in heart failure patients and somehow may even be converted to a stimulatory signal.
patients with chronic heart failure (38). Taken together, these findings suggest that the normal inhibitory control of sympathetic activation accompanying increased atrial pressures is lost in heart failure patients and somehow may even be converted to a stimulatory signal.
Table 2-1 Body Fluid Distribution | ||||||||||||||||||||||||
---|---|---|---|---|---|---|---|---|---|---|---|---|---|---|---|---|---|---|---|---|---|---|---|---|
|
In summary, the afferent or sensor mechanisms for sodium and water excretion may be preferentially located on the arterial side of the circulation where diminished fullness of the arterial vascular tree owing to decreased cardiac output or systemic arterial vasodilation results in unloading of high-pressure receptors and subsequent renal sodium and water retention. Reflexes from low-pressure volume receptors may also be altered so as to influence renal sodium and water handling. In any event, changes in systemic and renal hemodynamics and activation of various neurohormonal systems largely comprise the efferent limb of the volume regulatory system.
Efferent Mechanisms Involved in Body Fluid Volume Regulation
THE NEUROHORMONAL RESPONSE TO ARTERIAL UNDERFILLING
Arterial underfilling secondary to a diminished cardiac output or systemic arterial vasodilation elicits a number of compensatory neurohormonal responses that act to maintain the integrity of the arterial circulation by promoting systemic vasoconstriction as well as expansion of the ECF volume through renal sodium and water retention. As noted, the three major neurohormonal vasoconstrictor systems activated in response to arterial underfilling are the sympathetic nervous system, renin-angiotensin-aldosterone system, and nonosmotic release of AVP. Baroreceptor activation of the sympathetic nervous system appears to be the primary integrator of the hormonal vasoconstrictor systems involved in the volume control system because the nonosmotic release of AVP involves sympathetic stimulation of the supraoptic and paraventricular nuclei in the hypothalamus (39), and activation of the renin-angiotensin-aldosterone system involves renal β-adrenergic stimulation (40). Thus, in low-output cardiac failure, diminished integrity of the arterial circulation as determined by decreased cardiac output causes unloading of arterial baroreceptors in the carotid sinus and aortic arch. Systemic arterial vasodilation produces unloading of these arterial baroreceptors in the setting of high-output cardiac failure, cirrhosis, and other states of arterial underfilling. This baroreceptor inactivation results in diminution of the tonic inhibitory effect of afferent vagal and glossopharyngeal pathways to the central nervous system (CNS) and initiates an increase in sympathetic efferent adrenergic tone with subsequent activation of the renin-angiotensin-aldosterone system. Various counterregulatory, vasodilatory hormones may also be activated in heart failure, including natriuretic peptides and vasodilating renal prostaglandins. Activation of these various neurohormonal vasoconstrictor and vasodilator systems substantially determines renal sodium and water handling in the edematous disorders and comprises a major part of the efferent limb of body fluid volume regulation. The pathogenesis of sodium and water retention associated with cardiac failure, liver disease, and the nephrotic syndrome are now reviewed in the context of the unifying arterial underfilling hypothesis of body fluid volume regulation.
PATHOGENESIS OF SODIUM AND WATER RETENTION IN CARDIAC FAILURE
Sodium and water retention and resultant edema formation are cardinal features of chronic cardiac failure. In fact, the inability to excrete a sodium load has been used as an index of the presence of heart failure (41), and a defect in water excretion is encountered routinely in such patients (42). Two theories have been proposed to explain the renal response to cardiac failure. The “backward” theory of heart failure, proposed in 1832, suggests that increased venous hydrostatic pressure owing to increased ventricular filling pressures causes edema by promoting transudation of fluid from the intravascular to the interstitial compartment, resulting in edema formation (43). The reduced intravascular volume then signals the kidneys to retain sodium and water, further exacerbating the venous hypertension and formation of edema. The alternative “forward” theory of cardiac failure suggests that a primary decrease in cardiac output activates afferent and efferent pathways and results in renal sodium retention (44). As pointed out by Smith (26), these theories are not mutually exclusive and both are operant in the pathophysiology of heart failure because both central venous hypertension and arterial underfilling are implicated in the afferent limb of body fluid volume regulation. Nevertheless, the dominant signal for sodium and water retention in cardiac failure appears to occur in the arterial circulation. Decreased cardiac output is the cause of the arterial underfilling in the case of low-output
heart failure, whereas systemic arterial vasodilation initiates the afferent limb of sodium and water retention in high-output cardiac failure (Fig. 2-1).
heart failure, whereas systemic arterial vasodilation initiates the afferent limb of sodium and water retention in high-output cardiac failure (Fig. 2-1).
Renal Hemodynamics in Cardiac Failure
Glomerular Filtration Rate
Many early investigators believed that the cause of sodium retention in heart failure was a decrease in GFR; however, studies failed to confirm such a correlation. In fact, GFR is often normal in early heart failure and may even be elevated in states of high-output cardiac failure. It is acknowledged, however, that the contribution of GFR to sodium balance is difficult to evaluate because very minute changes in GFR could lead to substantial changes in sodium excretion if absolute sodium reabsorption remained unchanged. Nevertheless, although GFR may be diminished in patients with advanced heart failure, an increase in tubular sodium reabsorption undoubtedly is an important cause of sodium and water retention in cardiac failure.
Renal Blood Flow
Heart failure is commonly associated with an increase in renal vascular resistance and a decrease in RBF. In general, RBF decreases in proportion to the decrease in cardiac output. Some investigators have also shown a redistribution of RBF from outer cortical nephrons to juxtamedullary nephrons in experimental heart failure (45). It was proposed that deeper nephrons with longer loops of Henle reabsorb sodium more avidly; thus, the redistribution of blood flow to these nephrons with heart failure would result in renal sodium retention. However, other investigators have not been able to demonstrate such a redistribution of blood flow in other models of cardiac failure (46). Thus, the role of redistribution of RBF in the sodium retention of cardiac failure remains uncertain.
Filtration Fraction
Filtration fraction is often increased in heart failure because RBF falls as cardiac output decreases and GFR is preserved. An increase in filtration fraction results in increased protein concentration and oncotic pressure in the efferent arterioles and peritubular capillaries that surround the proximal tubules. Such an increase in peritubular oncotic pressure has been proposed to increase sodium and water reabsorption in the proximal tubule. These changes in renal hemodynamics and filtration fraction, which favor proximal tubular sodium reabsorption, are primarily a consequence of constriction of the efferent arterioles within the kidney. These renal hemodynamic changes are mediated mainly by activation of neurohormonal vasoconstrictor systems because both activation of renal nerves and increased circulating norepinephrine and angiotensin II have been implicated in efferent arteriolar vasoconstriction (47, 48). In addition, decreased activity of such substances as vasodilating renal prostaglandins also may play a role in renal vasoconstriction (49).
Of note, micropuncture studies in dogs with vena caval constriction and AV fistulas have demonstrated the importance of distal nephron sites of increased sodium reabsorption. Increased filtration fraction primarily affects proximal tubular sodium reabsorption. Thus, although clearance and micropuncture studies in animals with heart failure have demonstrated increased sodium reabsorption in the proximal tubule (50), distal sodium reabsorption also seems to be involved. Furthermore, changes in filtration fraction have been observed in heart failure long before changes in sodium balance occur, questioning the dominance of peritubular factors and proximal reabsorption in the sodium retention of cardiac failure.
The Sympathetic Nervous System in Cardiac Failure
The sympathetic nervous system is unquestionably activated in patients with heart failure. Various studies have demonstrated elevated peripheral venous plasma norepinephrine concentrations in heart failure patients. Using tritiated norepinephrine in patients with advanced heart failure, Davis et al. (51) and Hasking et al. (52) have shown that both increased norepinephrine secretion and decreased norepinephrine clearance contribute to the high venous plasma norepinephrine concentrations seen in these patients, suggesting that increased sympathetic activity is at least partially responsible for the elevated circulating plasma norepinephrine. We have demonstrated that the initial rise in plasma norepinephrine in heart failure is solely caused by increased norepinephrine secretion, providing evidence of increased sympathetic nervous system activity early in the course of cardiac failure (53). Moreover, plasma norepinephrine is increased in patients with asymptomatic left
ventricular dysfunction (i.e., before the onset of overt heart failure) (54). Finally, studies employing peroneal nerve microneurography to directly assess sympathetic nerve activity to muscle have confirmed the presence of increased sympathetic activity in heart failure patients (55). Significantly, the degree of activation of the sympathetic nervous system—as assessed by the peripheral venous plasma norepinephrine concentration—has been correlated with poor prognosis in heart failure (56).
ventricular dysfunction (i.e., before the onset of overt heart failure) (54). Finally, studies employing peroneal nerve microneurography to directly assess sympathetic nerve activity to muscle have confirmed the presence of increased sympathetic activity in heart failure patients (55). Significantly, the degree of activation of the sympathetic nervous system—as assessed by the peripheral venous plasma norepinephrine concentration—has been correlated with poor prognosis in heart failure (56).
Activation of Renal Nerves
Renal nerves also are activated in human heart failure (52). Enhanced renal sympathetic activity may contribute to the avid sodium and water retention in heart failure by promoting renal vasoconstriction, stimulation of the renin-angiotensin-aldosterone system, and direct effects on the proximal tubule epithelium. Indeed, intrarenal adrenergic blockade has been shown to cause a natriuresis in experimental heart failure (57). In addition, in rats, renal nerve stimulation has been demonstrated to produce approximately a 25% reduction in sodium excretion and urine volume (58). The diminished renal sodium excretion that accompanies renal nerve stimulation may be mediated by at least two mechanisms. As already discussed, studies performed in rats have demonstrated that norepinephrine-induced efferent arteriolar constriction alters peritubular hemodynamic forces in favor of increased tubular sodium reabsorption (47). In addition, renal nerves have been shown to exert a direct influence on sodium reabsorption in the proximal convoluted tubule (58).
Bello-Reuss et al. (58) have demonstrated this direct effect of renal nerve activation to enhance proximal tubular sodium reabsorption in whole-kidney and individual nephron studies in rats. In these animals, renal nerve stimulation produced an increase in the tubular fluid-to-plasma inulin concentration ratio in the late proximal tubule, an outcome of increased fractional sodium and water reabsorption in this segment of the nephron. Hence, increased renal nerve activity may promote sodium retention by a mechanism independent of changes in renal hemodynamics. On the other hand, sodium retention persists in dogs with denervated transplanted kidneys and chronic vena caval constriction. Moreover, renal denervation does not prevent ascites in dogs with chronic vena caval constriction (59). Thus, renal nerves probably contribute but do not fully account for the avid sodium retention of heart failure.
The Renin-Angiotensin-Aldosterone System in Cardiac Failure
The renin-angiotensin-aldosterone system also is activated in heart failure, as assessed by plasma renin activity (PRA) (60). Renin acts on angiotensinogen to produce angiotensin I, which is then converted by angiotensin-converting enzyme (ACE) to angiotensin II. In heart failure, the resultant increased plasma concentration of angiotensin II exerts important circulatory effects, including peripheral arterial and venous vascular constriction, renal vasoconstriction, and cardiac inotropism. Activation of angiotensin receptors on the proximal tubule epithelium directly stimulates the Na+/H+ exchanger 3 and thereby increases sodium reabsorption (61). Angiotensin II also acts to promote the secretion of the sodium-retaining hormone aldosterone by the adrenal cortex and in positive-feedback stimulation of the sympathetic nervous system. Activation of this hormonal system may promote sodium retention in the kidney via several mechanisms, as discussed next. Moreover, like adrenergic activation, stimulation of the renin-angiotensin-aldosterone system is associated with an unfavorable prognosis in heart failure (62).
Renal Effects of Increased Angiotensin II and Aldosterone
Angiotensin II may contribute to the sodium and water retention in heart failure through direct and indirect effects on proximal tubular sodium reabsorption and, as mentioned, by stimulating the release of aldosterone from the adrenal gland. Angiotensin II causes preferential renal efferent arteriolar constriction, resulting in decreased RBF and an increased filtration fraction. As with renal nerve stimulation, this results in increased peritubular capillary oncotic pressure and reduced peritubular capillary hydrostatic pressure, which favors the reabsorption of sodium and water in the proximal tubule (48). Moreover, as noted, angiotensin II has been shown to enhance sodium reabsorption in the proximal tubule (63). In a study of the rat proximal tubule, Liu and Cogan (63) demonstrated increased tubular sodium chloride reabsorption during the infusion of angiotensin II, whereas the angiotensin II receptor antagonist, saralasin, decreased proximal tubular sodium chloride reabsorption. Finally, in a report from Abassi et al. (64), the administration of the angiotensin II receptor antagonist, losartan, to decompensated sodium-retaining rats with heart failure secondary to AV fistulas produced a marked natriuresis. Although proximal tubular sodium handling was not examined in
this investigation, the observation that losartan restored renal responsiveness to ANP is consistent with a losartan-induced increase in the delivery of sodium to the distal tubular site of ANP action. The role of distal tubular sodium delivery in the renal sodium retention of heart failure is discussed later.
this investigation, the observation that losartan restored renal responsiveness to ANP is consistent with a losartan-induced increase in the delivery of sodium to the distal tubular site of ANP action. The role of distal tubular sodium delivery in the renal sodium retention of heart failure is discussed later.
Watkins et al. (65) studied a conscious dog model of heart failure in order to define more precisely the role of the renin-angiotensin-aldosterone axis in cardiac failure. Using either partial constriction of the pulmonary artery or thoracic inferior vena cava (TIVC), these workers acutely produced a low cardiac output state characterized by reduced blood pressure, increased PRA and aldosterone concentrations, and renal sodium retention. As plasma volume and body weight increased over several days, the aforementioned variables all returned toward control levels. During the initial hyperreninemic period, a single injection of an ACE inhibitor significantly lowered blood pressure. Also, chronic administration of the converting enzyme inhibitor prevented a rise in aldosterone and prevented 30% of the sodium retention and subsequent volume expansion. These studies lend support to the hypothesis that aldosterone is an important factor in the pathogenesis of cardiac edema and suggest that angiotensin II plays an important physiologic role in heart failure by supporting blood pressure because of its vasoconstrictor effect and maintaining blood volume secondary to the sodium-retaining effects of angiotensin II and aldosterone. It likewise becomes clear that, depending on the status of cardiac decompensation and plasma volume, the patient with heart failure may have a high or normal PRA and aldosterone level. This may explain some of the controversy that existed regarding the levels of these hormones in patients with heart failure.
A further role for renin-angiotensin-aldosterone system activation in the sodium retention of human heart failure is supported by the finding that urinary sodium excretion inversely correlates with PRA and urinary aldosterone excretion in heart failure patients (66). However, the administration of an ACE inhibitor (ACEI) during heart failure does not consistently increase urinary sodium excretion in spite of a consistent fall in plasma aldosterone concentration (67). The simultaneous fall in blood pressure caused by decreased circulating concentrations of angiotensin II, however, may activate hemodynamic and neurohormonal mechanisms that could obscure the natriuretic response to lowered angiotensin II and aldosterone concentrations. Support for this hypothesis comes from the study performed by Hensen et al. (68). We examined the effect of the specific aldosterone antagonist, spironolactone, on urinary sodium excretion in patients with heart failure who were withdrawn from all medications before study. Avid sodium retention occurred in all patients throughout the period before aldosterone antagonism. During therapy with spironolactone (200 mg b.i.d.), all heart failure patients exhibited a significant increase in urinary sodium excretion and reversal of the positive sodium balance (Fig. 2-3). Moreover, the urinary sodium-to-potassium concentration ratio significantly increased during spironolactone administration, consistent with a decrease in aldosterone action in the distal nephron. Of note, PRA and norepinephrine increased and ANP decreased during the administration of spironolactone. Thus, this investigation demonstrates reversal of the sodium retention of heart failure with the administration of an aldosterone antagonist, despite further activation of various antinatriuretic influences, including stimulation of the renin-angiotensin and sympathetic nervous systems, and supports a role for aldosterone in the renal sodium retention. A prospective trial, Randomized Aldactone Evaluation Study (RALES), has shown improved survival of heart failure patients receiving 25 mg/day of spironolactone (a competitive inhibitor of aldosterone) (69). This effect of spironolactone in the RALES investigation was found to be independent of any change in sodium balance. An effect of spironolactone to block the effect of aldosterone-mediated cardiac fibrosis has been suggested as the mediator of this improved survival response. Natriuretic doses of spironolactone rarely have been used in patients with heart failure. One study was performed in congestive heart failure (CHF) patients receiving low-dose ACEIs who had diuretic resistance. These patients demonstrated a natriuresis with a daily dose of 100 mg of spironolactone (70). The Eplerenone Post-AMI Heart Failure Efficacy and Survival Study (EPHESUS) demonstrated reduced mortality after acute myocardial infarction (71).
In the Acute Decompensated Heart Failure Registry (ADHERE), decompensated CHF patients resistant to oral diuretics were hospitalized and 90% were given intravenous diuretics. Forty-two percent of these patients were discharged with unresolved symptoms, 50% lost ≤5 lb, and 20% actually gained weight. Approximately 25% to 30% of CHF patients become resistant to diuretics and, as discussed later, secondary hyperaldosteronism is an important factor in such diuretic resistance (72).
Nevertheless, natriuretic doses of mineralocorticoid antagonists may not be part of the therapeutic armamentarium for heart failure, primarily because of the fear of hyperkalemia (73). Many of these CHF patients are receiving ACEIs or angiotensin receptor blockers (ARBs) and/or β-blockers, which predispose to hyperkalemia. Whether low-potassium diet, sodium polystyrene sulfonate (Kayexalate), and potassium-losing diuretics may avoid the occurrence of hyperkalemia during use of natriuretic doses of mineralocorticoid antagonists has not been studied. Given the challenge of treating cardiac patients with acute decompensation, as noted in the
ADHERE registry, inhibiting the secondary hyperaldosteronism in sodium-retaining CHF patients who are diuretic resistant needs to be undertaken. Isotonic removal of sodium in CHF patients with ultrafiltration is another therapeutic approach. Fluid removal in CHF patients with ultrafiltration or diuretics can improve cardiac and renal function in addition to treating pulmonary congestion and edema. The mechanisms are shown in Figure 2-4 (74).
ADHERE registry, inhibiting the secondary hyperaldosteronism in sodium-retaining CHF patients who are diuretic resistant needs to be undertaken. Isotonic removal of sodium in CHF patients with ultrafiltration is another therapeutic approach. Fluid removal in CHF patients with ultrafiltration or diuretics can improve cardiac and renal function in addition to treating pulmonary congestion and edema. The mechanisms are shown in Figure 2-4 (74).
The Nonosmotic Release of Arginine Vasopressin in Cardiac Failure
Plasma AVP is often elevated in patients with CHF and correlates in general with the clinical and hemodynamic severity of disease and the serum sodium level. Using a sensitive radioimmunoassay for AVP, Szatalowicz et al. (75) initially showed that plasma AVP was detectable in 30 of 37 patients with cardiac failure and hyponatremia. It was concluded that the nonosmotic AVP release in these patients was the result of baroreceptor stimulation secondary to diminished cardiac output because these patients had sufficient hyponatremia and hypo-osmolality, which would normally suppress maximally the osmotic release of AVP. Riegger et al. (76) also have reported that several patients with heart failure had inappropriately high plasma AVP levels. Cardiac output increased and plasma AVP levels normalized when two of these patients were treated with hemofiltration to remove excess body fluid. Other studies have also incriminated AVP in hyponatremic CHF patients (77, 78). Taken together, these observations demonstrate enhanced nonosmotic AVP release in response to a decrease in cardiac output (i.e., arterial underfilling).
Renal Effects of Arginine Vasopressin
AVP, via stimulation of its renal or V2 receptor subtype, enhances water reabsorption in the distal nephron, namely, the cortical and medullary collecting ducts. The evidence supporting a role for AVP in the water retention of heart failure comes from studies using selective peptide and nonpeptide antagonists of the V2 receptor of AVP in several animal models of cardiac failure. For example, Ishikawa et al. (79) have assessed the antidiuretic effect of plasma AVP in a low-output model of cardiac failure secondary to vena caval constriction in rats. Plasma AVP concentrations were increased in these animals, and an antagonist of the antidiuretic effect of AVP reversed the defect in water excretion. An orally active nonpeptide V2 receptor AVP antagonist, OPC-31260, was originally described in 1992 (80). The intravenous administration of OPC-31260 during a dose-ranging study in normal human subjects was shown to increase urine output to a similar extent as 20 mg of furosemide given intravenously (81). Virtually simultaneous publications by Xu et al. (82) from our laboratory and Nielsen et al. (83) demonstrated the upregulation of aquaporin 2 (AQP2) water channels in
coronary-ligated rats with CHF. The latter group also demonstrated that AQP1 and AQP3 were not upregulated in this CHF model and that increased trafficking of the AQP2 to the apical membrane occurred. Our group further showed that a V2 vasopressin antagonist reversed the upregulation of the AQP2 protein in the renal cortex and medulla of the CHF rats (81). This effect of the nonosmotic release of AVP to cause water retention in cardiac failure recently has been associated with increased transcription of messenger RNA (mRNA) for the AVP preprohormone in the rat hypothalamus (84).
coronary-ligated rats with CHF. The latter group also demonstrated that AQP1 and AQP3 were not upregulated in this CHF model and that increased trafficking of the AQP2 to the apical membrane occurred. Our group further showed that a V2 vasopressin antagonist reversed the upregulation of the AQP2 protein in the renal cortex and medulla of the CHF rats (81). This effect of the nonosmotic release of AVP to cause water retention in cardiac failure recently has been associated with increased transcription of messenger RNA (mRNA) for the AVP preprohormone in the rat hypothalamus (84).
In a study by Bichet et al. (85), the effect of the ACEI captopril and the α1-adrenergic blocker prazosin to reverse the abnormality in water retention in patients with class III and IV heart failure was examined. The resultant cardiac afterload reduction and increased cardiac output with either agent were associated with improved water excretion and significant suppression of AVP in response to an acute water load. A role of angiotensin II in modulating the effect of AVP in heart failure was unlikely because captopril and prazosin had divergent effects on the renin-angiotensin system; yet their effects to suppress plasma AVP and improve water excretion were comparable. In this regard, it is important to note that in this study by Bichet et al. (85), the average decrease in mean arterial pressure was 5 mm Hg, a decrement that is less than the 7% to 10% necessary to activate the nonosmotic release of AVP (86). Thus, these results are compatible with the suggestion that a decrease in stroke volume and cardiac output, rather than a fall in mean arterial pressure, may sometimes be the primary stimulus for the nonosmotic release of AVP in low-output cardiac failure. The association of improved cardiac output and water excretion during afterload reduction is compatible with unloading of high-pressure baroreceptors leading to increased AVP release.
The most recent advance relative to the nonosmotic release of AVP in CHF is the FDA approval of vasopressin receptor antagonists for clinical use in the United States. Conivaptan, a combined V1 and V2 receptor antagonist, has been approved for treatment of hyponatremia in cardiac failure. This antagonist can be used inhospital by intravenous administration for 4 days. The potential effect of the combined V1 and V2 antagonist properties in heart failure is shown in Figure 2-5 (74). Recently, the first orally active V2 receptor antagonist, tolvaptan, has been approved for use in cardiac failure, cirrhosis, and the syndrome of inappropriate antidiuretic hormone (SIADH) (87). In association with the increase in plasma sodium concentration in hyponatremic CHF patients, the self-reported SF12 demonstrated a significant improvement in mental status in these patients. There are other V2 receptor antagonists in phase 3 trials. Taken together, these agents are known as aquaretics to emphasize that the resultant increase in solute-free water excretion occurs in the absence of a change in electrolyte excretion. This is the major difference with diuretics that increase urinary sodium chloride and other electrolyte excretion. These aquaretic agents can correct plasma sodium concentration in the absence of fluid restriction. In chronic hyponatremia, the correction of plasma sodium concentration with an aquaretic should not exceed 8 mmol over 8 hours or 10 to 12 mmol over 24 hours in order to avoid osmotic demyelination.
Altered renal hemodynamics may contribute to water retention in heart failure in addition to persistent AVP secretion. Decreased RBF and increased filtration fraction would be expected to increase proximal reabsorption of sodium and water, thereby diminishing fluid delivery to distal diluting segments. Increasing distal fluid delivery by administration of furosemide has improved the diluting ability of patients with heart failure (88).
In summary, activation of the sympathetic nervous system, the renin-angiotensin-aldosterone system, and the nonosmotic release of AVP by exerting direct (tubular) and indirect (hemodynamic) effects on the kidneys are implicated in the renal sodium and water retention of heart failure. These neuroendocrine mechanisms appear to be activated in response to arterial underfilling and suppressed by maneuvers that restore the integrity of the arterial circulation toward normal. In addition, the effects of these neurohormonal vasoconstrictor systems may be counterbalanced by endogenous vasodilatory and natriuretic hormones.
Natriuretic Peptides in Cardiac Failure
The natriuretic peptides, including ANP and brain natriuretic peptide (BNP), circulate at increased concentrations in patients with heart failure (89, 90). These peptide hormones possess natriuretic, vasorelaxant, and renin-, aldosterone-, and sympatho-inhibiting properties (91). Both ANP and BNP appear to be released primarily from the heart in response to increased atrial or ventricular end-diastolic or transmural pressures. We demonstrated that increased ANP production rather than decreased metabolic clearance was the major factor contributing to the elevated plasma ANP concentrations in a study of ANP kinetics in patients with cardiac failure (92). This finding is consistent with the observed increase in expression of both ANP and BNP mRNA in the cardiac ventricles of humans and animals with heart failure (93, 94). BNP has been shown to reduce pulmonary capillary wedge pressure (PCWP) and increase cardiac index in acute CHF (95). In a
coronary ligation model of heart failure in rats, the infusion of a monoclonal antibody shown to specifically block endogenous ANP in vivo caused a significant rise in right atrial pressure, left ventricular end-diastolic pressure, and systemic vascular resistance (96). Thus, natriuretic peptides appear to attenuate to some degree the arterial and venous vasoconstriction of heart failure.
coronary ligation model of heart failure in rats, the infusion of a monoclonal antibody shown to specifically block endogenous ANP in vivo caused a significant rise in right atrial pressure, left ventricular end-diastolic pressure, and systemic vascular resistance (96). Thus, natriuretic peptides appear to attenuate to some degree the arterial and venous vasoconstriction of heart failure.
Renal Effects of the Natriuretic Peptides
In normal humans, ANP and BNP increase GFR and urinary sodium excretion with no change or only a slight fall in RBF (97). These changes in renal hemodynamics are likely mediated by afferent arteriolar vasodilation with constriction of the efferent arterioles. However, in addition to increasing GFR and filtered sodium load as a mechanism of their natriuretic effect, ANP and BNP are specific inhibitors of sodium reabsorption in the collecting tubule (98). An important role for endogenous ANP in the renal sodium balance of heart failure has been demonstrated by Lee et al. (99). Similar decreases in cardiac output were induced in two groups of dogs by constriction of the TIVC or acute rapid ventricular pacing. Sodium retention paralleled the activation of the renin-angiotensin-aldosterone system in the TIVC constriction group. Atrial pressures and plasma ANP were not increased in this group of dogs. In comparison, the ventricular pacing group did not experience sodium retention or activation of the renin-angiotensin-aldosterone system. This group had similar reductions in cardiac output and arterial pressure as the TIVC constriction group but, unlike the TIVC constriction group, had increased atrial pressures and circulating endogenous ANP levels. In a third group, exogenous ANP was administered to TIVC constriction dogs to increase plasma ANP levels to those observed in the pacing model. The ANP infusion prevented the sodium retention and activation of the renin-angiotensin-aldosterone system.
Unfortunately, the administration of synthetic ANP to patients with low-output heart failure results in a much smaller increase in renal sodium excretion and less significant changes in renal hemodynamics compared with normal subjects (100). Like ANP, the natriuretic effect of BNP is blunted in rats with high-output heart failure produced by AV fistulas (101). In a trial of BNP, 127 patients with a PCWP of 18 mm Hg or higher and a cardiac index of 2.7 L/min/m2 of body surface area or less were randomly assigned to double-blind treatment with placebo or BNP (nesiritide) infused at a rate of 0.015 or 0.030 µg/kg of body weight per minute for 6 hours (95). BNP significantly decreased PCWP and resulted in improvements in global clinical status in most patients (i.e., reduced dyspnea and fatigue). The most common side effect was dose-related hypotension, which was usually asymptomatic. Therefore, intravenous nesiritide may be useful for the short-term treatment of patients hospitalized with decompensated CHF (95). A recent retrospective report, however, demonstrated an increase in serum creatinine and mortality in heart failure patients receiving BNP (102).
The possible mechanism of the relative renal resistance to natriuretic peptides in heart failure are the following:
Downregulation of renal ANP receptors
Secretion of inactive immunoreactive ANP
Enhanced renal neutral endopeptidase activity limiting the delivery of ANP to receptor sites
Hyperaldosteronism causing increased sodium reabsorption in the distal renal tubule
Diminished delivery of sodium to the distal renal tubule site of ANP action
A strong positive correlation between plasma ANP and urinary cGMP (the second messenger for the natriuretic effect of ANP and BNP in vivo) has been shown in sodium-retaining patients with heart failure (103). This observation supports the active biologic responsiveness of renal ANP receptors in heart failure and thus suggests that diminished distal tubular sodium delivery may explain the natriuretic peptide resistance observed in patients with cardiac failure. In cirrhosis, another edematous disorder associated with renal ANP resistance, increased distal tubular sodium delivery with mannitol has been shown to reverse the ANP resistance (104). Moreover, in heart failure, the administration of an angiotensin II receptor antagonist or furosemide, which is expected to increase distal tubular sodium delivery, also improves the renal response to ANP (64, 105). Finally, studies in rats with experimental heart failure have demonstrated that renal denervation reverses the ANP resistance (106), an effect likely mediated by increased distal tubular sodium delivery. In Figure 2-6, the proposed role of diminished distal tubular sodium delivery in natriuretic peptide resistance and impaired aldosterone escape in states of arterial underfilling is shown.
Renal Prostaglandins in Cardiac Failure
Renal prostaglandins do not regulate renal sodium excretion or renal hemodynamics to any significant degree in normal subjects and intact animals. However, prostaglandin activity is increased in patients with heart failure and has been shown to correlate with the severity of disease as assessed by the degree of hyponatremia (107). Moreover, it has been well documented that the administration of a cyclooxygenase inhibitor in heart failure patients may result in acute reversible renal failure, an effect proposed to result from inhibition of renal prostaglandins (108). An investigation in patients with moderate heart failure and a normal sodium intake demonstrated that the administration of acetylsalicylic acid in doses that decrease the synthesis of renal prostaglandin E2 results in a significant reduction in urinary sodium excretion (109). These observations support a role for prostaglandins in attenuating the renal vasoconstriction and sodium retention in patients with heart failure.
PATHOGENESIS OF SODIUM AND WATER RETENTION IN CIRRHOSIS
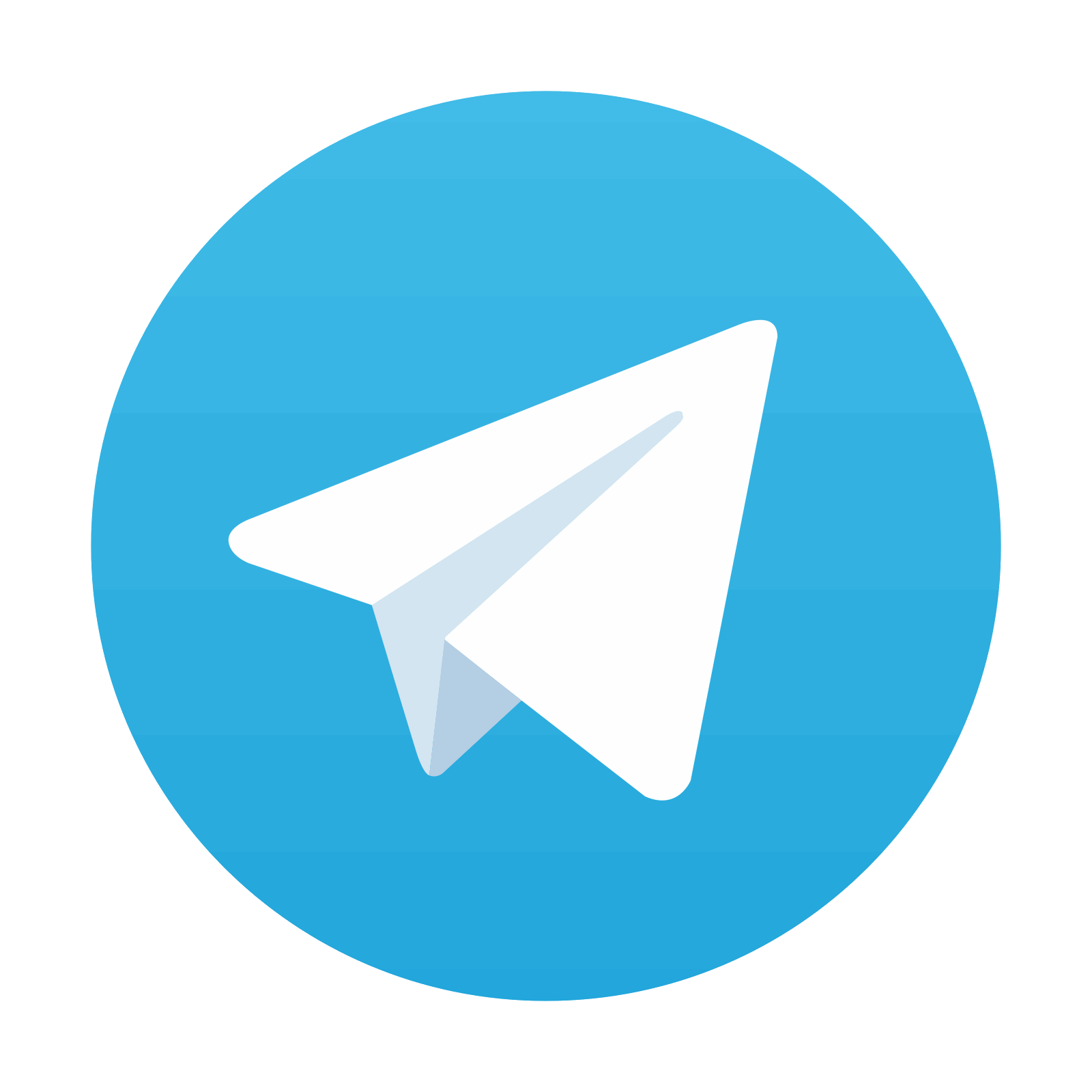
Stay updated, free articles. Join our Telegram channel

Full access? Get Clinical Tree
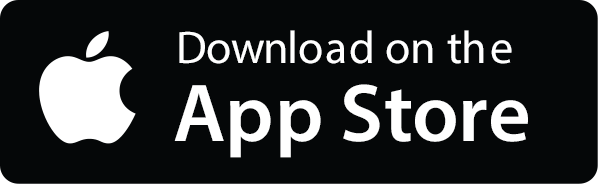
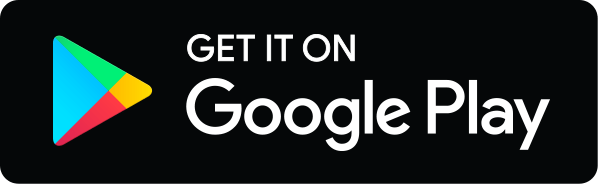