Outline
Introduction 19
Methodology for Organogenesis 20
Renal Organogenesis 23
Isotransplantation/Allotransplantation of Renal Primordia 23
Preservation Prior to Transplantation 26
Xenotransplantation of Renal Primordia 29
Alternatives to the Use of Embryonic Kidney Primordia to Achieve Organogenesis 29
Use of Embryonic Stem Cells as Starting Material 29
Use of Renal Precursor Cell Components 31
Use of Non-renal Precursor Cells Integrated into Renal Primordia 31
Use of Scaffolds 31
Current Challenges in the Application of Renal Organogenesis Technology 31
Achieving Adequacy of Blood Flow to Transplants 31
Achieving Adequate Excretion of Urine: Difficulties with Ureteroureterostomy/Ureteroneocystectomy 32
Achieving Adequate Growth of Transplanted Renal Primordia 32
Conclusion 33
Acknowledgment 33
Dialysis and allotransplantation of human kidneys represent effective therapies to replace kidney function, but the former replaces only a small component of renal function and the latter is limited by a lack of organ availability. Xenotransplantation of whole kidneys from non-primate donors is complicated by humoral and cellular rejection. The use of individual cells or groups of cells to repair damaged tissue (cell therapies) offers an alternative for renal tissue replacement. However, recapitulation of complex functions such as glomerular filtration, reabsorption and secretion of solutes that are dependent on a three-dimensionally integrated kidney structure are beyond the scope of most cellular replacement therapies. The use of non-vascularized embryonic renal primordia for transplantation circumvents humoral rejection of xenogeneic tissue and ameliorates cellular rejection. Renal primordia are preprogrammed to attract a vasculature and differentiate into a kidney, and in this manner undergo “organogenesis” after transplantation into the mesentery of hosts. This chapter reviews progress in renal organogenesis.
Introduction
Dialysis and kidney transplantation are mature technologies. They were developed during the first half of the twentieth century and came into wide use during the latter decades of the century . Reflecting on the first year of his nephrology training at Washington University in 1958, Peter A.F. Morrin notes, “The common problems of nephrology were essentially the same as they are today, but our knowledge of the underlying pathophysiology and our ability to deal with them were significantly less. Dialysis for acute renal failure was considered an experimental therapy and limited to a few centers with special interests in the area. There was no effective treatment for chronic renal failure” .
Dialysis is life-preserving, but replaces only a small fraction of normal kidney function and has a considerable morbidity . Transplantation is limited by the number of human organs available . While it is certain that dialysis and transplantation will continue to be employed during the foreseeable future, it is likely that one or more alternatives currently under development will eventually supplant their use.
The use of individual cells including stem cells or differentiated cells to regenerate or repair damaged kidney tissue (cell therapies) offers an alternative to whole organ replacement . Since cells are non-vascularized, these approaches can circumvent humoral rejection of xenogeneic tissue mediated by preformed antibodies directed against donor endothelial antigens. Furthermore, cellular rejection can be ameliorated if embryonic cells are transplanted . However, cell transplantation to replace the function of structurally complex organs such as the kidney has limitations.
Formation of a functional kidney is dependent on the growth and differentiation of its precursor cells within the intermediate mesoderm into a mature organ consisting of many different cell types. Al Awqati and Oliver have estimated that there are at least 26 terminally differentiated cell types in the kidney of a newborn mouse that arise from at least four cell types present in the undifferentiated metanephric blastema when renal development begins. Delineation of 26 terminally differentiated nephron cell types takes into account cell morphology, location and function . In order for glomerular filtration, reabsorption and secretion of fluid and electrolytes to take place in a manner that will sustain life, individual nephrons must be integrated in three dimensions with one another and with a collecting system, the origin of which is yet another separate structure, the ureteric bud . Concomitantly, vascularization must occur in a unique organ-specific manner from endothelial precursors that may originate from both inside and outside the developing renal primordium .
While it is conceivable that endocrine functions of the kidney, such as erythropoietin production, could be recapitulated by transplanting one particular type of renal cell, and it is possible that replacement of one or another type of injured renal cell could enhance the function of damaged tubules, it is difficult to imagine how glomerular filtration and reabsorption in kidneys could be reconstituted de novo by infusion of individual cells. Similarly, programming embryonic stem cells (ESCs) or stem cells from other sources to generate an organ as complex as a kidney presents a formidable and thus far unanswered challenge.
Early during its development, the renal metanephric primordium is avascular, making it a suitable cell transplant . Renal primordia are programmed to develop into a kidney in a way that ESCs are not. Therefore, one possible approach to replacing complex kidney functions is through renal organogenesis, or the growing of kidneys in situ originating from transplanted renal primordia/anlagen . This chapter will review the development of methodology for kidney organogenesis and then focus on its application for renal replacement. It will describe alternatives to the use of embryonic kidney primordia as starting material to achieve organogenesis. It will outline current challenges in the use of renal organogenesis technology and conclude by listing a body of experimentally derived general principles.
Methodology for Organogenesis
The methodology for studies directed towards renal organogenesis derives from a literature describing the transplantation of embryonic kidney. Renal primordia have been transplanted successfully to the chorioallantoic membrane of developing birds , subcutaneously , into the anterior eye chamber , into the renal cortex of recipients , beneath the renal capsule and into the abdominal cavity . Most studies that employed renal subcapsular transplantation, placement into the anterior chamber of the eye and onto the chorioallantoic membrane were conducted to define the immune response to fetal kidney transplants or to delineate the means by which renal primordia are vascularized. However, information emerged from these studies leading to approaches that use transplantation to grow a replacement kidney.
Perusal of the literature provides four theoretical reasons why the use of developing metanephroi for transplantation might be advantageous relative to developed kidneys. First, if developing renal primordia are obtained at a sufficiently early stage, antigen-presenting cells (APCs) that mediate direct host recognition of alloantigen or xenoantigen are absent. Second, donor antigens such as major histocompatibility complex (MHC) class I and II may not be expressed on renal primordia. Third, the immune response to transplanted fetal renal tissue differs from that to adult tissue. Fourth, one might expect a transplanted primordium to be supplied by host blood vessels and as such be less susceptible to humoral rejection post-transplantation across a discordant xenogeneic barrier.
Antigen Presentation
In order that a host T-cell-mediated response directed towards antigens from transplanted tissue can be mounted, transplant antigens must first be presented to host T cells. Transplant antigens can be presented by APCs originating from within the donor organ (direct presentation) or by host APCs (indirect presentation). One theoretical advantage gained through the use of embryonic tissue for transplantation is that functional APCs may be absent from the transplants because APCs have yet to mature in the embryos or migrate into the tissue .
The metanephric kidneys originate in the rat on day 12.5 of a 21 day gestation period . Foglia et al. transplanted kidneys from adult rats or metanephroi from outbred Sprague–Dawley rat embryos aged embryonic day (E)15–21, beneath the renal capsule of non-immunosuppressed adult Sprague–Dawley hosts. Under these conditions adult kidney transplants undergo acute rejection within 7 days. In contrast, growth and survival of embryonic transplants was age dependent in that enlargement and differentiation in situ over 15–30 days was best for metanephroi obtained from E15 embryos and worsened progressively for those obtained on E16–21. Primordia from E15 embryos showed maturation of renal elements when examined 10 days post-transplantation without rejection, whereas those obtained on E20 had a poor architecture and dense lymphocytic infiltrate. In contrast, liver harvested on E15 transplanted beneath the renal capsule underwent little growth and prompt rejection.
Velasco and Hegre transplanted metanephroi or liver tissue from E15, E17, E18 or E19 inbred Fisher rat embryos with rat major histocompatibility complex (RT1) RT1 lvl , beneath the renal capsule of RT1-incompatible Wistar Furth adult rats (RT1 u ). All embryonic hepatic grafts were rejected within 10 days. In contrast, the degree of rejection of the metanephroi was age dependent, those from E15 embryos showing minimal or moderate rejection and those from older embryos showing more. If liver and metanephroi from E15 embryos were cotransplanted at different sites, metanephroi underwent a more severe rejection than if they were implanted without liver. APCs populate liver well before E15 in rats, but are not present in circulation until days later. It was speculated that the absence of APCs in metanephroi from E15 embryos together with their presence in liver obtained concurrently explains the differential fate of metanephroi transplanted with or without liver. Under the former conditions, but not the latter, direct presentation of donor antigens to host T cells takes place .
MHC Expression
In the mouse, metanephroi arise on day 11.5 of a 20 day gestation period . Statter et al. transplanted metanephroi originating from E14 to adult C57Bl/6 mice (H-2 b ) beneath the renal capsule of adult congenic B10.A hosts (H-2 a ). Expression of donor and host-specific class I (H2K b ) and class II (A β b ) transcripts in E14 donor tissue was low and increased progressively in renal tissue from older mice. After transplantation, surviving kidney grafts showed enhanced expression of class I and II transcripts. However, neither class I nor II protein could be detected in transplanted renal primordia.
In human embryos, the metanephric kidneys arise during the first trimester . Dekel and co-workers carried out a series of investigations in which human adult or embryonic kidney tissue was transplanted beneath the kidney capsule of immunodeficient rats [severe combined immunodeficiency (SCID/Lewis and SCID/nude chimeric rats)]. Human adult kidney fragments transplanted beneath the renal capsule of such rats survive for as long as 2 months. The architecture of the transplanted tissue and the normal structure of glomeruli are preserved. Intraperitoneal infusion post-transplantation of allogeneic human peripheral blood mononuclear cells (PBMCs) results in rejection of adult grafts.
Human fetal kidney fragments transplanted beneath the renal capsule of immunodeficient rats display rapid growth and development. Glomeruli and tubular structures are maintained for as long as 4 months post-transplantation. In contrast to the case for transplanted adult human kidney fragments, infusion of allogeneic human PBMCs into hosts results in either minimal human T-cell infiltration or infiltrates that do not result in rejection or interfere with the continued growth of the human fetal renal tissue. Fetal human kidney grafts have reduced expression of tissue human leukocyte antigen (HLA) class I and II relative to the adult grafts, consistent with reduced effectiveness in inducing an alloantigen-primed T-cell response.
Immune Response
Dekel et al. showed that transcript levels for interferon-γ and interleukin-2 in fetal human kidneys grafted under the renal capsule of immunodeficient rats are markedly reduced after transplantation relative to levels in adult human kidney tissue grafted to the same site. Peak levels of these cytokines appear late after PBMC infusion. Concomitant with these findings, interleukin-4 mRNA is upregulated during the early phase post-PBMC infusion, and interleukin-10 mRNA is expressed throughout the post-PBMC infusion interval. In addition, levels of mRNA coding for chemokines [regulated on activation, normal T cell expressed and secreted (RANTES) and macrophage inflammatory protein-1β (MIP-1β)], their receptor, CCR5, and the cytolytic effector molecule, Fas ligand, are suppressed in the fetal grafts relative to levels in adult grafts. Thus, fetal kidney induces the downregulation of Th1 cytokines, chemokines and Fas ligand, and the upregulation of Th2 cytokines in the grafts. The findings suggest that the human immune response of kidney rejection is dependent on whether the target organ is of fetal or adult origin. An allogeneic immune system appears to mount a T-helper-2-biased response when the target organ is fetal, resulting in enhanced survival of transplanted tissue relative to adult tissue against which a T-helper-1-biased response is mounted.
Subsequently, Dekel et al. showed that developing human kidneys had restricted expression of multiple factors that determine immune recognition. Thirteen out of 57 genes that were significantly upregulated in adult versus fetal human kidney tissue belonged to the HLA class I and II systems. In addition, molecules that mediate trafficking of leukocytes into the graft such as chemokines RANTES and MCP-1, adhesion molecule E-selectin, proinflammatory cytokines such as osteopontin and complement genes had reduced expressions in embryonic relative to adult kidneys. Reduced immunogenicity of embryonic human or pig kidneys transplanted into immunodeficient mice was confirmed by the absence of cellular rejection following infusion of human PBMCs .
Vascularization
The major arterial vessels supplying the kidney originate from lateral branches of the abdominal aorta that terminates in a plexus of arteries in close proximity to the renal pelvis, the renal artery rete . It is a matter of controversy whether the renal microvasculature (smaller vessels and glomerular capillaries) arises exclusively via this angiogenic process, or also in part from endothelial cells resident in the developing metanephros. However, it is clear that during its development, the renal primordium is able to attract at least its major arterial vessels from the developing aorta . In that its blood supply originates at least in part from outside of the developing renal primordium, the kidney may be regarded as a chimeric organ. Its ability to attract its own vasculature in situ establishes the renal primordia as cellular transplants, capable of attracting a blood supply from an appropriate vascular bed .
Insight into the origin of the renal microvasculature supply is provided by experiments in which developing kidneys are transplanted to ectopic sites. However, the results of these experiments are somewhat contradictory. One explanation for the differences may be that the means of vascularization is site specific. For mouse or chick metanephroi obtained from E11.5 embryos grafted onto the chorioallantoic membrane of the quail, the vasculature is derived entirely from the host . In the case of metanephroi from E11–12 mouse embryos grafted into the anterior chamber of the eye in genetically identical mice, the glomerular endothelium derives from both donor and host . For metanephroi from E15 rat embryos transplanted into the abdominal cavity of mice , or from E28 pig embryos transplanted into the abdominal cavity of rats or mice , the microvasculature is largely or entirely host. In all cases, large external vessels derive from the host.
Host immune responses directed against antigens located on the endothelium of a transplanted vascularized organ such as a kidney, or mediated by transplant endothelial cells, are reduced in proportion to the extent that an organ can be transplanted in cell form and be supplied by host vessels as it develops in situ. Such reduction would be beneficial in the case of allotransplantation. However, for xenotransplantation it could provide a way to ameliorate humoral rejection (hyperacute rejection and acute vascular rejection) that represents an obstacle to the use of non-primate vascularized organs, such as kidneys from adult pigs, for transplantation into humans .
Humans and pigs are of comparable size and share a similar renal physiology. The maximum concentrating ability (1080 mOsm/l) and glomerular filtration rate (GFR; 126–175 ml/h or 5 ml/min/kg) of porcine kidneys are similar to those of human kidneys (1200 mOsm/l and 120–125 ml/h or 4 ml/min/kg, respectively) . The specific gravity of pig urine is 1.01–1.05 and the pH 5.5–7.7 (mean 6.40), while those of human urine are 1.002–1.028 and 4.6–8.0, respectively . Physiological differences do exist in renal function between humans and pigs. For example, in adult swine, the normal level of proteinuria is 6–20 mg/100 ml . However, experimental observations in the pig-to-primate model have shown that plasma urea, sodium, chloride and potassium remain within normal limits in hosts with good long-term renal function . In view of this level of physiological compatibility between swine and non-human primate renal function, and because pigs are plentiful and can be bred to be pathogen free, pigs represent a suitable kidney donor for humans .
Hyperacute rejection occurs as a result of the binding of preformed or natural xenoreactive antibodies present in the circulation of hosts to cells of the donor species followed by activation of the host’s complement system. Approximately 85% of the natural antibodies in humans that bind to pig cells are directed against galactose-α-1,3-galactose (alpha-gal), a sugar expressed on the vascular endothelium of cells in most mammals, but not in humans, great apes and old-world monkeys. The etiology of acute vascular rejection is multifactorial and incompletely understood. Several of the processes implicated as causative reflect a fundamental incompatibility between host proteins/protein systems and the vascular endothelium of the donor. Factors that are thought to contribute include circulating xenoreactive antibodies that trigger adverse reactions in transplant endothelium, the failure of primate natural killer cells to recognize porcine MHC I molecules, and incompatibilities between porcine proteins/receptors and circulating primate/human proteins such as clotting factors .
Humoral rejection following the transplantation of pig kidneys into non-human primates can be ameliorated or overcome through the use of genetically altered organs originating from pigs transgenic for the human complement activator, decay accelerating factor (hDAF) , or the use of organs from transgenics that do not express alpha-gal . Unfortunately, neither the immunosuppressive regimens used for transgenic-pig to primate kidney transplantation nor the outcomes would be acceptable in humans. Transplantation of kidneys from pigs transgenic for hDAF in combination with host immunosuppression and splenectomy enabled survival for up to 78 days in otherwise anephric cynomolgous monkeys. However, there was a high incidence of adverse events such as development of edema, ascites, vomiting, diarrhea or lymphoproliferative disorders. All recipients had to be euthanized because of renal failure, gastrointestinal hemorrhage or pancreatitis . Survival for as long as 83 days was achieved post-transplantation of kidneys from alpha-gal-deficient donors into immunosuppressed thymectomized and splenectomized baboons, but only if vascularized pig thymic tissue was cotransplanted. Baboons died from serum sickness, infection or myocardial infarction .
Renal Organogenesis
In contrast to xenotransplantation of whole vascularized organs from pig to primates, cell transplants such as pancreatic islets from pigs can be transplanted into humans without triggering hyperacute or acute vascular rejection . As delineated above, the isolated renal metanephric primordium is a cell transplant. It had been speculated that developing nephrons implanted beneath the renal capsule or into tunnels fashioned in the cortices of host kidneys may become incorporated into the collecting system of the host, and thereby increase host renal function. Woolf et al. implanted pieces of sectioned renal primordia originating from embryonic day E13–16 mice into tunnels fashioned in the cortex of kidneys of newborn outbred mice. Differentiation and growth of donor nephrons occurred in the host kidney. Glomeruli were vascularized, mature proximal tubules were formed and extension of metanephric tubules into the renal medulla was observed. However, incorporation of donor nephrons into the collecting system of hosts was not demonstrated.
The present author’s group performed experiments similar to those of Woolf et al. in which metanephroi from E15 Sprague–Dawley rat embryos were implanted beneath the renal capsule of adult Sprague–Dawley hosts. Hosts received no immunosuppression. E15 renal primordia contained segments of ureteric bud and condensing metanephric blastema, but no glomeruli . To determine whether subcapsularly transplanted rat renal primordia became integrated into host kidneys, kidneys of host rats were examined 6 weeks after subcapsular transplantation. To clear blood from the organ, kidneys were back-perfused. This results in a blanching of the kidney as blood is replaced by perfusate. Normally, the entire kidney blanches. However, following perfusion of kidneys that contained a transplanted renal primordia, blood remained in the transplanted structure relative to the host kidney ( Fig. 2.1 A, cortex arrows). This is likely to reflect a reduced perfusion in chimeric blood vessels (derived from transplant and host kidneys) that have been shown to supply subrenal capsularly transplanted primordia relative to perfusion in those supplying only the host kidney. Blood could be traced into the papilla of the host kidney ( Fig. 2.1 A, medulla, arrows). Histological examination of kidneys showed that glomeruli (g) in the transplanted kidney ( Fig. 2.1 B) had been poorly perfused relative to glomeruli present in the host kidney ( Fig. 2.1 C), in that they contained more red blood cells. Also, glomeruli in the transplanted kidneys (g) were smaller than those in the host kidney (G).

Collecting ducts from transplanted renal primordia migrated towards the papilla of host kidneys in parallel with the vasculature . However, like Woolf et al. , this study was unable to determine that any connection between the collecting systems of donor and host kidneys was made. In addition, the growth of transplanted renal primordia was constrained by their placement beneath the host kidney capsule.
Isotransplantation/Allotransplantation of Renal Primordia
Renal primordia transplanted into a host rat’s fold of mesentery undergo differentiation and growth in hosts that is not confined by a tight organ capsule . A renal primordium in a retroperitoneal dissection from an E15 rat embryo is shown in Fig. 2.2 (a). The ureteric bud is delineated by an arrowhead. If transplanted to mesentery with its ureteric bud attached ( Fig. 2.2 , center panel), the renal primordium enlarges and becomes kidney shaped within 3 weeks ( Fig. 2.2 b). The ureteric bud differentiates into a ureter ( Fig. 2.2 b, arrowhead). In contrast to transplanted developed kidneys that undergo acute rejection , renal primordia transplanted into non-immunosuppressed hosts have a normal kidney ultrastructure postdevelopment in situ and become vascularized via arteries that originate from the superior mesenteric artery of hosts and veins that originate from the host omentum . Figure 2.3 (a) shows a radiocontrast study that demonstrates that the transplanted metanephros (transplant) is supplied by the host’s superior mesenteric artery (SMA). Figure 2.3 (b) shows a renal primordium or metanephros (m), 3 weeks after allotransplantation. An artery (a) and vein (v) originating from the host are delineated. A ureteroureterostomy (arrow) between the ureter originating from the transplanted renal primordium (m) and the host ureter is shown in Fig. 2.3 (c).

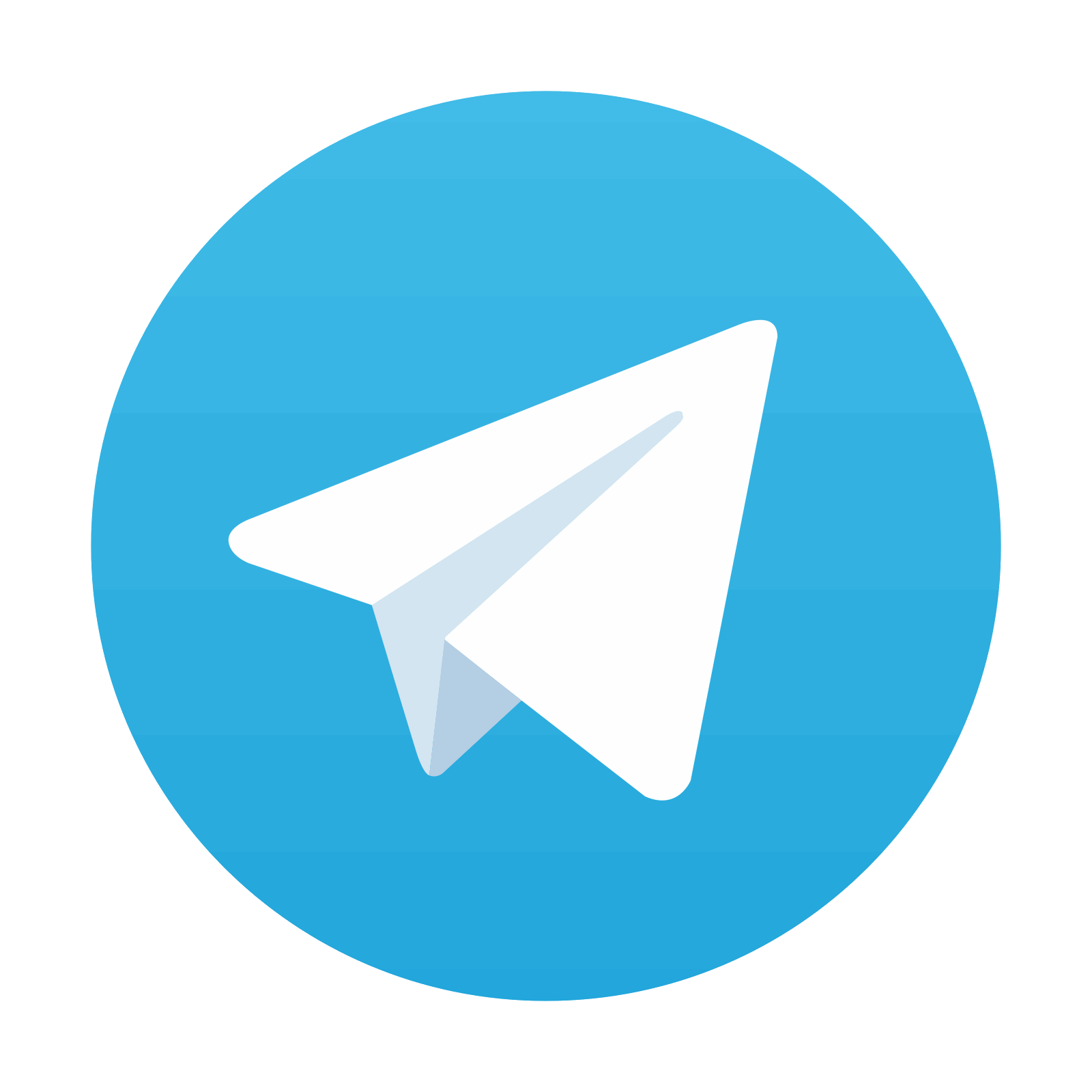
Stay updated, free articles. Join our Telegram channel

Full access? Get Clinical Tree
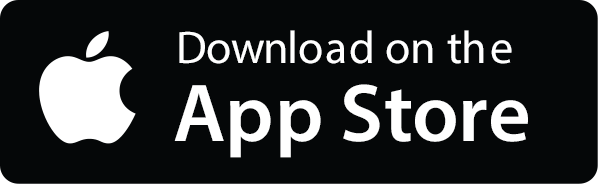
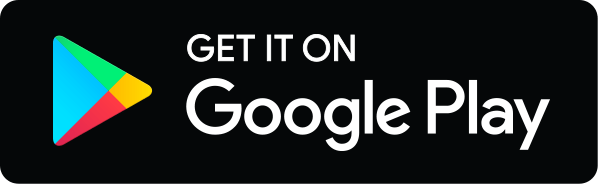
