Obesity-related dyspnea
Sleep-disordered breathing
Snoring
Upper airway resistance syndrome
Obstructive sleep apnea (OSA)
Obesity hypoventilation syndrome
Obesity-related respiratory failure
Severe OSA with associated hypercapnia
Lone obesity hypoventilation syndrome (OHS)
Combined OHS and OSA
Asthma
Increased severity of symptoms
Possible role in etiology
Chronic obstructive pulmonary disease (COPD)
Decreased mortality
Overlap syndrome (COPD and OSA)
Anesthesia-related complication
Difficult bag and mask ventilation
Difficult endotracheal intubation
Perioperative atelectasis and hypoxemia
13.2 Role of Fat Distribution
Studies have shown that the effects of obesity on the respiratory system are influenced more by the distribution of fat rather than the absolute value of weight or BMI. Central obesity (upper body fat distribution) is more common in males and is caused by deposition of adipose tissue in the abdomen, anterior abdominal wall, and anterior chest wall while peripheral obesity (lower body fat distribution) is associated with excess subcutaneous fat. The two can be distinguished by waist circumference to hip ratio (WHR) with ratios greater than 0.95 being indicative of central obesity. Subscapular and biceps skinfold thickness can also be used as indirect measures of upper body fat distribution [5]. Higher values of WHR and abdominal height correlate well with decline in lung function [6–8]. Central obesity increases the resistive load on the respiratory muscles and decreases diaphragmatic excursion, thereby, limiting expansion of basal lung units and premature closure of peripheral lung airways. In contrast, peripheral obesity is associated with minimal compromise of lung function and, thereby, less obesity-related complications.
13.3 Effect of Obesity on Pulmonary Mechanics
Obesity affects virtually all parameters of lung function testing. Obese patients have lower lung compliance, chest wall compliance, and total respiratory compliance [9, 10]. Among the static lung volumes, a consistent relationship has been established with increase in BMI and reduction in expiratory reserve volume (ERV) and hence the functional residual capacity (FRC) [11] (Fig. 13.1). Weight loading of the thoracic chest wall causes normal tidal respiration to occur at lower levels of FRC. Intra-abdominal fat deposition decreases diaphragmatic movements resulting in micro-atelectasis and early closure of small airways in the basal juxta-diaphragmatic lung units and, therefore, volume loss and reduction in FRC and ERV. Figure 13.1 illustrates the effect of obesity on static lung volumes. Among the dynamic lung volumes, forced expiratory volume in first second (FEV1) and forced vital capacity (FVC) are generally well preserved until extreme elevations in BMI [12]. Excessively obese patients tend to have low FEV1 and FVC with FEV1/FVC ratio being normal (>70 %) suggestive of a restrictive pattern [13].


Fig. 13.1
Effect of obesity on lung volumes: Expiratory reserve volume (ERV) decreases in obesity. The functional residual capacity (FRC), sum of ERV and residual volume, is reduced, often approaching residual volume (see arrow). The decline in FRC in obese subjects is primarily the result of reduced ERV. Compensatory increase in inspiratory reserve volume may occur so that the total lung capacity (TLC, the sum of FRC and inspiratory capacity or IC) is often normal (Reproduced with permission from Sood [11])
Airway resistance is higher in obese individuals compared to normal controls. This can be explained in part by the low lung volumes at which normal tidal respiration occurs in these patients leading to closure of small airways [14]. Studies have shown that corrected for lung volume, the airway conductance (inverse of airway resistance) is normal in obese subjects [15]. A positive correlation between airway conductance and FRC has been established; however, there is no conclusive evidence to support a relation between absolute BMI and airway conductance [16]. Inflammatory cytokines (adipokines) released by adipose tissue have also been hypothesized to contribute to airway inflammation and increased airway resistance [17].
Obese individuals generally have normal arterial oxygen pressure (PaO2), but alveolar-arterial oxygen gradient [P(A-a)O2] is often widened, especially in morbidly obese subjects who may also have hypoxemia. This is explained by ventilation-perfusion (V/Q) mismatch secondary to premature closure of airways in dependent portions of the lungs which have good perfusion but are underventilated [18]. These changes are more marked in the supine position while P(A-a)O2 gradient and PaO2 improve during exercise which is explained by recruitment of the collapsed basal units [19]. Recent studies have shown a correlation between WHR and changes in PaO2, PaCO2, and P(A-a)O2 gradient [20]. Gas exchange is generally normal in obese subjects with some studies showing that DLco when corrected for lung volume in extremely obese (height/weight ratio > 1.10) may actually be elevated [21].
Obese individuals have higher basal oxygen consumption which can be attributed to the increased work of breathing caused by higher resistive loads of the anterior chest wall, overstretching of the diaphragm resulting in lower efficiency, and increased basal body requirement when compared with lean subjects [22]. Therefore, resting minute ventilation is higher in obese subjects, at the expense of higher respiratory rates as obese patients cannot significantly increase tidal volume [22, 23]. Minute ventilation increases during exercise, and the increase is more marked as compared to normal subjects which can be explained by the recruitment of basal atelectatic lung units during exercise [19].
The effects of obesity on pulmonary physiology are summarized in Table 13.2.
Table 13.2
Altered resting respiratory physiology in obesity
Physiologic parameter | Effect of obesity |
---|---|
Respiratory compliance | Decreased |
Respiratory muscle strength | Decreased |
Work of breathing at rest | Increased |
Vital capacity (VC) | Normal or decreased |
Forced expiratory volume in one second (FEV1) | Normal or decreased |
Ratio (FEV1/VC) | Normal, increased, or decreased |
Maximal expiratory flow rates at low lung volumes | Decreased |
Expiratory reserve volume (ERV) | Decreased |
Residual volume (RV) | Normal |
Inspiratory capacity (IC) | Normal or increased |
Total lung capacity | Normal or slightly decreased |
Airway resistance | Decreased |
Specific airway conductance | Normal |
Diffusion capacity | Variable |
Alveolar-arterial oxygen tension gradient [P(A-a)O2] | Decreased |
13.4 Obesity and Dyspnea
Overweight and obese subjects are more likely to have self-reported dyspnea at rest and exertion compared with lean individuals even in the absence of an underlying pulmonary disease [24, 25]. Prior studies have suggested that the increased perception of dyspnea in obese patient may be related to increased work of breathing and lower efficiency of respiratory muscles [24, 26]. Weight loss can reverse these symptoms; however, it still remains to be explained why only some obese subjects complain of exertional dyspnea [25].
13.5 Obesity and Sleep-Disordered Breathing
Sleep–disordered breathing (SDB) refers to a set of conditions associated with narrowing of the upper airways. As the upper airway resistance increases, the severity of SDB increases. The least severe presentation is in the form of simple snoring during sleep without sleep fragmentation and daytime somnolence. At the other end of the spectrum is obstructive sleep apnea (OSA) wherein patients have episodes of apnea and/or hypopnea followed by arousal from sleep and, therefore, sleep fragmentation leading to excessive daytime somnolence. The presentation of patients with upper airway resistance syndrome (UARS) is intermediate of normal subjects and those with OSA as characterized by sleep fragmentation and daytime somnolence but without the typical apneic and hypopneic episodes of OSA. Epidemiological studies have shown obesity to be a major risk factor for OSA, and nearly 50 % of patients with this syndrome have a BMI > 30 kg/m2. Further, in obese subjects, the risk of developing OSA increases with rising BMI, and morbidly obese individuals have 55–90 % risk of developing OSA [27]. In newer studies, neck circumference has been demonstrated to correlate better with risk of OSA than absolute BMI while abdominal circumference may be a superior predictor than both neck circumference and BMI [28, 29].
Obesity predisposes to development of SDB by increasing collapsibility of the upper airway (UA). The airway passes through a partly closed maxillomandibular bony enclosure, and increased deposition of adipose tissue in this region causes narrowing of the pharyngeal airway, resulting in an increase in critical pressure (P crit) which is defined as the pressure inside the airway at which airway collapse occurs. As P crit increases, severity of OSA increases and effective therapy of OSA requires application of continuous positive airway pressure (CPAP) higher than P crit. Obese persons also have higher basal oxygen consumption and consequently greater fall in oxygen saturation during apneas/hypopneas as compared to nonobese patients with OSA of same severity [30]. Further, as OSA causes fatigue and increased daytime somnolence, the level of physical activity is likely to be lower making weight loss difficult. Studies have also shown that decreased sleep at night increases daytime intake of carbohydrates thereby promoting obesity [31]. Hence, the relationship between OSA and obesity is bidirectional, setting up a vicious cycle. Consequences of SDB can result either from sleep fragmentation and excessive daytime sleepiness or from nocturnal hypoxemia/hypercapnia during apneic and hypopneic episodes. Table 13.3 summarizes the consequences of SDB.
Table 13.3
Consequences of obstructive sleep apnea
Cardiovascular |
Hypertension |
Coronary artery disease |
Nocturnal angina |
Myocardial infarction |
Heart failure |
Arrhythmias especially bradyarrhythmias |
Pulmonary hypertension |
Chronic cor pulmonale |
Sudden cardiac death |
Neurological and neuropsychiatric |
Morning headache |
Refractory epilepsy |
Stroke |
Anxiety |
Depression |
Acute delirium |
Endocrine |
Insulin resistance |
Diabetes mellitus |
Impotence and erectile dysfunction |
Metabolic syndrome |
Automobile accidents |
Miscellaneous |
Hoarse voice |
Nocturia |
Gastroesophageal reflux disease |
Polycythemia |
The clinical features of SDB depend on the severity of upper airway obstruction. Patients with OSA commonly present with complaints of snoring, apneas witnessed by partners, fatigue, chronic sleep deprivation, excessive daytime sleepiness, and morning headaches. Diagnosis of obstructive sleep apnea can only be confirmed by overnight multichannel polysomnography (PSG) which includes electrocardiogram, electroencephalogram for staging sleep, electrooculogram for detecting rapid eye movements, chin electromyogram to measure muscle tone, respiratory inductance plethysmography to measure respiratory muscle effort, nasal airflow sensors, and pulse oximetry for recording arterial oxygen saturation. Apnea is defined as reduction in airflow by 90 % or more for at least 10 s and hypopnea is defined as 30–90 % reduction in airflow for a minimum of 10 s with either a reduction in baseline oxygen saturation by 3 % or an event-associated arousal from sleep [32]. Apnea-hypopnea index (AHI) measures the average number of obstructive sleep events (apnea and hypopnea) per hour of sleep time and AHI ≥ 5 events/h in the presence of symptoms, or AHI ≥ 15 events/h in the absence of symptoms is diagnostic of OSA. Severity of OSA is also graded based on AHI: ≥ 5 to 15 events/h – mild OSA, ≥ 15-30 events/h – moderate OSA and > 30 events/h – severe OSA. Obstructive sleep apnea syndrome (OSAS) is classically defined as OSA (diagnosed by polysomnography) with excessive daytime sleepiness (EDS). Epworth Sleepiness Scale (ESS) is a subjective test, and a score greater than 10 out of 24 denotes EDS. Objective tests include multiple sleep latency test or maintenance of wakefulness test but are seldom needed. EDS is not synonymous with OSA and also associated with several other conditions. However, all patients with unexplained EDS should be tested for OSA.
PSG has critical limitations in being expensive, requiring specially trained personnel, and a well-equipped sleep laboratory. In patients without major medical complications such as heart failure, coronary artery disease, and respiratory failure, home testing using portable monitors that measure oxygen saturation and airflow has been validated and is equally reliable for diagnosis and monitoring response to treatment [33].
Sleep apnea, even when asymptomatic, confers an increased risk of associated medical complications, most importantly cardiovascular diseases [34]. Although the literature has reported conflicting results, snoring alone has been associated with hypertension, angina, and insulin resistance [35, 36]. Continuous positive airway pressure (CPAP) at night acts as a pneumatic splint, preventing airway collapse, and is the most beneficial treatment modality reducing both the AHI and risk of complications. The pressure requirement is calculated by CPAP titration during polysomnography, and modern machines have facility of auto-CPAP which detects changing obstruction and makes automatic adjustments to CPAP. Bi–level positive airway pressure (BPAP) machines use higher pressures during inspiration compared with expiration, and this therapy is indicated in patients with high PAP titration pressures (>13–14 cm H2O), type II respiratory failure, and coexistent obesity hypoventilation syndrome. PAP therapy for OSA is provided with room air except for complications such as pulmonary hypertension and respiratory failure when supplemental oxygen therapy or formal noninvasive positive pressure ventilation (NIPPV) is needed. The choice of delivery system depends upon patient comfort and includes full face masks, oral masks, nasal masks, and nasal pillows. Nasal masks have greater tolerance and are generally preferred as the first choice. However, the drawback of PAP is discomfort to the patient from the delivery system and difficulty in daily adherence. Surgical procedures like uvulopalatopharyngoplasty may benefit patients with mild disease, but cure rate is reported to be less than 20 % [37]. Oral appliances including tongue repositioning devices and mandibular advancing devices can be used as primary therapy in patients with mild to moderate OSA or as adjunct to PAP in severe OSA [38]. Weight loss through lifestyle changes or bariatric surgery results in both subjective and objective (AHI) improvement in patients with OSA [39, 40].
13.6 Obesity Hypoventilation Syndrome
Obesity is central to the pathogenesis of obesity hypoventilation syndrome (OHS) which is defined by BMI ≥ 30 kg/m2, chronic daytime hypoxemia and hypercapnia (PaO2 < 70 mmHg and PaCO2 ≥ 45 mmHg), and sleep-disordered breathing (SDB) [41, 42]. Other causes of hypoventilation such as underlying pulmonary, neuromuscular, skeletal, and metabolic diseases need to ruled out [43]. The most common form of SDB in OHS is OSA, occurring in nearly 90 % of the patients [44]. The remaining 10 % of the patients have “lone” OHS with an AHI < 5 events/h. SDB in this subgroup is termed as sleep hypoventilation and is defined by an increase in PaCO2 by 10 mmHg during sleep or significant decline in oxygen saturation not explained by apnea or hypopnea [41, 43]. While majority of OHS patients have OSA, a causal relationship between the two has not been established [44]. Among patients with OSA only 10 % have features of OHS [45, 46].
As discussed previously in this chapter, obesity causes restrictive lung functions and increased basal demands. Normal obese subjects can meet these demands by increasing their respiratory drive. However, patients with OHS have greater respiratory restriction and have poor central responsiveness to hypoxemia and hypercapnia resulting in chronic hypoventilation [47]. Leptin has been shown to increase central responsiveness in obese individuals, and patients with OHS have high leptin levels indicative of leptin resistance [48]. In addition to hypoventilation, V/Q mismatch secondary to physiologic effects of obesity, as already mentioned, also contributes to the daytime hypoxemia and increased P(A-a)O2. Nonetheless, why some obese patients develop OHS and others do not is still not clearly understood.
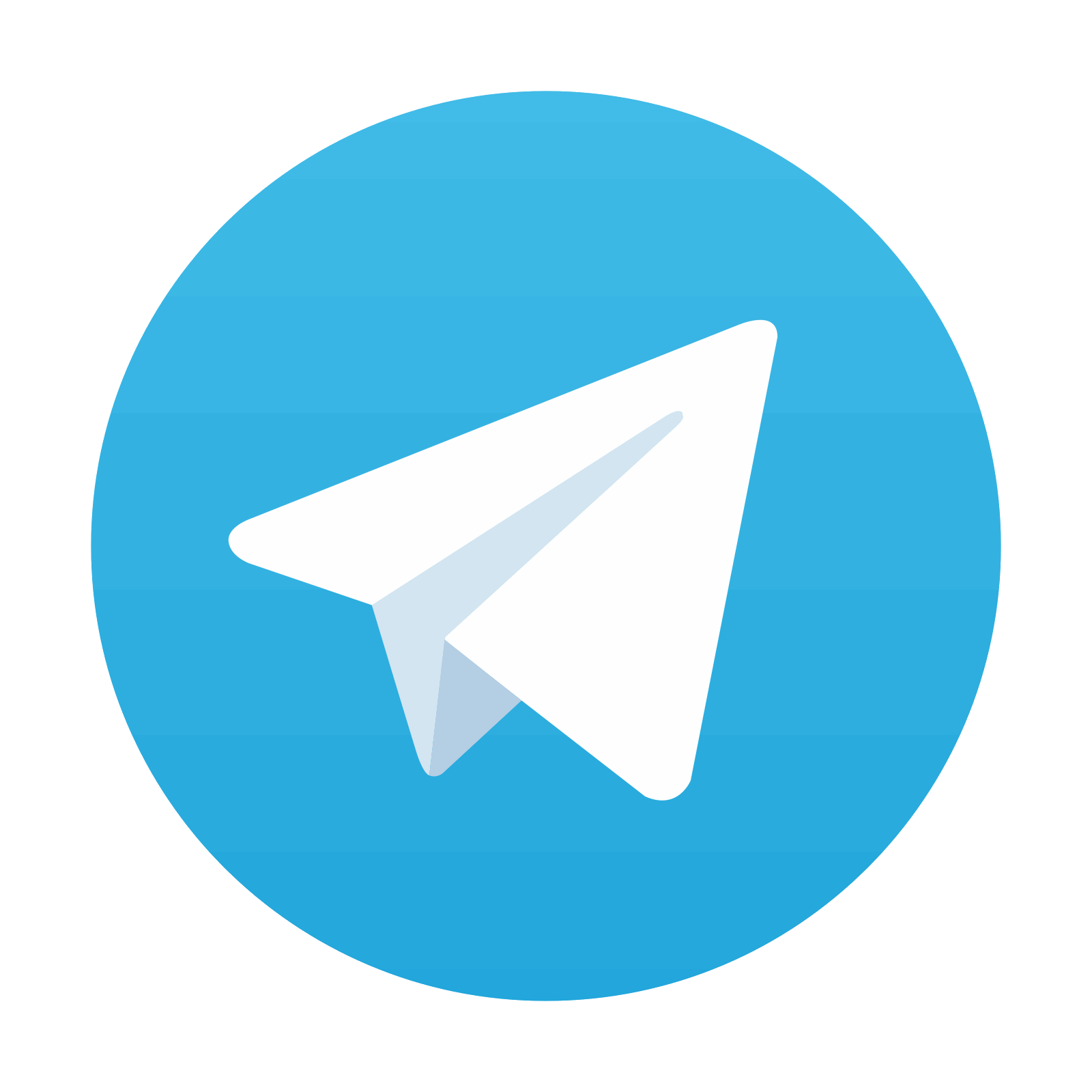
Stay updated, free articles. Join our Telegram channel

Full access? Get Clinical Tree
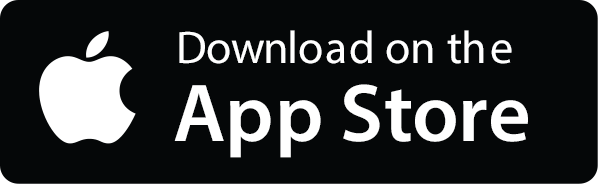
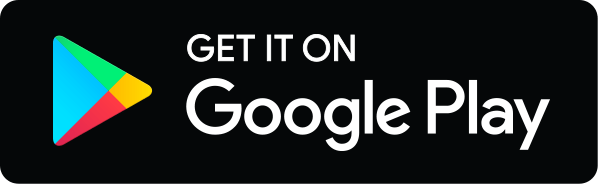