Epidemiology
Pulmonary atresia (PA) with ventricular septal defect (VSD) and major aortopulmonary collaterals (MAPCAs) is a complex lesion with great morphologic variability that represents approximately 2 percent of congenital heart defects, with a prevalence of 0.07 per 1000 live births.
Morphology
This particular malformation entails the association of atresia of the pulmonary valve, a tetralogy-type VSD, and pulmonary collateral vessels that usually originate from the descending thoracic aorta.
Clinical features
Neonates born with PA, VSD, and MAPCAs may have very unpredictable presentation owing to the anatomic variability of the lesion. Patients may be minimally symptomatic or severely cyanotic, or may develop congestive heart failure (CHF) from pulmonary overcirculation.
Diagnosis
Diagnostic evaluation includes echocardiography, cardiac catheterization, and, in selected cases, computed tomographic angiography (CTA) to clarify the anatomy of the MAPCAs.
Treatment
Definitive treatment is surgical. The ultimate goal of surgical therapy is creation of separated, in-series pulmonary and systemic circulations. Achievement of this goal requires unifocalization of the vascular systems, VSD closure, and reconstruction of the right ventricular outflow tract (RVOT). “Single-stage” unifocalization with or without concurrent VSD closure is favored by the authors.
Outcomes
In over 460 patients managed by the authors’ protocol, complete unifocalization via median sternotomy was achieved in 76 percent of patients. Intracardiac repair (VSD closure) was possible at initial operation in 56 percent. Ninety-five percent of patients were completely repaired by 5 years of age. Recent operative mortality was 2.3 percent, with a 5-year actuarial survival of 85.5 percent.
Pulmonary atresia (PA) with ventricular septal defect (VSD) and major aortopulmonary collateral arteries (MAPCAs) is a complex lesion with great morphologic variability. The lesion is characterized by atresia of the pulmonary valve, a tetralogy of Fallot-type VSD, and pulmonary collateral vessels originating from the aorta. The major variability occurs in the pulmonary vascular circulation. Development of the pulmonary arterial system occurs by fusion of the left and right sixth dorsal aortic arches with the plexus of systemic arteries carried by the lung buds from the foregut. Failure of the normal fusion process results in PA. Development of the true pulmonary arteries (PAs) is variable and depends on the point in gestation at which connection of the lumen of the true PAs with the right ventricle (RV) is lost.1 True central PAs vary from normal size to absent. The mean diameter of the central PAs in the author’s series of over 300 patients was 1.5 mm. A small central confluence is usually present. When confluent PAs are present, there is typically no ductus arteriosus. When the right and left main PAs are discontinuous, bilateral ducti may exist with the right typically originating from the innominate or subclavian artery.
Persistent connections from the aorta (MAPCAs) probably originate from splanchnic vessels. Collaterals vary widely in origin, course, size, and histologic appearance. The mean number of collaterals in the author’s series was 3.8 ± 1.4. Most commonly, aortopulmonary collaterals originate from the proximal descending aorta. There is great variability in the way in which MAPCAs connect to the pulmonary circulation. A given lung segment may have its blood supply uniquely derived from the true PA system, MAPCAs, or both; collateral vessels may supply anything from a small proportion of the total lung parenchyma to the majority of the pulmonary vascular bed. Variable degrees of obstruction of the collateral vessels may occur, and obstructions may be inconsistent in their location—proximally or distally—within the vessels. Diffuse collateralization from the systemic circulation, especially the chest wall, is common, particularly after surgical intervention.
The pulmonary microcirculation is the healthiest at birth, and declines progressively afterward. The natural history of the MAPCAs is to frequently progress to stenosis, which may in turn result in distal microvascular hypoplasia. In the absence of stenosis, the systemic pressure within the pulmonary vasculature may cause pulmonary vascular obstructive disease (PVOD). Surgical interventions requiring the use of systemic-to-PA connections (e.g., Blalock–Taussig shunt or aortopulmonary window) may also result in PVOD due to the persistence of pulmonary vascular flow at systemic pressure.
The intracardiac anatomy is typified by an anteriorly malaligned VSD (hence the alternative designation as tetralogy of Fallot with PA). Although the ventricles are typically normally developed, the infundibulum of the RV may be quite small. There are normal atrioventricular and ventriculoarterial connections (Fig. 69-1).
PA with VSD represents approximately 2 percent of congenital heart defects. In the Baltimore–Washington Infant Study, a prevalence of 0.07 per 1000 live births was reported.2 PA with VSD accounted for 1.4 percent of all forms of congenital heart disease, and 20.3 percent of all forms of tetralogy of Fallot. Of patients with PA and VSD, approximately 60 percent have a ductus arteriosus and normal branch PAs, and 40 percent have MAPCAS. Infants of overtly diabetic mothers are at approximately 10-fold risk of developing PA with VSD. Genetic factors are considered likely to be etiologically contributory, since an increased risk of occurrence to siblings (2.5–3 percent) or to children of parents with tetralogy of Fallot (1.2–8.3 percent) has been observed.
Associated cardiac and extracardiac anomalies may occur. Commonly associated cardiac anomalies include secundum atrial septal defects, coronary anomalies, and multiple VSDs. Other less common cardiac anomalies may also occur.
A significant proportion of patients with PA and VSD have associated syndromes or extracardiac anomalies. Such associations include the VATER syndrome, Alagille syndrome, DiGeorge (velocraniofacial) syndrome, and trisomy 21 among others. There may be an unusually high incidence of tracheobronchial anomalies, particularly the origin of the right upper lobe bronchus from the trachea, which may result in postoperative respiratory complications involving the right upper lobe.
Children born with PA, VSD, and MAPCAs may have very unpredictable presentation due to the anatomic variability of the lesion. Approximately 80 percent are minimally symptomatic, and have only mild cyanosis. The VSD is typically unrestrictive. Patients with valvar atresia but little collateralization may be severely cyanotic. Patients with severe atresia and/or hypoplastic true PAs but extensive collateralization may be minimally symptomatic and plethoric. Such patients may eventually develop congestive heart failure (CHF) due to pulmonary overcirculation, or may develop progressive cyanosis. Cyanosis occurs due to paucity of pulmonary flow because of inadequate collaterals, stenosis of the collaterals, or pulmonary microvascular obstructive disease. Development of these complications may occur at very variable rates, resulting in unpredictably delayed presentation. Cyanotic spells may occur. In patients with PA these occur due to decreases in systemic vascular resistance resulting in markedly decreased pulmonary blood flow [rather than due to RV infundibular spasm, as is usual in tetralogy without atresia]. Late presentation may occur3 and may include polycythemia, “clubbing,” cerebral embolism, and cerebral abscesses.
Following presentation with the symptoms described above, patients require complete diagnostic investigation. Pulse oximetry is a useful indicator of adequacy of pulmonary blood flow. Patients with oxygen saturation greater than 85 to 90 percent will typically have well-developed collateral systems and are at risk for CHF. Patients with oxygen saturations of less than 75 to 80 percent may have poorly developed pulmonary vascular and collateral systems.
Chest radiographs may demonstrate pulmonary plethora or hypoperfusion of the lung fields. The electrocardiogram is typically normal at birth but will gradually develop evidence of RVH comparable to tetralogy of Fallot. Echocardiography is highly reliable in making the diagnosis of PA, VSD, and MAPCAs, but is typically inadequate for complete evaluation. Echocardiography is sensitive for detection of aortopulmonary collaterals, but inadequate for complete delineation, which is essential for surgical management. The intracardiac anatomy is well delineated by echocardiography. Echocardiography may also show other associated cardiac lesions including ASD or a patent foramen ovale (PFO), multiple VSDs, and coronary anomalies.
Cardiac catheterization is essential in evaluation of the true PAs and aortopulmonary collateral system (Fig. 69-2). It is particularly important to clarify the complete true pulmonary arterial and aortopulmonary collateral distribution. Evaluation of the true PA system may be difficult unless there is duct-dependent pulmonary circulation or a connection between a collateral and the true PA system. Retrograde venous wedge angiography may be needed to evaluate the true PA system in the absence of a ductus or connections between collateral and true PAs. The true central PA system appears as a “seagull”— like vascular structure best visualized in the left lateral or left anterior oblique view. Following intervention, repeated cardiac catheterization is essential to assess results and to plan for subsequent interventions when staged repair is necessary. In addition to imaging, acquisition of hemodynamic data is important. Presence of elevated distal pressure within collateral vessels (mean pressure greater than 20–25 mm Hg) suggests significant PVOD within the affected lung segments. Evaluation of RV pressure may be helpful when planning VSD closure in patients who have been managed by complete unifocalization and placement of an RV–PA conduit without VSD closure, as is advocated by some authors. RV pressure less than 50 percent systemic (under optimal circumstances) or, at most, up to 75 percent systemic suggests that the VSD can be closed. After unifocalization and right ventricular outflow tract (RVOT) reconstruction without VSD closure, the presence of a left-to-right shunt at the ventricular level indicates adequacy of the pulmonary vasculature to tolerate at least one full cardiac output and suitability for VSD repair.
Figure 69-2
Aortic arch angiogram before (A) and main pulmonary arteriogram after (B) one-stage complete unifocalization in a 2-month-old infant with five MAPCAs and 1.5-mm confluent PAs. VSD was left open. Postoperative angiogram demonstrates complete perfusion of both lungs. (Reproduced with permission from Reddy VM, McElhinney DB, Amin Z, et al. Early and intermediate outcomes after repair of pulmonary atresia with ventricular septal defect and major aortopulmonary collateral arteries: Experience with 85 patients. Circulation 2000;101:1826.)

Computed tomographic angiography (CTA) and magnetic resonance imaging are gaining prominence in evaluation of anatomy of complex collaterals in selected circumstances such as in neonates and in older postoperative patients. Due to difficulty in evaluation of angiograms, three-dimensional CTA and MRI reconstructions may be helpful for detailed anatomic assessment and operative planning.
The initial management of patients presenting with PA, VSD, and MAPCAs is based on medical stabilization of the patient so that full evaluation and proper therapeutic planning can be undertaken. Some patients with excessive pulmonary blood flow may require management of CHF. Interventional cardiac catheterization is important in both short- and long-term management. Interventional procedures may include coil occlusion of collaterals providing duplicate flow to individual pulmonary segments and balloon dilatation of stenoses. Pulmonary valve perforation and dilatation may be performed in the very rare instance of PA with VSD and an adequately developed main pulmonary arterial system.
The ultimate goal of surgical therapy is the creation of separated in-series pulmonary and systemic circulations. Ideal age for repair of this lesion is unknown. If the patient is well balanced physiologically, we prefer to perform the repair when the patient is between 3 and 6 months of age, and perform then a single-stage repair. There are many advantages of early one-stage repair. Cardiovascular physiology is normalized and cyanosis is corrected. The patient is protected against pulmonary hypertension related to high flow either through collaterals or through systemic shunts. The number of operations is reduced, reducing the complexity and morbidity of reoperative surgery. The major problem in management is the natural and intervened history of the MAPCAs and the complexity of surgical intervention on the pulmonary vascular bed (Fig. 69-3).
Figure 69-3
Anatomy of pulmonary blood supply and unifocalization in a 3.5-month-old child who underwent one-stage complete unifocalization and repair of tetralogy of Fallot with MAPCAs. A. Illustration of pulmonary blood supply with left pulmonary artery (LPa) and right pulmonary artery (RPa), a coronary collateral (COR) connecting to the true pulmonary artery, and three MAPCAs (1–3) from the Innominate artery (IA) and descending aorta (AO). B. MAPCAs are opened along the dashed lines A–A′, D–D′, and E–E′, whereas the true pulmonary arteries are filleted open from hilum to hilum (B–B′). C. Complete unifocalization and reconstruction of the pulmonary artery is completed with full native tissue continuity. Collateral 1 A–A′ is anastomosed side to side to the incision B–B′ in the true pulmonary artery as a long onlay. Collateral 2 is anastomosed end to side to the undersurface of the RPA from D–D′ to C–C′. Collateral 3 is anastomosed end to side to the undersurface of the LPA from E–E′ to F–F′. (Reproduced with permission from Reddy VM, Liddicoat JR, Hanley FL. Midline one-stage complete unifocalization and repair of pulmonary atresia with ventricular septal defect and major aorto-pulmonary collaterals. J Thorac Cardiovasc Surg 1995;109:832–845.)

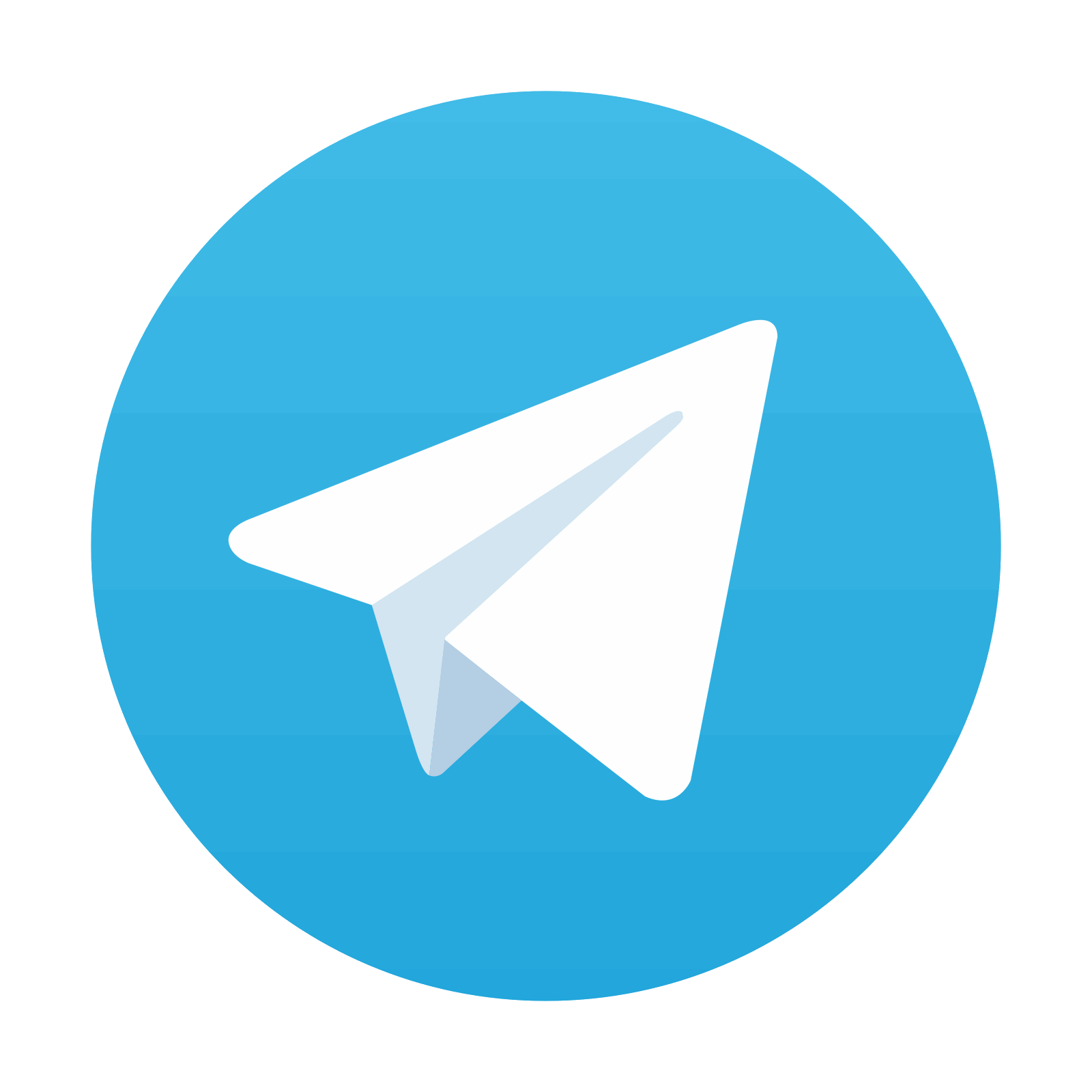
Stay updated, free articles. Join our Telegram channel

Full access? Get Clinical Tree
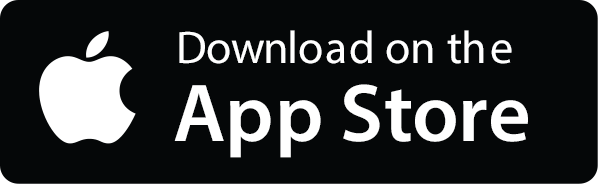
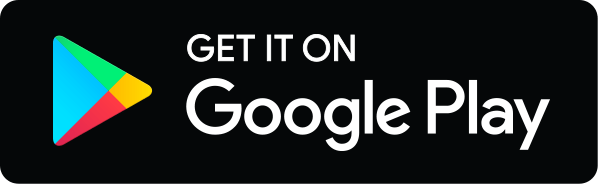