Renal Physiology and Pathophysiology in Pregnancy
Normal pregnancy is characterized by alterations in renal and cardiovascular functions that accommodate the hemodynamic and metabolic demands of the growing fetus. Glomerular filtration rate (GFR) and renal blood flow increase in the first trimester, coincident with the dramatic increases in pregnancy-related hormones. Generalized vasodilation is present, and in normal gestation, maternal blood pressure (BP) decreases and cardiac output (CO) increases. These physiologic adjustments are necessary for normal fetal growth and well-being. Reduced GFR and hypertension are risk factors for poor pregnancy outcomes, including fetal growth restriction, preeclampsia, and fetal death. In this chapter, we will discuss the fundamentals of renal physiologic principles in pregnancy, the unique considerations relevant to pregnancy in women with kidney disease, and the hypertensive disorders in pregnancy.
Renal Physiology in Pregnancy
MATERNAL CIRCULATION AND BLOOD VOLUME REGULATION IN PREGNANCY
During normal pregnancy, the maternal circulation is characterized by vasodilation, which starts in the sixth week of gestation and leads to a decrease in systemic vascular resistance (SVR) (1,2). Vasodilation has been attributed to an increase in progesterone, prostacyclin, vascular endothelial growth factor (VEGF) (3), nitric oxide (4–9), angiotensin 1–7 (10,11), and relaxin (12–20). To date, the exact mechanisms leading to systemic vasodilation and the degree to which each of these factors contributes have not been completely defined.
In order to maintain adequate blood volume despite a decreased SVR, maternal total body water is increased by 6 to 8 L, with an increase of 4 to 6 L in the extracellular compartment (21). Renal plasma flow (RPF) increases early in pregnancy also by the sixth week of gestation. By midpregnancy, the plasma volume is increased by 50% (21,22), leading to a 40% to 65% rise in RPF (as measured by para-aminohippurate clearance) (23). RPF peaks at 80% above prepregnancy levels during the second trimester (21,22) and declines in the third trimester to 60% greater than prepregnancy values (23).
There is also a net reabsorption of 500 to 950 mEq of sodium during pregnancy (21,22), which permits maternal plasma volume expansion. Sodium homeostasis is discussed in further detail later in this chapter. Pregnancy-associated volume expansion resolves rapidly by 2 weeks postpartum (24).
Plasma volume expansion in pregnancy is well tolerated, although peripheral edema is not uncommon. It is typically an adaptive phenomenon rather than a pathologic state of volume overload and does not adversely affect cardiovascular hemodynamics. During pregnancy, SVR drops, whereas CO, stroke volume, and heart rate are all increased (25–29). CO increases by 11% during the first trimester, remains stable during the second trimester, and increases again to 17% after 28 weeks of gestation (25), with the highest CO reported being 50% greater than nonpregnancy values (26,28,29). The timing of the CO peak and the magnitude of the increase in the third trimester have diverged across studies (29,30) potentially related to differences in methodologies where echocardiography and impedance have both been performed. Postpartum, CO returns to prepregnancy levels in approximately 2 weeks (31). Measurements of CO were done by echocardiography in 74% of studies and by impedance for 18% (29).
BLOOD PRESSURE REGULATION IN NORMAL PREGNANCY
During pregnancy, generalized vasodilation leads to a decrease in mean arterial blood pressure (MAP) of approximately 10 mm Hg (32), starting early in the first trimester, with a nadir between 12 and 28 weeks of gestation (2,32,33). In late pregnancy, BP gradually starts to increase again back to prepregnancy levels. Normal BP in pregnancy is lower compared to nonpregnancy; however, optimum BP has not been defined. Women are considered to have hypertension if BP is greater than or equal to 140/90 mm Hg. However, this threshold does not adequately address what is the optimum BP for most women.
All components of the renin–angiotensin–aldosterone system (RAAS) are increased during pregnancy. Plasma renin activity (PRA) rises by 6 weeks of gestation (2) and may quadruple by midpregnancy (32). The early gestational rise in PRA is likely a consequence of vasodilation and lower BP, and is accompanied by a rise in aldosterone (2). Blockade of the RAAS with a single dose of captopril, administered to first and second trimester pregnant women, results in an augmented drop in BP and rise in renin compared to nonpregnant women (34), suggesting that the stimulated RAAS in pregnancy is a physiologically appropriate response to lower BP. Chapman et al. reported that in the third trimester, aldosterone continues to rise, whereas PRA remains constant (2). We and others have found that both serum and urine aldosterone concentration continuously increase as pregnancy progresses (35–37). Our results also suggest that PRA and aldosterone levels are highly correlated throughout gestation (unpublished results). The recent studies of Gennari-Moser et al. (38) demonstrate that elevated aldosterone levels in pregnancy help maintain BP and are also consistent with the notion that the stimulated RAAS in pregnancy is a physiologic response to the hormonally mediated changes in BP and vasodilation.
Several investigators have observed that the maternal vasculature is significantly less responsive to angiotensin II, norepinephrine, and vasopressin (as compared to nonpregnant women) (39–46). The basis for this observation is not known; it may be a consequence of “downregulation” of vascular receptors resulting from higher levels of circulating angiotensin II occupying and internalizing these receptors, or it may reflect the fact that BP is lower owing to vasodilatory pregnancy-related hormones that lead to compensatory increases in angiotensin II, norepinephrine, and other pressors that do not generate as significant a BP response.
Gestational resistance to the stimulated RAAS is relative, and the RAAS remains an important regulator of salt balance and BP in pregnancy (47). The RAAS demonstrates typical endocrine regulation, and aldosterone increases after standing up in pregnancy (48,49) and decreases with salt loading (35,49). As mentioned, in women admitted for pregnancy termination, the administration of an angiotensin-converting enzyme inhibitor leads to a marked hypotensive response and increase in PRA (34). Salt loading in pregnant women results in similar changes in urine Na levels as seen in nonpregnant women (38).
The interaction of estrogen, progesterone, and the RAAS, and their role in BP regulation in pregnancy is particularly intriguing. Elevations of estrogen are in part responsible for the elevations in angiotensinogen in pregnancy and lead to a doubling of PRA (32,50,51). Progesterone is a weak mineralocorticoid receptor antagonist (52,53), which leads to aldosterone resistance by competitively binding to the mineralocorticoid receptor (54,55). Progesterone may also decrease BP by other natriuretic mechanisms (56), including impairment of proximal Na reabsorption (52).
The sympathetic nervous system has been reported to be overly active during the first few weeks of pregnancy (57) as well as during the third trimester (58). Some investigations suggest that sympathetic nervous system overactivation is even more pronounced in hypertensive pregnancies; however, the impact on sustained hypertension is unclear (58–61).
RENAL ANATOMY DURING NORMAL PREGNANCY
There is a 30% increase in kidney size during pregnancy (62,63), most likely a result of the increased renal blood flow and resulting vascular and interstitial expansion. Relaxation and expansion of the renal calyces is also reported as a physiologic finding (62,64–68). This has been attributed to compression of the ureters between the iliopsoas muscle and the gravid uterus (69–71). However, the appearance of dilatation of the urinary tract on renal ultrasound may also be a consequence of hydronephrosis, especially in the third trimester (72,73). These anatomic alterations may contribute to the development of bacteriuria and pyelonephritis in pregnancy.
RENAL FUNCTION IN PREGNANCY
Glomerular Hemodynamics in Pregnancy
In the context of generalized vasodilation and volume expansion, it is not surprising that the GFR is increased during pregnancy (8,23,24,48,74–80) (Fig. 13-1). Inulin-measured GFR and creatinine clearance increase by 50% early in the first trimester (23,81). By the third trimester, increases of up to 75% above nonpregnant levels are reported (2). As previously mentioned, RPF rises even more (by up to 80%) in early pregnancy (23,74–76) and returns to prepregnancy levels by the third trimester (2,23). RPF remains stable and even slightly lower than prepregnancy levels in the postpartum period (82). The filtration fraction, defined as the ratio of GFR/RPF, is therefore lowered earlier in pregnancy (from 6 to 36 weeks of gestation) (2) and subsequently increases (2,75,82). Although volume expansion resolves by 2 weeks after delivery, the GFR remains 20% above prepregnancy levels (24) and only returns to prepregnancy levels a month postpartum (83) (Fig. 13-2).
It is likely that there are mechanisms in addition to vasodilation and increased RPF underlying the increase in GFR during pregnancy. Older data suggest that progesterone may contribute to the gestational increase in GFR (55). Relaxin has been associated with gestational vasodilation but is not likely to be the primary mediator of the renal hemodynamic changes and GFR increase during human pregnancy (18,84). Other factors believed to be contributing to the gestational rise in GFR include a decrease in vascular and glomerular oncotic pressure, and increased glomerular basement membrane permeability noted in pregnancy (77,85,86) and also in the postpartum period (24,82). A potential role for estrogen in the increased GFR in pregnancy has been postulated, based in part on gender differences in chronic kidney disease (CKD) progression demonstrating better outcomes in women compared to men, and this is an area worthy of further study (87).
Consistent with increases in measured GFR, creatinine clearance also increases progressively during pregnancy (81). The 24-hour urinary creatinine excretion, however, remains unchanged (2), leading to an overall decrease in serum creatinine during pregnancy from an average of 0.83 mg/dL (73 mmol/L) prepregnancy to 0.74 mg/dL (65 mmol/L) in the first trimester, 0.58 mg/dL (51 mmol/L) in the second trimester, and 0.53 mg/dL (46 mmol/L) in the third trimester (88). This decrease in serum creatinine is not solely attributable to an increase in GFR but may also be influenced by an increase in maternal nonmuscle body weight. Creatinine-based formulas such as the Modification of Diet in Renal Disease (MDRD) (89–91), Cockroft–Gault (91,92), or Chronic Kidney Disease Epidemiology Collaboration (CKD-EPI) (93) are frequently used to estimate GFR but have not been validated in pregnancy and are not thought to be accurate calculations of GFR in this setting. Cystatin C-based formulas to estimate GFR have also not been validated in pregnancy (91,94). Consequently, the best estimator of GFR during pregnancy remains creatinine clearance (determined by a 24-hour urine collection).
Figure 13–1 Renal hemodynamic changes in pregnancy. Black arrows depict changes in comparison with the nonpregnant state. CO, cardiac output; TPR, total peripheral resistance; RPF, renal plasma flow; PGC, glomerular oncotic pressure; PGC, glomerular capillary pressure; Kf, transcapillary ultrafiltration coefficient; GFR, glomerular filtration rate. (From Hussein W, Lafayette RA. Renal function in normal and disordered pregnancy. Curr Opin Nephrol Hypertens. 2014;23(1):46–53, with permission from Wolters Kluwer Health, Inc.)
Figure 13–2 Longitudinal changes in renal hemodynamic parameters during pregnancy. The percentage change in GFR, RPF, and FF are expressed at different time points in gestation. GFR was measured by inulin or iothalamate and RPF by p-aminohippurate clearance methodology, respectively, at different time points during gestation. FF, filtration fraction; GFR, glomerular filtration rate; RPF, renal perfusion flow. (Republished with permission of American Society of Nephrology from Odutayo A, Hladunewich M. Obstetric nephrology: renal hemodynamic and metabolic physiology in normal pregnancy. Clin J Am Soc Nephrol. 2012;7(12):2073–2080; permission conveyed through Copyright Clearance Center, Inc.)
TUBULAR FUNCTION IN PREGNANCY
During pregnancy, GFR and RPF increases lead to increased demands on tubular transport. Tubular reabsorption may fail to match these rising demands, leading to increased excretion of protein, glucose, and amino acids (95).
Proteinuria and Albuminuria in Normal Pregnancy
Normal pregnancy is associated with an increase in daily urinary protein excretion (96–99). Proteinuria is normally below 150 mg/day; it increases by approximately 100 mg/day during pregnancy (97) and may reach 260 mg/day in normal pregnant women (99). Increased proteinuria is more common in twin compared to singleton gestations (100). The threshold for abnormally elevated proteinuria is considered to be greater than 300 mg/day during pregnancy (101).
During pregnancy, the total daily albuminuria is not significantly increased (97,102–106) and therefore does not account for the physiologic proteinuria of normal pregnancy. A few studies have reported that the urinary albumin to creatinine ratio is elevated in pregnancy (107) and increases as gestation progresses (108,109) and in the peripartum period (102). Despite these observations, the urinary albumin to creatinine ratio does not increase enough to account for the important increase in proteinuria (107) and does not reflect an overall increase in the 24-hour excretion of albumin (106).
The preponderance of nonalbumin proteinuria therefore points to a tubular rather than glomerular origin of proteinuria. Indeed, tubular proteins such as retinol-binding protein (97,104,110), N-acetyl-β-glucosaminidase (98,104,107,111–114), β2-microglobulin (104,107,115), α1-microglobulin (104), transferrin (98,115), alanine aminopeptidase (98,114), and Clara cell protein (104) are elevated in the urine of healthy pregnant women. Whether this is a manifestation of tubular dysfunction and impaired tubular protein reabsorption is not clear.
Uric Acid Excretion in Normal Pregnancy
Increased GFR and decreased tubular reabsorption during pregnancy lead to an increase in urinary excretion of uric acid and generally lower levels in plasma and serum (56,95). As pregnancy progresses (116) beyond 20 weeks (117), uric acid excretion decreases and plasma levels of uric acid may increase again to prepregnancy levels in the third trimester (116,118).
Glucose and Amino Acid Excretion in Normal Pregnancy
Glucosuria may be present in normal pregnancies, and older studies suggest that it is because of decreased proximal tubular reabsorption (119–121). Proximal tubular glucose reabsorption may be decreased in pregnancy owing to volume expansion, leading to decreased sodium reabsorption and therefore less glucose reabsorption (122,123). Increased GFR and hyperfiltration, as well as glucose loading, may further saturate the ability of the tubules to reabsorb glucose and induce glucosuria (122,124) independently of glycemia (125). Therefore, mild glucosuria is not a reliable screening test for diabetes in pregnancy (126,127).
Amino acids are also reabsorbed by the proximal tubule and are excreted in the urine in larger amounts during pregnancy (125). Different amino acids have distinct patterns of variation throughout gestation (128). Water-soluble vitamins, including nicotinic acid (129), vitamin C (125), and folate (125,130,131), are also found in larger amounts in the urine of pregnant women.
WATER HANDLING IN PREGNANCY
During pregnancy, plasma osmolality is decreased by 5 to 10 mOsm/kg early in the first trimester with a decrease in serum sodium by 5 mEq/L. Pregnancy is considered to be a condition characterized by a reset osmostat, because osmolality is maintained at this level with appropriate osmoregulation in response to water excess and deprivation (132–136). There is also evidence of nonosmotic release of antidiuretic hormone (ADH) in the setting of vasodilation and lower BP (137).
Measurement of serum ADH or vasopressin is technically difficult given that circulating levels are typically low and below the level of detection of the assay; therefore, investigators have recently started to measure copeptin as a surrogate for ADH. Copeptin is co-secreted with ADH and has a longer half-life, and the assay allows for detections of relatively low circulating levels, which renders this peptide easier to measure reliably (138,139). During pregnancy, copeptin levels rise with gestational age (139); this occurs to a larger extent if preeclampsia develops (138,139).
Leucine aminopeptidase (140), also referred to as placental vasopressinase (141), has been described as an important mitigator of physiologic hyponatremia of pregnancy. In certain instances, in the third trimester, placental vasopressinases may cause ADH resistance that is manifested as gestational diabetes insipidus. Because these enzymes act at the N-terminal of the native ADH molecule, administering dDAVP (a synthetic agonist of ADH with a different N-terminal) may correct gestational diabetes insipidus (142).
Potential mechanisms for a reset osmostat in pregnancy include elevated levels of β-human chorionic gonadotropin (HCG) (134,143) and relaxin (144,145). It has been hypothesized (136) that β-HCG induces relaxin release from the placenta, which then causes the reset osmostat of pregnancy; however, this has not been reproduced experimentally.
ELECTROLYTE HANDLING IN PREGNANCY
Calcium and Vitamin D Balance
Both total serum calcium (Ca) and albumin decrease in pregnancy; however, ionized Ca remains unchanged (146,147). An increase in Ca clearance and urinary Ca excretion has been reported, but the fractional excretion of ionized Ca is relatively stable in pregnancy (147). The increase in urinary total Ca excretion may be attributed to an increase in GFR (147) and to an increased dietary Ca intake that is encouraged by many obstetricians in order to maintain adequate calcium balance for fetal bone mineralization (148). Also, 25-hydroxylation of vitamin D is increased during normal pregnancy (149) owing to placental conversion of vitamin D3, resulting in levels of 1,25-dihydroxyvitamin D (1,25-D) that are two to three times the nonpregnant levels and result in increased intestinal calcium absorption (reviewed by Hojo and August (150)). Calcitonin increases (151) and intact PTH (parathyroid hormone) decreases in order to counteract bone resorption (152).
Calcium metabolism is dysregulated in women with preeclampsia. Decreased urinary excretion of calcium has been reported both prior to and at the time of diagnosis of preeclampsia (153–157). The mechanism of hypocalciuria may be in part related to the mild decreases in GFR; however, changes in both 1,25-D and PTH may also be responsible (153,158). 1,25-D is lower in women with preeclampsia (155,159–161), and women with lower levels of 25-hydroxyvitamin D (less than 30 nmol/L as opposed to 50 or above, at 14 weeks) (162) and 1,25-D (159) are at a higher risk of preeclampsia, preterm birth, and small for gestational age (SGA) infants (163). Several clinical trials have suggested a benefit of calcium supplementation in prevention of preeclampsia (164). Although not extensively studied, there is some suggestion that vitamin D supplementation may have beneficial effects with respect to prevention of hypertension in pregnancy (149,165). Despite hypocalciuria and decreased levels of active vitamin D, ionized calcium is maintained at a normal or mildly lower level in preeclampsia. Intact PTH is reported to be increased in preeclampsia in some, but not all, studies (155,159,160).
Sodium and Potassium Homeostasis
Total body sodium (Na) and total body potassium (K) both increase during pregnancy in order to accommodate the developing fetus and placenta. Na accumulation is estimated to be 900 to 1,000 mEq (approximately 2–6 mEq/day). Total potassium accumulation is approximately 320 mEq (86). Total body electrolyte stores are not necessarily reflected by serum electrolyte concentrations because of the increased plasma volume. Normal ranges for electrolytes have not been clearly established in pregnancy despite the observation that serum/plasma Na and K are often lower in the first two trimesters of pregnancy (166–168), owing to both hemodilution and hypoosmolality of pregnancy.
Renal Na and K excretion in pregnancy have not been studied extensively. Urinary Na excretion (UNa) decreases with gestational age (37,169). However, the literature is not unanimous about the patterns of urinary K excretion (UK). Tamas et al. report an increase in UK (37), whereas Ehrlich and Lindheimer report that on a controlled diet, UK is stable throughout pregnancy (170). In both cases, as the pregnancy proceeds, the ratio of UNa to UK drops (37,170), and the administration of a mineralocorticoid affects UNa but not UK (170). This uncoupling of Na and K excretion furthers the suspicion that Na and K excretions are differentially regulated by aldosterone in normal pregnancy or that other nonmineralocorticoid pathways play a significant role. Na retention occurs in synchrony with increasing aldosterone (167). A study by Tamas et al. failed to demonstrate a correlation between urine aldosterone and UNa (37).
The role of progesterone in the regulation of UNa, UK, and aldosterone is of considerable interest, but has not been extensively studied (86,170). Progesterone antagonizes aldosterone’s binding to the mineralocorticoid receptor, thereby preventing Na reabsorption by principal cells (54,171) (refer to BP Regulation in Pregnancy section) and may also prevent K excretion (170). In this regard, the observations that, in some women with primary hyperaldosteronism, both BP and serum K levels are normalized during gestation are of interest, and are consistent with an antimineralocorticoid effect of progesterone (172). During pregnancy, there is an increased extra-adrenal production of deoxycorticosterone, which may further contribute to Na retention (173). Natriuresis may also be increased independently of mineralocorticoid antagonism as a consequence of increased atrial natriuretic peptide levels (2,86,174), and possibly progesterone acting on proximal tubular reabsorption of sodium (52). Given the recent literature emphasizing the importance of the relationship between UNa, UK, and hypertension (175), additional studies of these electrolytes in hypertensive pregnancies are potentially important. Thus far, most studies of renal Na transporters and channels (including the Na+/K+ ATPase and the epithelial sodium channel) still need to be verified and reproduced in other animal studies.
ACID–BASE BALANCE IN PREGNANCY
During pregnancy, there is increased minute ventilation, alveolar ventilation, and tidal volume, which all lead to a decrease in arterial partial pressure of carbon dioxide (PCO2) and lower levels of circulating hydrogen ions (176–179). Progesterone is thought to be the stimulus for lowering PCO2 because progesterone levels are associated with both an increased minute ventilation and a lower PCO2 (176,180–182). Other potential triggers for increased ventilation in pregnancy include hypoosmolality and elevated ADH (179,182). In response to relative respiratory alkalosis, renal compensation occurs with a decrease in serum bicarbonate to levels in the 18 to 22 mEq/L range (179,183).
Kidney Disease in Pregnancy
Historically, women with CKD were often discouraged from becoming pregnant because of the high rates of maternal and fetal morbidity and mortality. With advances in neonatal intensive care, more sophisticated multidisciplinary maternal care, utilization of erythropoietin-stimulating agents as well as successful assisted reproduction, it is not unusual for women with CKD to become pregnant. These pregnancies are high-risk, and should be managed by a multidisciplinary team including a high-risk obstetrician and a nephrologist or internist. Women with a history of vasculitis and autoimmune disease should be closely monitored for immune activation that may resemble superimposed preeclampsia, whereas women with morphologic renal diseases (urinary tract malformation) are at a higher risk for infection (184). Specialists who are trained to care for pregnant women should be sought for the care of women with high burden of disease. It is also important to pursue birth control options and family planning in any woman of reproductive age with CKD in order to preemptively discuss risks and benefits of pregnancy in the context of the individual patient’s psychosocial and medical conditions (185).
CHRONIC KIDNEY DISEASE IN PREGNANCY
Stages of Chronic Kidney Disease and Effect on Pregnancy Outcomes
A diagnosis of CKD doubles the risk for adverse maternal outcomes and for preterm delivery (186,187). Fetal and maternal outcomes including preeclampsia, gestational hypertension, preterm birth, intrauterine growth restriction (IUGR; fetal size below the 10th percentile), SGA infants (birth weight below the 10th percentile), and even death are more common in women with a history of CKD (186,187,189). CKD, independent of its etiology (188) or other comorbidities (187,190), leads to worse pregnancy outcomes.
Even women with a normal GFR or stage 1 CKD are at an increased risk for adverse fetal outcomes (preterm birth, SGA, need for neonatal intensive care), new onset hypertension, new/doubling proteinuria; but not intrauterine fetal demise compared to women without CKD (188,191). Multigravid pregnancies increase fetal risks for an earlier delivery, low birth weight, intensive care needs, and even mortality, and if possible should be avoided in women with CKD (192).
Women with more advanced CKD (stages 4–5) at the start of pregnancy have worse maternal and fetal outcomes than women with milder CKD (stages 1 or 2) (191,193). When GFR is between 60 and 90 mL/kg/1.73 m2, the odds of developing preeclampsia or worsening renal function are increased by 6.75-fold compared to normal pregnancies, and the odds of developing adverse fetal outcomes (premature birth, IUGR, fetal death, low birth weight) triple (194). In women with an even lower GFR (<30 mL/kg/1.73 m2), prematurity rates increase further and reach 89% (as opposed to 41% with baseline GFR 45–100 mL/kg/1.73 m2, P = 0.02) (195).
Effect of Pregnancy on Renal Function in Chronic Kidney Disease
A meta-analysis of over 500,000 pregnancies showed that despite higher risk of poor pregnancy outcomes (preeclampsia, preterm birth, or SGA) in women with CKD stages 1 to 3, pregnancy was not clearly associated with long-term worsening in renal function (196). In women with preserved renal function, CKD 1, or mild renal impairment (Cr < 1.4 mg/dL), pregnancy does not seem to lead to long-term worsening of renal function (197,198). The risk profile is different for women who do not have a preserved renal function (with a Cr ≥ 1.4 mg/dL) or who have hypertension (199–202). Women with advanced CKD at baseline, however, are at a higher risk for deterioration in renal function as a result of pregnancy.
A baseline GFR below 40 mL/min/1.73 m2 significantly increases the risk for further renal function decline in pregnancy (203). In a study of 98 pregnant with CKD, having a GFR > 90 mL/min/1.73 m2 did not result in worsening in renal function (defined as a 25% increase in creatinine). Nevertheless, 41.3% of women with GFR 60 to 90 mL/min/1.73 m2 and 60% of those with GFR 15 to 59 mL/min/1.73 m2 developed worsening in renal function during pregnancy (P = 0.001) (194). As previously discussed, estimation of GFR using creatinine-based formulas may not accurately reflect renal function during pregnancy; thus, serum creatinine levels are recommended for monitoring renal function in these patients.
Katz et al. reported that in 121 women with mild renal insufficiency (serum creatinine level less than 1.4 mg/dL), only 16% had worsening renal function that was reversible in all cases (197). The risk for preterm birth was approximately 20%, with a 24% risk for IUGR and favorable fetal survival rates (89%) (148,197,204–206).
Jones and Hayslett reported that in women with prepregnancy serum creatinine level of 1.4 to 2.5 mg/dL, 50% developed worsening renal function (200), and among women who developed worsening renal function during pregnancy, 23% progressed to dialysis dependency within 6 months of delivery, whereas the rest recovered renal function (200). Older studies report fetal survival rates of 76% to 80% in women with moderate renal insufficiency (207–209). In a small study of 29 pregnant women with CKD, by Sato et al., four of seven women with moderate renal insufficiency were initiated on dialysis during pregnancy for blood urea nitrogen (BUN) >100 mg/dL (202).
Women with a prepregnancy serum creatinine above 2.5 mg/dL are at a high risk (40%) for developing dialysis dependency during pregnancy or postpartum. Preeclampsia is also very common in this group and affects at least 40% of pregnancies. Fetal outcomes are poor: 73% preterm birth and 57% IUGR (200). All of the 17 women with a prepregnancy creatinine >2.5 mg/dL in Sato et al.’s study were started on dialysis during pregnancy (202). Given the high fetal and maternal morbidity, we do not recommend pregnancy in women with this degree of renal dysfunction. A discussion of other options such as adoption or, when available, surrogacy is advised. In addition to reduced GFR, proteinuria of more than 1 g/day is associated with poor pregnancy outcomes (188,191,203).
The mechanism for worsening renal function in pregnant women with underlying CKD is not fully established. Experimental models have suggested that the etiology of CKD may be an important factor in glomerular response to pregnancy. Baylis et al. performed micropuncture experiments in a variety of experimental models of CKD during pregnancy. In all models that were studied, the glomerular capillary pressure (PGC) was not increased, suggesting that glomerular hypertension is not the primary cause of deterioration in renal function during pregnancy. The lack of increase in PGC is mediated by vasodilation of either the systemic or renal vasculature, depending on the cause of CKD. In the rat model of anti-basement membrane glomerulonephritis in pregnancy, renal vasodilation prevents further increases in PGC. In the 5/6 nephrectomy model, renal vasodilation is associated with a decrease in PGC (210). In the rat model of membranous glomerulopathy in pregnancy, there is decreased systemic BP, reduced PGC, and preferential constriction of the renal afferent arterioles.
There are data suggesting that hypertension is an important risk factor for adverse outcomes in pregnancies in women with CKD (201,211). Masuyama et al. reported that fetal outcomes (gestational age at delivery, need for intensive care unit, SGA, Apgar score) are more closely associated with elevated BP than proteinuria (212).
Hereditary Kidney Disease
Polycystic Kidney Disease
Autosomal dominant polycystic kidney disease (ADPKD) is the most common form of hereditary kidney disease, and reproductive age women may have hypertension and CKD, increasing their risk for pregnancy complications (213). Subclinical disease is occasionally present at conception, and a diagnosis is occasionally first made during pregnancy. Genetic counseling is recommended in women with a family history of ADPKD.
Women with ADPKD are at increased risk for complications compared to unaffected family members, especially if they have hypertension (213). The risks for adverse fetal events are increased with maternal age >30, reduced GFR, hypertension, and superimposed preeclampsia (213). A recent study of 92 pregnancies in women with ADPKD and well-preserved kidney function found an increased risk for hypertension, proteinuria, renal dysfunction, and preeclampsia compared to a control group of women with simple renal cysts (214). Rates of premature birth or spontaneous abortion were similar to those in the control group (214). The literature also suggests an increased risk of incident and recurrent urinary tract infections (UTIs) (214,215), and we recommend frequent urine cultures during pregnancy.
Repeated pregnancies may adversely affect long-term renal function in women with ADPKD (213) and increase the risk for the development and enlargement of liver cysts (216). Screening for cerebral aneurysms should be considered prior to labor, especially in women with a family history of cerebral aneurysms.
Alport Syndrome
There are only a few reports of pregnancy in women with Alport syndrome, and as in women with other etiologies of CKD, the risks of maternal and fetal complications are increased. In one small series of 18 pregnancies, deterioration in renal function (in 5/18 pregnancies), preterm birth (10/18), and stillbirth (2/18) were common (217). Evidence for the management of Alport syndrome in pregnancy is limited to case reports and small case series which suggest that in women with reduced GFR and hypertension, complications are more common (218,219)
Lupus Nephritis
Lupus nephritis during pregnancy presents unique problems. Although similar considerations apply regarding the relationship between level of renal function and BP to pregnancy outcome, in general, lupus is a much more unpredictable illness, because of the tendency of the disease to flare (220). Increased organ damage following pregnancy in women with lupus is associated with pregnancy duration, total disease duration, and disease activity and damage prior to pregnancy (221). Whether or not pregnancy per se is a risk factor for lupus flares has been disputed. Although some report no increase in flares attributable to pregnancy in patients in remission, prospective data suggest that pregnancy is in fact associated with a greater chance of disease exacerbation (222). Women with lupus nephritis are at greater risk for adverse maternal and fetal outcomes compared to women without renal involvement and are advised not to conceive unless their disease has been “inactive” for the preceding 6 months, because there is a higher incidence of fetal demise when disease is active at conception. Inactive disease usually means a creatinine measurement of <0.7 mg/dL or 62 mmol/L, proteinuria less than 0.5 g/day, and on spun urine examination, fewer than five red blood cells per high powered field. If there is active disease with a creatinine of >1.2 mg/dL or 106 mmol/L, fetal loss occurs in 25% to 50% (223).
Additional complications associated with lupus and pregnancy include placental transfer of maternal autoantibodies, which can cause a neonatal lupus syndrome characterized by heart block, transient cutaneous lesions, or both. Women with lupus are also more likely to have clinically significant titers of antiphospholipid antibodies (anticardiolipin antibody or lupus anticoagulant) which are associated with spontaneous fetal loss of 50% to 75%, hypertensive syndromes indistinguishable from preeclampsia, and thrombotic events including deep vein thrombosis, pulmonary embolus, myocardial infarction, and stroke (224). Thus, all women with systemic lupus erythematosus (SLE) should be screened for antiphospholipid antibodies early in gestation. When titers are elevated (more than 40 GPL), low-dose daily aspirin (80–325 mg) is recommended. If there is a history of thrombotic events, then low molecular weight heparin (LMWH) in combination with aspirin is recommended. Therapy with unfractionated or LMWH in addition to aspirin has been advocated for secondary prevention in women with a history of stroke, myocardial infarct, deep vein thrombosis, pulmonary edema, or placental thrombosis (225).
One of the difficulties in managing lupus nephritis during pregnancy is that increased activity of lupus may be difficult to distinguish from preeclampsia. Both are characterized by an increase in proteinuria, a decrease in GFR, and hypertension. Thrombocytopenia may also be observed in both conditions. Hypocomplementemia is not a feature of preeclampsia, whereas increases in liver function tests may be observed in preeclampsia but are not characteristic of lupus activity. If disease activity is present before 20 weeks of gestation, then the diagnosis is more likely to be a lupus flare. In the latter half of pregnancy, it may be impossible to distinguish between a renal lupus flare and preeclampsia. In fact, frequently both are present simultaneously as increased lupus activity often triggers increased BP and proteinuria, which is indistinguishable from preeclampsia. Spun urine microscopy for red blood cell casts can also signal lupus nephritis activity. Biomarkers such as fms-like tyrosine kinase-1 (sFlt1) and placental growth factor (PlGF) may help discriminate between these two conditions. A recent study demonstrated that, in women with SLE and/or antiphospholipid syndrome (APS), an sFlt1 level <1,872 pg/mL and PlGF >70.3 pg/mL has a negative predictive value of 95% for preeclampsia and for adverse pregnancy outcomes in women with SLE and/or APS with a negative predictive value 95% (226). Unfortunately, delivery may be necessary if immunosuppressive therapy and supportive care fails to stabilize the condition.
The approach to treatment of lupus nephritis during pregnancy is based largely on anecdotal experience and knowledge regarding treatment of lupus in nonpregnant patients.
Glomerulopathies
Glomerulonephritis in Pregnancy
Glomerulopathies may be present in women of childbearing age. They are sometimes not diagnosed before pregnancy but are then detected during routine prenatal care. Glomerulopathies most often encountered in younger women include immunoglobulin A (IgA) nephropathy, focal segmental glomerulosclerosis, membranoproliferative glomerulonephritis, minimal change disease, and lupus nephritis.
Women in whom proteinuria or hematuria is first detected during pregnancy are likely to have glomerulonephritis, especially in early pregnancy when preeclampsia is rare. However, in the late second and third trimesters, urinary abnormalities and hypertension may also be caused by superimposed preeclampsia. If the serum creatinine is elevated, it is helpful to know the baseline level from either early pregnancy or prepregnancy. Significant elevations in serum creatinine (e.g., >1.5 mg/dL) are unusual in women with preeclampsia, particularly if BP is normal and proteinuria minimal, and are more likely caused by the underlying primary kidney disease. Although most women with preeclampsia usually have only mild reductions in GFR (not greater than 30%), preeclampsia remains the most common cause of acute kidney injury (AKI) in pregnancy (see below).
If glomerulopathy is suspected, evaluation with renal ultrasonography, urinalysis, quantification of proteinuria, and serologic testing is recommended. Renal biopsy can be done, especially early in pregnancy when the patient is more easily positioned on her abdomen. We usually reserve kidney biopsy for women with worsening renal function, and/or heavy proteinuria in whom treatment decisions are likely to be altered. Several case series suggest that kidney biopsy in pregnancy can be helpful in improving the outcome of pregnancy in women with new onset or worsening CKD in pregnancy (230–232). Bleeding may be more likely because of the gestational increase in renal blood flow, and caution should be exercised when BP is uncontrolled (233–235). In women with near-normal GFR and without debilitating nephrotic syndrome or hypertension, it is usually safe to defer renal biopsy until after delivery. The timing for postpartum biopsy depends on the extent of renal function impairment. Proteinuria may improve once gestational hyperfiltration resolves.
Pregnancy outcomes and prognosis for maternal kidney function are dependent on the baseline GFR, presence of hypertension, and degree of proteinuria (230,236–238). There are few data to suggest an association with histologic diagnosis of glomerulonephritis and differential outcomes in pregnancy (230,231,239). Regardless of the type of glomerulonephritis, it is not uncommon for proteinuria to increase during pregnancy owing to the hemodynamic and hormonal alterations. However, if renal function is well preserved, pregnancy does not appear to adversely affect long-term renal prognosis (at 5 years) (230,237,238). Whether newly diagnosed nephrotic syndrome or worsening proteinuria during pregnancy should be treated has not been prospectively studied, and principles of treatment in nonpregnant women are generally applied. For example, a new diagnosis of minimal change glomerulonephritis in a pregnant woman would be treated with prednisone, whereas IgA nephropathy would most likely be managed conservatively. The optimum strategy for treatment of focal segmental glomerulosclerosis is less clear—oral steroids may be considered if levels of proteinuria are greater than 5 g/day, and if renal function is deteriorating; however, there are few data that specifically address this clinical challenge.
Diabetic Nephropathy
Diabetic nephropathy is a risk factor for poor maternal and fetal outcomes and is present in approximately 5% of pregnant women with type 1 diabetes mellitus (240), particularly those with type 1 diabetes for over 10 to 15 years. Proteinuria may increase during pregnancy, and in women with more advanced CKD, decreases in GFR may occur as pregnancy progresses (241). Women with normal GFR and microalbuminuria at baseline generally have excellent outcomes; however, the risk of preeclampsia and preterm delivery increases with macroalbuminuria and reduced GFR (196,240–243). Women with type 1 diabetes with microalbuminuria, normal renal function, and normotension should be encouraged not to postpone pregnancy because of the worse prognosis once overt nephropathy develops.
Management of pregnant women with diabetic nephropathy includes glycemic control, treatment of hypertension, and close monitoring of maternal and fetal well-being. Angiotensin-converting enzyme inhibitors and angiotensin receptor blockers are contraindicated during all three trimesters of pregnancy and are associated with a neonatal mortality rate of 25% in the second and third trimesters; therefore, women should be switched to other agents prior to conception (244). Although the evidence is controversial that these agents are associated with fetal malformations with first trimester exposure, we recommend avoiding their use during pregnancy. There is some evidence that optimum control of BP to normal levels is associated with improved pregnancy outcomes in women with diabetic pregnancy (245). Low-dose aspirin reduces the incidence of preeclampsia in women at high risk, and can be considered in women with diabetic nephropathy (243,245,246).
CKD is not as common in women in their childbearing years with type 2 diabetes. A multicenter analysis from Japan suggests that risk factors for adverse maternal and fetal outcomes are similar in women with type 1 and type 2 diabetes. This study demonstrated no differences in the rates of perinatal mortality and congenital malformation between pregnant women with type 1 and type 2 diabetes; however, women with type 2 diabetes displayed a higher risk of primary cesarean section (247). A meta-analysis of pregnancy outcomes in over 500,000 pregnancies reported better outcomes in women with diabetic CKD compared to other etiologies, although the reasons for this are unclear (196).
Urinary Tract-Related Renal Disease
Recurrent UTIs leading to CKD are often caused by anatomic abnormalities of the urinary tract. The most commonly encountered urinary tract-related renal diseases are vesicoureteral reflux (VUR) and chronic pyelonephritis.
VUR nephropathy is a common cause of CKD in young women and is associated with a greater risk for UTI and pyelonephritis during pregnancy. If baseline renal function is abnormal and hypertension present, there is an expected increased risk for preeclampsia; one prospective study of 54 pregnancies reported a 24% incidence of preeclampsia and an 18% incidence of worsening renal function (248,249). Preterm birth risk has been reported to be as high as 30% (249). Renal scarring is thought to be associated with adverse maternal and fetal outcomes (250–253). The children of women with VUR are at increased risk for VUR, because it is believed to be an autosomal dominant disorder with incomplete penetrance (249).
Given the increased risk of UTI, including pyelonephritis, close monitoring for early detection of bacteriuria with frequent urine cultures (at least once a month or if symptomatic) is recommended. Antibiotics should be initiated if bacteriuria is documented and should be tailored to culture and sensitivity results and should take into account pregnancy or fetal safety profiles.
STONE DISEASE AND PREGNANCY
Symptomatic renal stones are not uncommon in pregnancy; they complicate 1/200 to 1/1,500 of gestations (254–257). Women typically present in the third or second trimester with flank or abdominal pain and hematuria (254–256,258). Renal colic often occurs during pregnancy in women without a prior history of nephrolithiasis, and the diagnosis of renal stone can often be missed (254,259,260).
Gestational elevation in GFR, increased urine supersaturation, hypercalciuria, and decreased ureteral peristalsis should theoretically increase the risk of stone formation (258,261–264). However, the incidence of renal stones in pregnancy is comparable to that in nonpregnant populations. This is possibly caused by increased urinary magnesium, citrate, pH, and nephrocalcin, which protects from stone formation (258,261). The gestational increase in urinary pH and citrate is protective for uric acid and calcium oxalate stones; however, in pregnancy, unlike other populations, the majority of kidney stones are composed of calcium phosphate (265,266).
Renal ultrasonography is the first-line imaging modality for the diagnosis of nephrolithiasis in pregnancy, followed by magnetic resonance urography (without gadolinium) and noncontrast low computed tomography (257). Women with renal colic and hydronephrosis but without visualization of an obstructing calculus on ultrasound may still have a stone on ureteroscopy (20.5%) (267).
Supportive management with analgesics (acetaminophen or opiates but not nonsteroidal antiinflammatory drugs) and hydration is the mainstay of management. Renal calculi will pass or spontaneously fragment in 70% to 84% of cases (259,264,267,268). Ureteroscopy with stenting or stone retrieval is not a very risky procedure but should be performed only when the patient has a large (>1 cm) stone, persistent pain, sepsis, worsening hydronephrosis, an obstructed solitary kidney, or a high-grade obstruction (259,264).
Renal stones in pregnancy have been associated with pyelonephritis, preterm labor, spontaneous abortions, hypertension, and gestational diabetes (255,256,267,269). Interestingly, perinatal outcomes are not significantly altered in women with nephrolithiasis (266,270).
PREGNANCY IN WOMEN WITH END-STAGE RENAL DISEASE
Case reports and case series over the years have clearly documented poor pregnancy outcomes in women with end-stage renal disease (ESRD), and until recently, such pregnancies were rare and hazardous with less than 50% leading to a live birth. Fertility is impaired in women with ESRD at least in part owing to impaired hypothalamic luteinizing hormone release and anovulatory cycles (271). Additional reported abnormalities in reproductive hormones include elevated prolactin levels and decreased levels of estrogen and progesterone (272). Nevertheless, pregnancies do occur, and the pregnancy rate in women with ESRD appears to be increasing, possibly owing to implementation of more aggressive dialysis prescriptions and better control of comorbidities such as anemia and hypertension. In women with advanced CKD, who may not be ready to start dialysis, if pregnancy occurs, many nephrologists will initiate dialysis earlier because of emerging data that more aggressive treatment of the uremic environment is associated with improved maternal and fetal outcomes (273,274).
Hemodialysis
The literature on pregnancy outcomes consists mostly of case reports and case series that have documented a high rate of complications including a very high likelihood for preterm birth (67%–100% incidence), maternal hypertension (20%–66% incidence), IUGR (14%–80% incidence), need for neonatal intensive care (33%–100% incidence), and fetal demise (275). More recently, outcomes have improved most likely in response to increasing the time on dialysis and more aggressive treatment of uremia. A majority of pregnancies treated aggressively now result in live births (81.33%) without major complications (in 75% of pregnancies) (275,276). Some reports suggest that a predialysis BUN of 50 mg/dL or less is associated with a higher rate of live birth and longer gestation (277–279). Neonates born from women with very elevated BUN levels should be monitored for osmotic diuresis.
A higher dose of hemodialysis (HD) is associated with higher birth weight (211,280,281), gestational age at delivery (211,281), and lower fetal demise rates (281). A comparison of 22 pregnancies from the Toronto Pregnancy and Kidney Disease Registry and 70 pregnancies from the American Registry for Pregnancy in Dialysis Patients revealed that as more HD is administered, the rates of live birth increase significantly (48% live birth rates for women on ≤20 hours of HD weekly vs. 85% live birth rate for women on ≥37 hours weekly). Nightly HD for 6 to 8 hours was shown to be associated with excellent outcomes in a case series of five patients (282).
There is still no widely accepted dialysis schedule for pregnancy (211,275). Evidence suggests that more dialysis may reduce neonatal death and stillbirth (275). Frequent HD (≥5 times/week) with a total dose of at least 37 hours/week and maintaining BUN ≤50 mg/dL is a reasonable and safe strategy, although this schedule is rigorous and may present logistic and financial challenges. Home dialysis technologies (283) may decrease the burden of frequent and prolonged dialysis on pregnant women.
There are no data about the optimal dialysate and heparinization protocol for HD during pregnancy. Minimization of heparin is reasonable in order to prevent obstetric bleeding. In addition, the bicarbonate concentration in the dialysate can be decreased to 25 mEq/L in order to maintain the physiologic metabolic acidosis of pregnancy.
During pregnancy, anemia is often more pronounced, resulting in an increased requirement for erythropoietin and iron supplementation (282,284). There are limited data regarding appropriate management of calcium, phosphorus, and vitamin D in pregnant dialysis patients. Considerations that may provide guidance are that calcium needs increase in pregnancy, increased dialysis may permit liberalization of phosphorus intake, and 1,25-D levels may be increased because of placental 25-hydroxylation of vitamin D. Dry weight titration in pregnancy is challenging because of physiologic volume expansion and edema and should be attempted cautiously.
Peritoneal Dialysis
Peritoneal dialysis (PD) has outcomes that are similar to HD in the general population. However, during pregnancy the equivalence of both treatments has not been compared rigorously. A meta-analysis of 72 pregnant women on HD and 14 women on PD reported a lower incidence of SGA birth with HD (31%) rather than PD (67%, P = 0.015) (211). More data from PD patients are required to verify these findings.
In women who are dialyzed with PD during pregnancy, the exchange volume will need to be decreased and therefore, clearance will need to be achieved by increasing the frequency of exchanges, preferably using a cycler (285). As in nonpregnant women on PD, protein intake should be encouraged and the usual intake recommendation of 1 g/kg/d protein may need to be increased by 20 g/day (282).
Renal Transplantation
Kidney transplantation restores fertility and offers the possibility of near-normal reproductive function including successful pregnancy. Thousands of pregnancies in renal transplant recipients have been reported in case reports and registries since the first successful pregnancy was reported in 1963 (286).
Although pregnancy outcomes are excellent in transplant recipients with near-normal GFR and normal BP, similar to women with CKD, the rate of complications such as preeclampsia and preterm birth is increased. A consensus conference generated a report in 2005 that summarized the literature, proposed practice guidelines, and identified gaps in knowledge (287). Most pregnancies (greater than 90%) that proceed beyond the first trimester succeed; however, there are maternal and fetal complications resulting from immunosuppressant effects, preexisting hypertension, and renal dysfunction. These include maternal complications of steroid therapy such as impaired glucose tolerance, hypertension (47%–73%), preeclampsia (30%), and increased infection. Fetal complications include a 45% to 60% incidence of preterm delivery (mean gestational age is 36 weeks) and IUGR with lower birth weight (average 2.3–2.6 kg). It is recommended that women wait a minimum of 1 year posttransplant to conceive (288). This permits stabilization of immunosuppression regimens, and identification of women with reduced renal function and uncontrolled hypertension in whom pregnancy should be discouraged. Characteristics that are associated with improved pregnancy outcomes include absence of recent rejection, normal BP, absence of proteinuria, preserved renal function (serum creatinine of less than 1.5 mg/dL), being on lower doses of immunosuppressants (≤15 mg/day prednisone, ≤2 mg/kg/d, ≤5 mg/kg/d cyclosporine), and absence of pelvicalyceal dilatation (287,289,290).
Pregnancy in a renal transplant recipient should be monitored by a multidisciplinary team of obstetricians, transplant specialists, and internists familiar with the care of pregnant women (291). Monitoring should start before conception, when possible, in order to optimize risk factors and promote normal body mass index, control BP and institute pregnancy-safe immunosuppressive regimens. Similar to women with CKD of any etiology, maternal and fetal outcomes are best when GFR and BP are normal (96,292). The risk of transplant rejection is not significantly elevated, and rejections are often associated with medication nonadherence (292).
Medication-related complications may be encountered during pregnancy. Women on steroid-containing regimens are at risk for impaired glucose tolerance, hypertension (47%–73%), preeclampsia (30%), and infections. Pregnancy itself may lead to the development of antibodies directed at spouse or offspring. This phenomenon may be relevant in women who receive a subsequent transplant from the spouse or offspring, because donor-specific antibody levels may rise after transplantation from such donors, and women receiving human leukocyte antigen antibody-incompatible transplants from their partners or children have a higher rejection rate (293).
The impact of immunosuppression on maternal and fetal outcomes has been assessed with respect to fetal malformations, preterm birth, and hypertensive complications. A recent report suggested that the risk of preeclampsia was also increased in pregnancies fathered by males on immunosuppression after kidney transplantation (294).
Calcineurin inhibitors are used widely and considered safe, but are associated with a higher rate of preeclampsia and gestational hypertension compared to azathioprine, and possibly with slightly shorter gestations. Tacrolimus levels (both free and albumin bound) are altered during pregnancy as a result of multiple factors including anemia and hypoalbuminemia (295), thus making interpretation of whole blood levels difficult (296). It is likely that in many women, tacrolimus availability is increased despite lower whole blood trough levels. Suggested strategies for dosing include not adjusting the dose until whole blood levels reach 50% of prepregnancy levels, or maintaining the whole blood level constant and carefully monitoring for signs of drug toxicity. Sirolimus is classified as a pregnancy risk C medication, and its safety is not established in human pregnancies (297). Mycophenolate mofetil is embryotoxic in animals and has been associated with ear deformities, cleft lip/palate, and first trimester spontaneous abortions in human pregnancies (298), and should be avoided in pregnancy and in women who are planning to become pregnant at least 6 weeks before conception. Azathioprine can be used instead of mycophenolate mofetil in these women.
Detailed immunologic studies have not been reported in either pregnant renal transplant recipients or their offspring. Acute graft rejection during pregnancy occurs uncommonly and may be difficult to diagnose and differentiate from other causes of AKI or preeclampsia. Transplant biopsy may be necessary to make an accurate diagnosis; however, the logistics are complicated in late pregnancy by the enlarging uterus and if renal function is deteriorating, early delivery may be necessary. The treatment for rejection may include intravenous steroids and intravenous immunoglobulin (287). The safety of antilymphocyte antibodies and rituximab is not well established in pregnancy (287).
Transplant Donor
In recent years, several studies have raised concerns that women who donate a kidney may be at an increased risk of developing preeclampsia in later pregnancies (299–301). The incidence of preeclampsia was 11% in donors compared to 5% in a matched control group. Despite the increased incidence of preeclampsia and gestational hypertension in donors, there was no statistically significant difference in pregnancy outcomes: preterm birth and birth weight (301). Overall, it is important to discuss the increased risk of preeclampsia with any female kidney donor of reproductive age.
Pregnancy-Specific Renal Disorders
HYPERTENSION IN PREGNANCY
Hypertension is the most common complication of pregnancy and affects 6% to 10% of pregnancies (302). Hypertension is potentially a highly morbid and even lethal complication of pregnancy, and although rare, most pregnancy-related strokes are attributable to hypertension (303,304).
Hypertension in pregnancy is defined as a BP of 140/90 mm Hg or above. Mild to moderate hypertension refers to BP 140 to 159/90 to 109, and severe hypertension refers to BP 160/110 mm Hg or above (305).
Although several different classification schemas are used worldwide, the American College of Obstetricians and Gynecologists (ACOG) and the National Hypertension Education Program endorse the distinction of four major categories (101): preeclampsia-eclampsia; chronic hypertension; chronic hypertension with superimposed preeclampsia; and gestational hypertension.
Preeclampsia
Clinical Manifestations and Diagnosis
Preeclampsia affects 2% to 5% of pregnancies (306–308) and is responsible for 12% to 15% of maternal deaths (309). The traditional hallmarks of preeclampsia include new onset hypertension in the latter half of pregnancy with proteinuria. More recently, the ACOG has acknowledged that nonproteinuric forms of preeclampsia occur and have modified their diagnostic criteria to that effect (101). Thus, their recommended diagnostic criteria include new onset hypertension (BP ≥140/90 mm Hg) after 20 weeks of gestation and one of the following symptoms/signs, which cannot be attributed to another disease process:
• De novo proteinuria: >0.3 g/day on 24 hours urine collection or urine protein/creatinine ratio >0.3. Both quantification methods are considered adequate (101,310–312). A dipstick with 1+ protein can be used alternatively only when quantitative testing is not available.
• Neurologic symptoms
• Thrombocytopenia: platelets <100,000/mL
• Pulmonary edema
• Transaminitis: alanine aminotransferase (ALT) or aspartate aminotransferase (AST) above twice the normal range
• Renal insufficiency: creatinine >1.1 mg/dL or doubling from baseline.
Severe features of preeclampsia include: BP ≥160/110 mm Hg, platelet count <100,000 μL, pulmonary edema, transaminitis, severe right upper quadrant pain, creatinine >1.1 mg/dL or doubling, or new onset of cerebral or visual symptoms. The HELLP (Hemolysis, Elevated Liver enzymes, Low Platelets) syndrome and eclampsia (features of preeclampsia and seizures) are both considered to be severe variants of preeclampsia rather than separate diseases.
Risk Factors for Preeclampsia
Risk factors for preeclampsia include (313–316):
• African American race
• chronic hypertension (especially hypertension secondary to renovascular hypertension, pheochromocytoma or primary aldosteronism)
• thrombophilia
• diabetes mellitus
• chronic kidney disease
• obesity
• prior history of preeclampsia
• family history of preeclampsia
• primigravida
• maternal age >40 years
• multifetal pregnancy
Pathophysiology of Preeclampsia
The last decade has seen progress in our understanding of the pathophysiology of preeclampsia, although there remain significant gaps. Preeclampsia may be conceptualized as a two-stage process; the placenta is central to the pathophysiology of the syndrome, and this early stage is characterized by impaired uteroplacental blood flow (317). Studies suggest that defective remodeling of the terminal branches of the uterine artery (spiral arteries) is a fundamental lesion that then results in impaired placental perfusion. During normal pregnancy, placental cytotrophoblasts relocate from chorionic villi and invade the uterus, reaching the inner third of the myometrium and deeply invade the spiral arteries. Cytotrophoblasts migrate up these vessels and replace the maternal endothelial lining (317). The spiral arteries then acquire physiologic properties that permit adequate placental perfusion. In preeclampsia, cytotrophoblast invasion of the interstitial uterine compartment is shallow, although this is a variable finding. In many locations, spiral artery invasion is incomplete and some vessels retain portions of their endothelial lining with relatively intact muscular coats, although others are not modified. This impaired placental perfusion is believed to initiate a sequence of events that include (317) immune dysregulation, ischemia, and release of angiogenic factors into the maternal circulation, setting the stage for the second stage of preeclampsia, which encompasses the multifaceted maternal syndrome (318). The hallmark of the maternal syndrome is vascular endothelial injury.
Several circulating placental derived factors may contribute to maternal vascular endothelial injury, including sFlt-1, soluble endoglin (319), hypoxia-inducible factor-1 (HIF-1), syncytiotrophoblast debris, and various cytokines. Angiotensin II type 1 (AT1) receptor agonistic antibodies have also been reported to be involved in the pathogenesis of preeclampsia in animal models as well as in clinical cases of preeclampsia (320). Elevations of sFlt-1 and endoglin have been reported prior to and at the time of clinical disease (321,322). sFlt-1 is a circulating antagonist of VEGF released from the placenta, and is hypothesized to contribute to maternal vascular endothelial dysfunction and to cause glomerular endotheliosis (323). PlGF, an angiogenic factor similar to VEGF, is decreased in women with preeclampsia. The endothelial damage caused by placentally derived circulating factors results in maternal hypertension and dysfunction in the kidney, liver, and brain.
Preeclamptic Hypertension
Elevated BP is often the first clinical sign that preeclampsia is present; it is the clinical feature that is frequently the proximate cause of preterm delivery, and also an important risk factor for maternal intracerebral hemorrhage (324). Subtle increases in BP are detectable weeks before preeclampsia is diagnosed in women who develop the disease (325). In many women, it is the defining feature of the syndrome, with severe BP elevations that persist for days or weeks postpartum, whereas in others the hypertension is mild, asymptomatic, and disappears quickly after delivery. As mentioned above, normal pregnancy is characterized by early, marked vasodilation and lower BP compared to the case when nonpregnant. When preeclampsia develops, there is early and sustained suppression of the circulating renin–angiotensin system (36,47,326), and there are lower levels of relaxin (327) and altered levels of estrogen and progesterone (328), suggesting that decreased vasodilation contributes to preeclamptic hypertension. There is also evidence for excessive vasoconstriction with increased endothelin (329), deficient prostacyclin (330), increased sympathetic nervous system activity (59–61), and, as mentioned, agonistic autoantibodies to the AT1 receptor in some cases (331). Preeclampsia shares some clinical features with acute glomerulonephritis such as acute onset of hypertension, mildly reduced GFR, proteinuria, and edema. Although hypertension associated with glomerulonephritis is heterogeneous, clearly in some patients there is a sodium volume component in patients with acute decrements in GFR; the suppressed PRA (36) and increased ANF (332), which are features of preeclampsia, are consistent with excess volume (333,334). Underlying genetic heterogeneity and predisposition may play a role in the degree to which a woman will manifest hypertension when she develops preeclampsia, and this may explain some of the earlier genetic association studies that reported associations between single nucleotide polymorphisms in the angiotensinogen gene (335), angiotensin-converting enzyme gene (336), genes involved in the nitric oxide pathway (337), and risk for preeclampsia.
Serum levels of sFlt-1 are increased in women several weeks prior to the development of preeclampsia and until delivery, after which they fall rather quickly (338,339). sFlt-1 antagonizes the proangiogenic biologic activity of circulating VEGF and PlGF by binding to them and preventing their interaction with their endogenous receptors (323). Along with the increased circulating sFlt-1 levels, both PlGF and VEGF levels are decreased in women with preeclampsia. As VEGF is necessary for healthy vascular endothelial function, a plausible link between placental dysfunction and hypertension in preeclampsia is the presence of antiangiogenic factors, which, by blocking VEGF, cause endothelial dysfunction and hypertension. Support for this hypothesis includes the numerous clinical studies, demonstrating increased levels of sFlT-1, decreased PlGF and VEGF in women with preeclampsia, and a rising sFlt-1/PlGF ratio preceding the clinical manifestations of the syndrome (323,340–344). Also, experimental models of preeclampsia demonstrate that preeclampsia can be reproduced in a rodent model with administration of exogenous sFlt-1 (339).
Another particularly intriguing observation is that patients treated with chemotherapeutic agents that target the VEGF signaling pathway develop a preeclampsia-like syndrome characterized by hypertension, proteinuria, central nervous system dysfunction resembling the posterior reversible leukoencephalopathy syndrome and, at times, microangiopathy (345,346). The mechanism of antiangiogenic therapy-induced hypertension demonstrates similarities to that observed in women with preeclampsia—decreased nitric oxide production and increases in circulating endothelin levels (346). In experimental models, administration of an endothelin receptor antagonist has been shown to prevent the development of antiangiogenic therapy-induced hypertension (347). Angiogenic factors, especially sFlt-1 and PlGF, are promising biomarkers for preeclampsia but remain a research tool as they are not commercially available in the United States. Their use has shown promise in distinguishing worsening hypertension, lupus, or CKD from preeclampsia (212).
Renal Manifestations of Preeclampsia
Glomerular endotheliosis (Fig. 13-3) is a pathognomonic, yet not exclusive, feature of preeclampsia (348–350). Experimental as well as clinical data suggest that increased circulating levels of sFlt-1 and soluble endoglin may contribute to the pathogenesis of glomerular endotheliosis by binding to circulating VEGF. Glomerular endotheliosis is similar to the lesion described in patients who have developed proteinuria after treatment with antiangiogenic chemotherapy (7,323,351) and is characterized by a reduced number of fenestrae (352) and anionic binding sites (353,354).
Renal biopsy studies performed within 48 hours of delivery on preeclamptic women, with a slightly decreased GFR (mean GFR of 91 ± 25 mL/min/1.73 m2 compared to 149 ± 34 mL/min/1.73 m2 in control healthy pregnant women) demonstrate thickened basement membrane, reduced number of fenestrae and anionic binding sites, as well as subendothelial fibrinoid deposits that lower glomerular permeability (352,353). In addition, mesangial cell interposition restricts the filtration area and lowers the ultrafiltration coefficient (Kf), leading to the conclusion that the reduced GFR in preeclampsia has a primarily structural basis with a contribution of reduction in RPF (23,78,352).
Serum creatinine rarely rises to levels that are considered abnormal for nonpregnant individuals in women with preeclampsia, and in most women, serum creatinine is <1.2 mg/dL—a level that, although not dramatic, would represent a 50% decrement from normal pregnancy levels, which are, on average, about 0.60 mg/dL.
Preeclamptic Proteinuria
Considered for decades to be one of the key diagnostic features of preeclampsia, proteinuria is present in most women who are diagnosed clinically as having preeclampsia. Although it is well recognized that women with hypertension in the second half of pregnancy and evidence of other systemic manifestations of preeclampsia such as the HELLP syndrome may not always manifest proteinuria, from the nephrologist’s perspective it remains an important feature of the disorder. As in most glomerular diseases, most of the protein excreted in the urine of preeclamptic women is albumin. The precise mechanisms for preeclamptic proteinuria had, until recently, been somewhat of a mystery. Until recently, the descriptions of the pathophysiology of preeclamptic proteinuria have emphasized alterations in the glomerular basement membrane and the endothelial cells. As already mentioned, VEGF plays an important role in vascular endothelial integrity. The elevated sFlt-1 levels in preeclampsia, which lead to reduced VEGF, are a likely candidate mechanism for preeclamptic proteinuria, a hypothesis supported by the animal studies demonstrating that reductions in VEGF lead to a renal lesion with histologic characteristics of glomerular endotheliosis (355). Moreover, recent small studies of extracorporeal removal of sFlt-1 in women with early preeclampsia demonstrated that protein to creatinine ratios rose and fell in conjunction with sFlt-1 levels (356,357). Again, the analogy to cancer patients treated with anti-VEGF therapy is informative; proteinuria induced by these medications often resolves after cessation of the drug.
Figure 13–3 Glomerular endotheliosis and normal glomerulus seen on light microscopy. (A) Glomerular endotheliosis. (B) A normal glomerulus. (From Venkatachatam MA, Kriz W. Anatomy. In: Heptinstall RH, ed. Pathology of the Kidney. 4th ed. Wolters Kluwer ¿ 1992.)
Further studies have gone on to expand our knowledge of preeclamptic proteinuria. Garovic and colleagues reported increased excretion of podocytes in the urine of women with preeclampsia (358). They also reported decreased glomerular expression of nephrin and synaptopodin in kidney tissue from autopsies of women with preeclampsia (359). These observations have been confirmed, and others have also found increased serum and urinary nephrin levels, and increased urinary excretion of podocalyxin in preeclamptic women compared to normal pregnant women (360,361). Thus, a plausible scenario emerges whereby elevated levels of sFlt-1, released by an underperfused placenta, lead to VEGF depletion, which then contributes to alterations in the glomerular filtration barrier, including disruption of glomerular endothelial cells and podocyte injury/depletion. Podocyte shedding in the urine may be the marker for the latter phase of injury.
Additional renal manifestations of preeclampsia include elevated serum uric acid >5.5 mg/dL (327 mmol/L) presumably because of decreased renal clearance. Indeed, the fractional clearance of uric acid decreases, often preceding the appearance of overt disease (362). Marked hypocalciuria has been consistently observed and is likely caused by mildly reduced GFR and enhanced tubular reabsorption. Serum-ionized calcium is normal or slightly decreased. PTH concentration is elevated, although reports are conflicting. Serum 1,25-D is decreased probably secondary to reduced placental and/or renal production. This leads to reduced intestinal calcium reabsorption and a state of calcium deficiency, thereby increasing PTH and distal tubular reabsorption of calcium.
A number of cases of hyponatremia have been reported in women with preeclampsia (363–371). The mechanism of decreased free water excretion in preeclampsia has not been clarified; however, reports of increased copeptin levels may be relevant (138,372,373).
Multisystem Pathophysiologic Alterations in Preeclampsia
Preeclampsia leads to multiorgan involvement with variable presentations. We will briefly describe the main extrarenal pathophysiologic features that have been associated with this syndrome.
Women with preeclampsia have an increase in CO early in pregnancy that is followed by a decreased CO in the third trimester (374–380). Peripheral vascular resistance (381) and central venous pressure (382) are increased in preeclampsia. This is associated with an increased cardiac afterload and impaired diastolic function (383,384). Peripartum heart failure can occur in this setting, although it is usually a complication of preexisting heart disease.
Eclamptic seizures can occur with modest elevations in BP. Indeed, preeclampsia is associated with an impaired cerebral blood flow autoregulation (385), which persists even after lowering systolic BP below <140 mm Hg with medication (386). Severely elevated BP is a risk factor for intracerebral hemorrhage in women with preeclampsia (324). Posterior reversible encephalopathy syndrome is often found on magnetic resonance imaging in eclampsia (387,388), especially in younger women with thrombocytopenia or proteinuria (388). Headaches precede 64% of seizures, whereas visual changes are present in only 32% of cases (389). Therefore, both symptoms should prompt rapid evaluation during pregnancy and in the postpartum period.
Management of Preeclampsia
A detailed discussion of obstetric and medical management is beyond the scope of this chapter.
Important principles include early diagnosis and close maternal and fetal surveillance with appropriately timed delivery.
Treating severe range hypertension (BP >160/110 mm Hg) with antihypertensives has been shown to decrease the risk of intracerebral hemorrhage and maternal death (302). In order to further prevent adverse pregnancy outcomes, we suggest preventing the development of severe hypertension by initiating oral antihypertensive therapy when BP reaches ≥150/95 mm Hg.
In preeclamptic women with central nervous system involvement (hypertensive encephalopathy, intracranial hemorrhage, eclampsia), intravenous antihypertensives are indicated to decrease MAP by 25% in minutes to hours and to 160/100 mm Hg or less over subsequent hours (302). The target for BP control in severe hypertension is to maintain BP in the 140 to 150/90 to 100 mm Hg range (305).
The ACOG has identified three first-line agents for the treatment of severe hypertension: intravenous labetalol, intravenous hydralazine, and oral nifedipine (305). The second-line therapy for the treatment of severe hypertension that fails to respond to first-line agents includes the consultation of a specialist and a continuous infusion of labetalol or nicardipine (305).
The decision to deliver is often very difficult to make when the fetus is preterm, especially before 34 weeks of gestation. In the early preterm period (23–34 weeks), the goal is to prolong pregnancy as much as possible with BP control and bed rest. Delaying delivery to a higher gestational age will improve fetal outcomes. Nevertheless, signs of serious maternal disease (headache, abdominal pain, or HELLP syndrome) or fetal distress are indications for delivery, and delays may lead to poor pregnancy outcomes (390). Epigastric and chest pain should trigger a thorough workup in women with preeclampsia because hepatic rupture and hepatocellular necrosis can occur in HELLP/preeclampsia. Women with HELLP syndrome are at a high risk for poor pregnancy outcomes and should be delivered emergently.
At 34 to 37 weeks of gestation, expectant management is also preferred, and delivery is delayed until ≥37 weeks (391,392) unless severe features of preeclampsia or fetal indications that would prompt delivery are present. In women who are at a gestational age of 38 weeks or more, delivery for preeclampsia is indicated.
Prevention of Preeclampsia
Aspirin, at a low dose, administered early in the first trimester, is associated with a modestly decreased risk of preeclampsia (∙20%) (393–395) and is recommended in women who are at a high risk for preeclampsia (246,396).
Calcium supplementation of 1 g or more per day may decrease the risk of preeclampsia but only in women with low dietary intake (164).
Sodium restriction, dietary protein, fish oil, oral magnesium all failed to show a reproducible and statistically significant decrease in the risk of preeclampsia in clinical trials.
Intravenous magnesium sulfate is indicated for the prevention of seizures only in women with eclampsia, severe preeclampsia, and preeclampsia requiring C-section (101).
Treatment with LMWH has been investigated for the prevention of preeclampsia in high-risk groups but was not of clear benefit (397–400). However, women with a genetic or acquired thrombophilias (factor V Leiden, prothrombin gene G20210A, lupus anticoagulant) may benefit from LMWH therapy in pregnancy (401,402). Interestingly, a Cochrane review in 2014 failed to see a benefit for LMWH in women with a prior history of recurrent miscarriage whether or not they had an underlying thrombophilia (403). At this time, the clinical trial evidence is inconclusive, benefits of LMWH are only apparent in certain subgroups or case series, and data from larger randomized clinical trials are needed for confirmation (404).
Chronic Hypertension in Pregnancy
Pathophysiology and Clinical Manifestations of Chronic Hypertension in Pregnancy
Chronic hypertension is defined as hypertension that is diagnosed before pregnancy, or prior to 20 weeks of gestation (101). The estimated prevalence is 3.6% to 9.1% (405). The majority (89%) of women with chronic hypertension, however, have essential hypertension (406); women with chronic hypertension should undergo a workup for potential secondary causes if hypertension is severe, or if there are renal or electrolyte abnormalities, preferably before conception.
Chronic hypertension in pregnancy is associated with higher rates of maternal and perinatal morbidity and mortality. This has been confirmed in a number of recent large, population-based studies (406,407). However, these and other reports have not consistently distinguished between women with preeclampsia superimposed on chronic hypertension and pregnant women with uncomplicated chronic hypertension alone (408–411). Although there is little doubt that women with superimposed preeclampsia have higher rates of adverse maternal and fetal/neonatal outcomes, the independent risks associated with uncomplicated chronic hypertension are less clear. Chronic hypertension is also associated with increased risk of gestational diabetes (odds ratio 1.8, 95% confidence interval 1.4–2.0). This may reflect common risk factors for both conditions such as obesity as well as similar pathogenic mechanisms, e.g., insulin resistance (410).
Placental abruption, which is associated with life-threatening maternal hemorrhage, is estimated to be threefold higher in women with chronic hypertension, although most of this risk is associated with superimposed preeclampsia (408,412,413). Other studies have not demonstrated an increased risk of abruption in women with chronic hypertension without superimposed preeclampsia (411,414,415). Differences in sample size and study population may account for the varying results.
Other adverse maternal outcomes include accelerated hypertension during pregnancy with resultant target organ damage, e.g., kidneys, heart, and brain, although in the absence of superimposed preeclampsia, this is extremely uncommon. One exception may be women with severe hypertension prior to conception, many of whom have underlying renal disease or secondary hypertension. Some women with secondary forms of hypertension, such as chronic renal disease and collagen disorders, may suffer from irreversible deterioration in renal function during and after pregnancy. In the case of SLE, there may be multiorgan morbidity, regardless of the development of superimposed preeclampsia.
Finally, although the expectation is that pregnancies in women with uncomplicated chronic hypertension will be successful, these women are more likely to be hospitalized for hypertension (414).
Perinatal mortality is also higher in pregnancies complicated by chronic hypertension, with most of this increased risk attributable to superimposed preeclampsia and fetal growth restriction (408,409,414).
Chronic Hypertension with Superimposed Preeclampsia
Superimposed preeclampsia is likely when any of the following are present (101):
• A sudden increase in BP that was previously well controlled or escalation of antihypertensive medications to control BP.
• New onset of proteinuria or sudden increase in proteinuria in a woman with known proteinuria before or early in pregnancy.
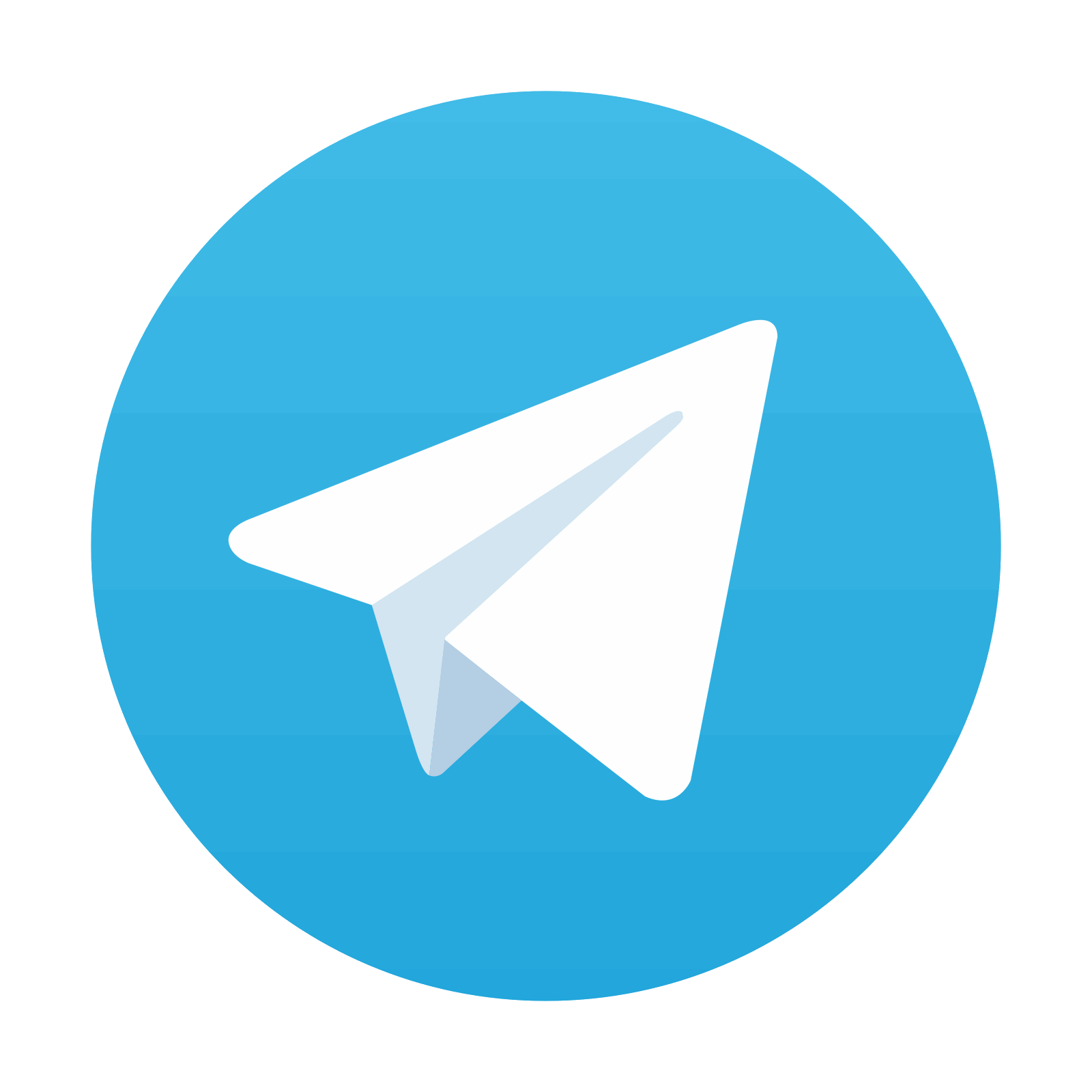
Stay updated, free articles. Join our Telegram channel

Full access? Get Clinical Tree
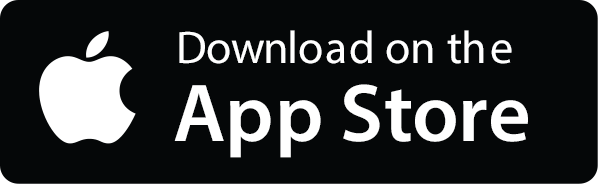
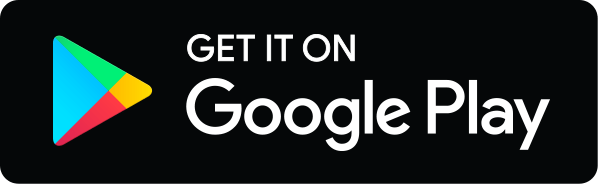