28 Mark J. Salji1,5,7, Imran Ahmad1,5, Sarah Slater1,2,5, Fat‐Wui Poon3, Abdulla Alhasso2, Nadja V. Melquiot4, Holly Bekarma1, Jane Hendry8, and Hing Y. Leung1,2,5,6 1 Department of Urology, NHS Greater Glasgow and Clyde, Glasgow, UK 2 The Beatson West of Scotland Cancer Centre, NHS Greater Glasgow and Clyde, Glasgow, UK 3 Department of Radiology, NHS Greater Glasgow and Clyde, Glasgow, UK 4 Department of Histopathology, Forth Valley Royal Hospital, Larbert, UK 5 Beatson Institute for Cancer Research, Glasgow, UK 6 Institute of Cancer Sciences, University of Glasgow, Glasgow, UK 7 Institute of Cancer Sciences, University of Glasgow, Glasgow, UK 8 Department of Urology, Queen Elizabeth University Hospital, Glasgow, UK Prostate cancer (PCa) is the most common urological cancer and accounts for nearly a quarter of all new cancers in men. Since the widespread use of prostate‐specific antigen (PSA) testing in the 1990s, the incidence of PCa has risen sharply, but overall mortality from PCa has remained static. All areas of PCa from diagnosis through to surgical and medical treatments are rapidly advancing. Besides ongoing evaluation of novel biomarkers and genetic profiling, ground‐breaking advances in MRI imaging, biopsy techniques, robotic prostatectomy, imaging‐guided radiotherapy, and multiple large‐scale clinical trials of new drugs within the last decade make PCa an exciting and ever‐expanding field in urology, thus transforming how urologists manage patients with PCa. Keywords prostate cancer (PCa); magnetic resonance imaging (MRI); prostate‐specific antigen (PSA); prostatectomy; radiotherapy In the Western world, prostate cancer (PCa) incidence has increased generally since 1970s with a rapid increase in the 1990s and is largely attributable to an aging population and prostate‐specific antigen (PSA) testing. Widespread PSA testing has led to the diagnosis of less‐aggressive cancers in younger men who are asymptomatic [1]. PCa incidence varies globally, with highest rates in North America and Scandinavia and lowest rates in East Asia [2, 3]. Similarly, PCa mortality varies worldwide, with the highest mortality in the Caribbean and Scandinavia, intermediate rates in US and UK, and lowest rates in China and Japan [4]. PCa has become the most common cancer in men, with more than 41 000 new cancers per annum in the UK, or a lifetime risk of one in eight, accounting for 25% of all new cancer cases in UK men [1]. More than 10 000 men every year die from PCa. The crude UK incidence rate for 2012 was 134 new PCa cases per 100 000 males [1]. Only 8% of men will get clinically significant PCa and only 3% will die from PCa. This is because PCa commonly has a slow natural history and is predominantly a cancer of elderly men. The majority of men (84%) with PCa are predicted to survive more than 10 years [5]. The aetiology of PCa is poorly understood. Established nonmodifiable risk factors include age, ethnicity, and family history (genetic susceptibility) have been associated with PCa. Epidemiological migration studies suggest modifiable risk factors such as environmental and lifestyle factors to be important as well, however, have not been conclusively linked to PCa development. PCa has been considered as a cancer of aging as suggested by autopsy studies where nearly 30% of men 50–60 years of age were found to have PCa, nearly 40–50% of men in their 60–70 years of age, >65% in their 70–80 years, more than 80% in their 80s, and all men in their 90s had PCa [6]. Albeit the vast majority of men will die with PCa than of it. Only 1% of PCa cases are diagnosed in men younger than age 50 [1]. The peak age for diagnosis is 75–79 (Figure 28.1). PCa is already a huge health problem in the Western world and its importance on health economics will only increase with an aging population and increasing life expectancy. The population of men aged 65 or older is predicted to increase fourfold between the years 2000 and 2050. By 2030, the older‐than‐65 population is forecast to make up about one‐fifth of the global population demographics [7]. Figure 28.1 Prostate cancer, average number of new cases per year and age‐specific incidence rates, UK males 2009–2011. Source: Taken from UK Cancer Research, 2012 [5]. Hereditary PCa is defined as having three generations affected or three first‐degree relatives or at least two relatives younger than 55 years of age with PCa. First‐degree relatives of men with PCa have approximately twice the risk of developing the disease, relative to men without a family history [8]. This is compounded by an early age of onset, in that if the first‐degree relative has PCa diagnosed at younger than 60 years of age, the risk is more than fourfold that of men without a family history [9]. In Nordic twin studies, when one of the twins was diagnosed with PCa, identical twins (monozygotic) carried a 50% higher risk of PCa when compared to dizygotic twins [10, 11]. It is estimated that 5–10% of all PCa cases and up to 40% of those occurring in men <55 years of age may be hereditary [12, 13]. When compared to sporadic cases, hereditary PCa tends to be diagnosed on average six to seven years earlier [13]. There have been seven susceptibility gene loci for PCa identified, including HPC‐1 (chromosome 1q24–25) and RNaseL, but demonstrating linkage has proved to be problematic [14]. Genome wide association studies (GWAS) have identified more than 40 PC‐risk genetic variants [15]. Somatic genetic alterations in fatty acid synthase (FASN), Hepsin (HPN), alpha‐methylacyl‐CoA racemase (AMACR), and MYC have been fairly consistent and linked to PCa development [16]. Deleterious germ line mutations of BRCA1 and BRCA2 (chrom 13q) are associated with increased PCa risk [14, 17]. Incidentally, family history of breast cancer has been associated with the same mutations of the BRCA genes. Generally, African American men are at a higher risk of PCa, whereas Asians have a lower risk. The highest incidence is seen in people of African American and Jamaican descent and lowest in people native to Japan [18]. Many modifiable risk factors have been studied to find a linkage with development or progression of PCa; however, no strong associated evidence was found for many of these factors with scanty evidence at best. Migration studies of Japanese men settling in the US showed that their PCa incidence increases and with increased risk correlated with younger age at the time of immigration and longer duration living in the new environment [19]. In a multicentre study of dietary factors, PCa risk was associated with total fat intake in Caucasians, African Americans, and Asian Americans [20]. There is evidence that dietary fat (omega‐6 polyunsaturated fatty acids: present in red meats) may play a role in accelerating tumour growth [21]. Conversely, various studies have suggested that cooked and processed tomatoes or tomato products (rich with the lycopene antioxidant), β‐carotene, selenium, vitamins E and D, omega‐3 unsaturated fatty acids, and other antioxidants may have a weakly protective effect for PCa development [22, 23]. In a US prospective study of 400 000 men with high body mass index (BMI; 35–39.9) had increased risk of dying from PCa, and it was 34% higher when compared to those with normal BMI [24]. Furthermore, high BMI has been found to be associated with advanced PCa [25, 26]. Possible biological mechanisms include higher levels of androgens, insulin‐like growth factor 1 (IGF‐1), and serum calcium which are associated with PCa development. Despite the known androgen dependence of PCa, there is little evidence that circulating levels of androgens, oestrogens, or 5α‐reductase levels are associated with risk of developing the disease [16]. PCa risk does not appear to be affected by other factors such as vasectomy, sexual activity, smoking, alcohol consumption, physical activity, or social class [4, 23]. 5‐alpha reductase inhibitors (5ARI) such as finasteride and dutasteride have been studied for their possible risk reducing effects in PCa [27, 28]. Using 13 years’ of survival data from the Prostate, Lung, Colorectal and Ovarian (PLCO) trial and data from the Reduction by Dutasteride of Prostate Cancer Evens (REDUCE) trial (a clinical research study to reduce the incidence of prostate cancer in men who are at increased risk), both initially showed a reduction of the incidence of PCa, but further analysis showed an increase in the risk of higher‐grade disease (i.e. Gleason 8–10), suggesting a worrying slight increase in more aggressive PCa [27, 28]. In the REDUCE trial, which compared 5ARI, dutasteride, and placebo in 6729 men with raised PSA (2.5–10 ng ml−1), showed a 22.8% relative risk (RR) reduction from 25.1% (848 of 3424) to 19.9% (659 of 3305). However, during the last two years, a significantly greater number of patients in the dutasteride group were diagnosed with high‐grade Gleason score 8–10 (1 out of 2343 compared to 12 out of 2447). Secondary analysis of Prostate Cancer Prevention Trial (PCPT) data also showed a RR reduction in PCa mortality of 0.87 (95% CI 0.72–1.06). Currently no 5ARIs have been licenced for use in risk reduction in PCa. Statins have also been implicated in PCa prevention. Epidemiological studies have suggested a reduction in the rate of PCa in patients taking statins; however, the evidence in not clear [29]. The effects of statins in PCa cell culture is to induce apoptotic cell death via reduction of the insulin‐like growth factor 1 receptor (IGF‐1R) and can also be potentiated by the effects of IGF‐1R antagonists [29–31]. As the most prevalent male cancer, PCa has become an increasingly pressing clinical problem for urological surgeons worldwide [3]. Despite its prevalence, the disease is poorly understood by the general public, and most men older than the age of 60 will have some experience of PCa through family or friends. Early or localised PCa (T1–2, N0 M0) is typically detected following investigations for concurrent lower urinary tract symptoms (LUTS), haematuria, or after a screening PSA test [32]. Locally advanced PCa (T3–4, N0 M0) can cause similar symptoms as the localised disease in addition to obstructive uropathy or lower limb oedema if lymphatics are involved. Nearly 10% of patients present with signs and symptoms of advanced or metastatic PCa with general health decline, weight loss and cachexia, falls and pathological fractures or bone pain from bony metastases, or lower limb weakness from spinal cord compression by vertebral metastases. Bearing in mind metastatic disease can also be asymptomatic and only present with an elevated PSA. For many, PCa has an indolent nature, with a prolonged natural history with at least 10 years from initial diagnosis to the first cancer‐related mortality [33]. Hence, it is necessary to risk‐stratify newly diagnosed PCa into low, intermediate, and high‐risk groups. Low‐risk PCa confers a disease‐specific mortality of <1% over 10 years follow up, thus the option of active surveillance (AS) with deferred treatment is generally used. [33]. This increases dramatically for intermediate‐ and high‐risk PCa groups to about 10% cancer‐specific mortality at 10 years without radical treatment [34]. The prognosis of patients with metastatic PCa remains poor, with an average survival of only 42.7 months (3.5 years) from diagnosis, while once castration‐resistant PCa (CRPC) has developed, time to death is typically within 22 months [35]. Locally advanced PCa also impacts prognosis, increasing the risk of cancer‐specific death by seven times with an overall 10 years cancer‐specific mortality rate of 23.9% [36]. Therefore, the understanding of the natural history of PCa is vital to ensure patients are managed appropriately. PSA is a 34 kDa serine protease, also known as Kallikrein Related Peptidase‐3 encoded by the KLK3 gene on chromosome 19. Its physiological function is the hydrolysis of semenogelin‐1, causing liquefaction of the seminal ejaculate to aid spermatozoa mobility [37]. It has a half‐life of 2.2 days with 75% of circulating PSA bound to alpha‐1 antichymotrypsin and alpha‐2 macroglobulin and is metabolised in the liver. The remaining 25% is free and is excreted in the urine. It is found in high quantities in seminal fluid and its discovery as a PCa serum biomarker occurred concurrently with its discovery and use in forensic analysis of seminal fluid leading to many pseudonyms such as γ‐seminoprotein. In 1979 T. Ming Chu purified and characterised PSA from serum and was the first to appreciate its clinical relevance as a biomarker for PCa [38]. Prior to PSA, prostatic acid phosphatase (PAP) was widely used; however, it was only raised in metastatic disease and was not useful in early detection or screening. In 1987, Stamey et al. showed that PSA was superior to PAP as a clinical biomarker for detecting early PCa [39]. Today, PSA is the most widely used clinical biomarker. The major limitation of PSA as a biomarker for PCa is that it is produced by both normal and malignant prostatic epithelium, and its detection in serum is proportional to the amount of prostate tissue (both healthy and diseased, benign and malignant), with variable normal range amongst men, increasing with age (Table 28.1). PCa cells produce less PSA than non‐PCa cells; however because of the disruption of the basement membrane architecture of the gland in cancer, more PSA ‘leaks’ into the circulation. Table 28.1 Age‐specific reference ranges used to trigger referral for prostate biopsy adapted from NICE guidelines [40, 41]. NICE, National Institute for Health and Care Excellence. A number of conditions that irritate the prostate can cause the serum PSA to elevate such as, urinary tract infections (UTIs), prostatitis, benign prostatic hyperplasia (BPH), acute urinary retention, catheterizations, prostatic biopsy or operations such as transurethral resection of prostate (TURP). Prostatic massage, digital rectal examination (DRE), and anything causing pressure on the perineum may also transiently increase the PSA levels. Avid cyclists have been known to have an intermittently raised PSA due to pressure from the bike saddle and strenuous exercise [42]. The PSA kinetics, rather than the absolute values, are more important in identifying men harbouring an early tumour [43]. Ultimately, to decide whether to proceed to prostatic biopsy requires a fully informed patient on the risks and benefits of the procedure and should not be based on PSA alone, taking into account an individual’s risk of PCa and comorbidity factors as well as any co‐existing UTI or prostatitis and findings on DRE [40]. A number of PSA kinetics exist, but only PSA doubling time and velocity are regularly used in clinical practice for monitoring in AS and during biochemical relapse following treatment [44]. They are a measure of increasing volume of disease and the rate of progression and can predict PCa specific survival [45]. PSA density is the serum PSA level per ml of prostate tissue. PSA levels are higher in men with larger prostate glands. The PSA density is sometimes used for men with large prostate to try to adjust for this. The volume of the prostate gland can be measured using transurethral ultrasound (TRUS) or computed tomography (CT) scans. A PSA density of >0.15 ng ml−1 per ml of prostate tissue is highly indicative of PCa [46]. Doubling time is the length in months or years in which the PSA doubles. The doubling time is used more for follow‐up of patients’ progress after treatment or during AS. Clinical examples of the doubling time use: a doubling time of five years is highly suggestive of PC, while three years in patients undergoing AS indicates disease progression, and a doubling time of three months after radical treatment might trigger need for hormonal therapy. Multiple online calculators can aid in the doubling time calculations (e.g. https://www.mskcc.org/nomograms/prostate). PSA velocity is the rise in PSA per year, with a rise of more than 0.75 ng ml−1 per year being associated with a higher risk of PCa [47]. The velocity can be calculated by using the following formula: PSA Velocity = 0.5 × ([PSA2 – PSA1/time1] + [PSA3‐PSA2/time2]); time being in years. As the majority of PCa arises in the peripheral zone of the prostate, PCa can be easily palpated during DRE and used for staging purposes as well. A DRE can give you an estimation of the size of the prostate, symmetry, firmness, presence of nodules, and in advanced disease, a craggy solid fixed feeling prostate. Although an abnormal feeling prostate would raise the suspicion of PC, the risk increases if the PSA was also raised (Table 28.2) [48, 49]. DRE alone can detect only 18% of PC, irrespective of the PSA. Abnormal DRE with an elevated PSA level of >3 ng ml−1 gives a positive predictive value of just under 50% [50] A number of risk calculators are available online [51]. Table 28.2 Predicted value for prostate cancer based on digital rectal examination (DRE) and prostate‐specific antigen (PSA). Prostate biopsies remain the clinical standard and the most reliable means to diagnose PCa (Figure 28.2). Figure 28.2 Imaging algorithm in patients with elevated prostate‐specific antigen (PSA), abnormal rectal examination, or family history of prostate cancer. mpMRI, multiparametric magnetic resonance imaging; MRI, magnetic resonance imaging; PSA, prostate‐specific antigen; TRUS, transurethral ultrasound. Source: Modified from ESUR prostate MR guidelines [52]. On grey scale TRUS, the normal central part of the prostate has a lower echogenity (darker) compared with the peripheral part (Figure 28.3). About 60–75% of the tumours have a lower echogenicity compared with the surrounding prostate tissue. Many nonmalignant conditions such as prostatitis, infarction, and adenoma may give similar hypoechoic appearance. Approximately 12–30% of prostate tumours are isoechoic and not visible with the usual grey scale ultrasonography. A small proportion of tumours are hyperechoic [53]. The sensitivity and specificity of TRUS are therefore too low for PCa screening, and its main role is to provide guidance for prostate biopsy. Figure 28.3 Cancer of the prostate may be more (hyperechoic), less (hypoechoic), or equally dense (isoechoic) on ultrasound as the normal tissue. Compared with normal prostate tissue, malignant focus has increased cell density and therefore a change in tissue elasticity. When manual compression is applied, this change in tissue elasticity causes a change in reflection of applied sound waves, and this can be detected by a technique known as ‘elastography’. Elastography is able to increase PCa detection compared to the standard grey scale TRUS [54]. Early results are promising, with one study showing a PCa detection rate of about 93%, compared with 59% by grey scale TRUS [55]. Shear wave elastography is an alternative technique using acoustic impulse to produce a shear wave. This technique is less operator‐dependent and is easier to perform because it does not require the operator to apply pressure. Early published results are favourable [56], but large prospective trials are needed to fully evaluate their value as imaging tools to diagnose PCa. TRUS also allows the measurement of the prostate size, which is the equation for area of an ellipsoid: prostate volume (cm3) = anteroposterior diameter (cm) × width (cm) × sagittal (cm) × (π/6). Each patient should be informed of how the procedure is carried out (no enema is required) and the potential complications; written informed consent should be obtained. Anticoagulation therapy, warfarin and anti‐platelet agents, should be stopped before biopsy, and antibiotic prophylaxis must be given to all patients. General anaesthesia is rarely necessary and local peri‐prostatic anaesthesia should be given. The diagnostic yield of systemic sextant biopsies varies between patient population studied, but in general, between 20 and 35% of patients are confirmed to have cancer using the original technique described by Hodge et al. [57]. Increasing the number of biopsy cores and biopsy areas will increase the yield of the procedure. Most studies had demonstrated that extended biopsy is superior to the sextant protocol without incurring significant complications [58]. The addition of laterally directed biopsies has shown to increase the PCa detection rate by 5–35% [59, 60]. This is explained by the simple fact that PCa mainly arises in the outer zone of the prostate (Figure 28.4). The base and the apex of the peripheral gland are the sites where most tumours are located and where biopsies should be directed. Biopsies of the transitional zone add little to cancer detection and should therefore not be sampled during initial biopsy [61]. Recommendations are for 10–12 core samples taken during systematic biopsy from as far posterior and lateral in the peripheral gland as possible. These can be supplemented with cores from suspected areas detected by either DRE or TRUS [32]. Figure 28.4 Most invasive cancer arise in the outer zone of the prostate which is not removed by transurethral resection of prostate (TURP); hence, cancer is often found in the tissue left behind. TRUS‐guided biopsy is in general a safe procedure. Complications are minor and self‐limiting. These include vasovagal episode, pain, mild haematuria, transient rectal bleeding, haematuria, and haematospermia. Major complications are rare; however, they can lead to significant morbidity and include severe rectal or urinary bleeding, acute or clot urinary retention and infection, and severe sepsis. Because a negative biopsy does not rule out PCa, a continued suspicion of malignancy on the basis of rising or persistently raised PSA level, suspicious DRE, or abnormal histology such as atypical small acinar proliferation (ASAP) [32], a repeat biopsy is usually indicated. It has been shown that more than 90% of PCa can be detected by two successive sextant biopsies [60] and is unlikely to miss any clinically significant disease. However, the more biopsies obtained, the less the probability of finding PCa is, with 22% on the first biopsy, 10% on the second, 5% on the third, and 4% on the fourth [62]. Furthermore, the more biopsies taken, the more likely a lower‐grade disease will be detected, therefore, more likely a clinically insignificant disease. In saturation biopsy, 20 or more cores of tissue are obtained in a systemic fashion. The theory behind the saturation biopsy is that the more biopsies one takes, the higher is the chance of detecting PCa [63, 64]. However saturation biopsy is recommended only for patients with an initial negative biopsy because this technique does not offer any benefit as a routine initial biopsy protocol [65]. The approach of transperineal template prostatic biopsies can also be considered. If clinical suspicion for PCa persists despite repeated negative prostate biopsies, magnetic resonance imaging (MRI) may be used to investigate the possibility of an anterior located tumour, which can then be targeted for biopsy [32]. 18 F‐fluorodeoxyglucose (FDG) positron emission tomography–computed tomography (PET–CT) is now widely used for staging, restaging, and monitoring of treatment response of an increasing number of cancers. The rising number of incidental prostatic 18FDG uptake has created a dilemma for clinicians. A retrospective review of more than 11 000 men who underwent 18FDG PET–CT showed the prevalence of incidental prostate uptake to be 1.8%. Within this group, there was a one‐in‐five chance of finding PCa in the older patient with uptake within the peripheral zone; in such incidental cases, referral and further evaluation is suggested [66]. However, as a primary imaging modality, 18FDG PET–CT does not reliably detect primary PCa due to low metabolic activity within PCa tumours and also signals being obscured by contrast accumulation in the urine [40]. Classification is by the tumour, node, and metastasis (TNM) staging system (Table 28.3 and Figure 28.5) [67]. Table 28.3 TNM staging. PSA, prostate‐specific antigen; TNM, tumour, node, metastasis; TURP, transurethral resection of prostate. Figure 28.5 Diagrams representing tumour, node, metastasis (TNM) staging system for prostate cancer. PCa spreads by direct invasion, lymphatics, veins, and along the perineural spaces, and adequate staging should precede treatment. Direct invasion occurs into seminal vesicles, urethra, base of the bladder, and around the rectum. Cancer never penetrates Denonvilliers’ fascia unless it has been perforated by a biopsy needle. The notion of the ‘capsule’ in the context of carcinoma is misleading. The only anatomical capsule is a thin layer of connective tissue continuous with that of the veins and fat around the gland. This layer of connective tissue is thinner than the loop of the resectoscope and extends alongside the small nerves, arteries, veins, and lymphatics which enter the prostate at many places. In addition to the lymphatics each nerve is surrounded by a perineural space in which cancer readily spreads. Lymph nodes may be invaded in many apparently localised cancers. In addition to the involvement of lymph nodes by prostatic cancer, there is a direct route from the prostate through its veins and lymphatics to the marrow of the vertebrae, pelvis, and upper third of the femur. CT defines the intraprostatic features poorly and is not reliable for local tumour staging. If MRI is contraindicated, CT can be offered, if knowledge of staging could affect management. Recent technical improvement and the availability of functional parameters such as diffusion‐weighted imaging (DWI), dynamic contrast‐enhanced, and spectroscopic imaging have greatly improved the accuracy of MRI in local tumour staging. Multiplanar T2‐weighted (T2W) imaging is the foundation of multiparametric examination and provides high‐resolution images of the prostate’s zonal anatomy and capsule (Figure 28.6a). T2W imaging alone is estimated to have a sensitivity of 48–88% and specificity of 44–81% for cancer detection [41, 68]. Criteria for extracapsular extension includes capsular irregularity, localised bulge, measurable extracapsular disease, and obliteration of the rectoprostatic angle (Figure 28.6b). Figure 28.6 (a) MRI of pelvis with normal anatomy axial T2W image showing normal zone anatomy of the prostate. The peripheral zone (P) exhibits high T2W signal (bright) with a lower T2W signal (darker) from the central (C) and transitional zones. The prostate capsule is represented by a thin dark rim outlining the prostate gland. (b) MRI pelvis T3 prostate cancer, axial T2W image of the prostate showing the extracapsular spread of the tumour. (c, d, e) DWI (c) Axial T2W image of a hypointense (darker) prostate tumour. (d) DWI reveals the tumour as a hyperintense mass (e) On ADC map, the tumour shows hypointensity (restricted diffusion) compared with normal prostate parenchyma. (f) Radioisotope bone scan Multiple 99mTc‐MDP avid lesions are present in the ribs, spine, pelvis, shoulders, and femurs. This uptake pattern is in keeping with multiple bone metastases. ADC, apparent diffusion coefficient; DWI, diffusion‐weighted imaging; MRI, magnetic resonance imaging; T2W, T2‐weighted. Biopsy can cause haemorrhage, inflammation, and infarction. This causes abnormalities that can mimic tumour and reduces the accuracy of the staging MRI. Most radiologists therefore recommend an interval of at least six to eight weeks between biopsy and MRI to minimise this artefact. DWI measures the movement of water molecules through the soft tissues under the influence of strong magnetic field and radiofrequency pulses (Figure 28.6c–e). Cancer tissue exhibits a reduced diffusion compare to normal tissue. Apparent diffusion coefficient (ADC) maps of the prostate are then derived which demonstrate the tumour as a low signal focus relative to surrounding normal prostate. DWI, therefore, provides an easily visible contrast between normal and cancerous tissue. Combination of DWI with the more anatomical T2W imaging improved the overall diagnostic accuracy with an estimated sensitivity and specificity of 80–90% [69, 70]. In dynamic contrast‐enhanced MRI (DCE‐MRI), multiple rapid sequential images of the prostate are obtained following the intravenous administration of a gadolinium‐based contrast medium. The increased perfusion and permeability of the new vessels in PCa will cause an early, fast, and intense enhancement of the tumour with early wash‐out of the contrast agent. By comparison, normal peripheral zone tissue usually shows a less intense, slower, and progressive wash‐in. The DCE‐MRI data and the resultant enhancement curves can be assessed qualitatively (visually), semi‐quantitatively, or quantitatively with appropriate imaging software. As there is considerable overlap between the contrast enhancement rate for the tumour, normal tissue in the transitional zone, and hypervascular benign prostatic hypertrophy, DCE‐MR is not recommended to be used as a stand‐alone study [71]. It is a helpful tool for detecting, localising, and staging PCa but should be used in combination with T2W imaging, DWI, and possibly spectroscopic imaging as part of a multiparametric approach to prostate imaging [52]. To improve consensus on the diagnosis and management of patients with localised PC, the American College of Radiology, the European Association of Urology Section of Urological Research (ESUR), and the AdMeTech Foundation formed a joint committee to develop essential standards for a Prostate Imaging Reporting and Data System (PI‐RADS). The goal was to facilitate the application of high‐quality MRI by radiologists, urologists, and oncologists in a user‐friendly diagnostic scoring format. In 2015, PI‐RADS v2 (updated PI‐RADS system as version 2) [72] was introduced, providing a simplified numerical score from one (most probably benign) to five (most likely malignant) for each intraprostatic lesion on MRI. The score is based on T2W images, a DCE study, and DWI sequence. Each study provides useful information on the lesion scored. T2W images are scored from one to five for the peripheral zone and transitional zone separately providing anatomical localization and lesion size. DWI is scored from one to five, confirming the lesion on T2W imaging is likely malignant. DCE is either positive or negative and may be omitted in the latest scoring system depending on the quality of the study. The updated system also ensures that an index lesion is identified as the most clinically significant intraprostatic lesion. Local pelvic nodal disease can be estimated as part of the overall MRI examination. About 70% of the involved lymph nodes are too small (<8 mm) to be evaluated using MRI, so conventional size criteria tends to underestimate the extent of nodal involvement [73]. In an autopsy study, haematogenous spread was found in 35% of the 1589 patients with a history of PCa. The most frequent involved sites were bone (90%), lung (46%), liver (25%), pleura (21%), and adrenals (13%) [74]. It was also noted that the burden of bone disease correlates directly with survival [75]. In patient where distant extraskeletal metastasis is suspected, a whole body CT is often indicated. Skeletal metastasis is currently routinely assessed by radionuclide imaging using technetium methylene diphosphonate (99mTc‐MDP; Figure 28.6f). Radioisotope bone scan is not indicated in asymptomatic patients if the serum PSA level is <10 ng ml−1 in the presence of well‐differentiated or moderately differentiated tumours [32]. If PSA is <20 ng ml−1, <5% of patients will have lymph node involvement and <1% will have skeletal metastasis. Radioisotope bone scan has an acceptable false‐negative rate but a high false‐positive rate mainly as a result of other coexisting hyper‐metabolic lesions such as degenerative disease, fractures, healing metastasis, or Paget disease. Metastatic involvement is usually demonstrated by increased activity and is more commonly seen in bone with a greater percentage of red marrow such as pelvis, spine, ribs, and skull. Although the predictive value of radioisotope bone scans increases with the number of metastatic sites, the ability to ascertain the exact nature of individual lesions is limited without further diagnostic tests. Traditionally, plain radiograph of the abnormal area is taken to detect the presence of possible sclerotic metastasis, but advances in other imaging techniques have rendered this almost obsolete. Single photon emission computed tomography–computed tomography (SPECT–CT), utilising a scanner that can obtain planar and cross‐sectional isotope images as well as selected CT images of the affected area during the same examination, increases the specificity and number of lesions identified compared with the traditional planar radioisotope bone scan. For example, increased tracer activity in the vertebral‐pedicle combination has a predictive value of 73–82% for metastatic disease, while the demonstration of activity only in the facet was 100% predictive of benign degenerative changes [76]. SPECT scanning alone could detect lesion down to 2 mm in diameter [77]. Diffuse metastatic deposits involving most of the skeletal system (super‐scan) will produce generalised increased activity of most bones and may be difficult to be recognised. The hypermetabolic response as a result of healing metastasis (i.e. flare response) can lead to a false‐positive diagnosis of disease progression. It usually takes between three to six months for this flare response to subside completely. If the radioisotope bone scan is taken three months after introduction of therapy shows worsening of disease, there is a high probability that this represents metastatic disease. If, however, the patients’ clinical parameters indicate a response, then flare response should be considered. A follow up scan at six months will usually resolve the issue [78]. If the result of the radioisotope bone scan or SPECT–CT remains uncertain, other imaging modality such as MRI has been recommended to differentiate active metastases from benign or healing disease [32]. MRI, which can demonstrates early bone marrow infiltration, is more sensitive than radioisotope bone scan with sensitivities and specificities of 100 and 88% for MRI and 46 and 32% for radioisotope bone scan, respectively [79]. Rising PSA after radical prostatectomy or radiotherapy signifies biochemical recurrence. Although the patient’s symptomatology and pathology may be helpful, further imaging is often needed to identify possible local, nodal, or distant disease. TRUS biopsy is effective in about 50% of post radiotherapy relapse. MRI and CT are the two most frequent imaging modalities used currently to evaluate such patients, but results remain poor largely because of low‐volume disease [73]. Radioisotope bone scan is useful for possible bone metastasis, but its lack of specificity often requires further imaging evaluation. PET–CT already has an established role in the management of a large number of cancers. Unfortunately, most PCa cells have a low avidity towards the most commonly used radiotracer, FDG. In addition, this tracer is excreted by the kidneys, and the presence of bladder activity limits pelvic evaluation unless bladder catheterisation and diuresis are used. Altered choline metabolism has been noted as a characteristic of PCa and novel radiotracers such as 18F‐Choline and 11C‐Choline have been investigated as a single non‐invasive modality in staging patients with biochemical recurrence after primary treatment with a sensitivity between 43 and 93% [80, 81]. Prostatic intraepithelial neoplasia (PIN) consists of an abnormal proliferation of cytologically atypical epithelial cells within the prostatic glands without stromal invasion. It does not secrete PSA. Glands involved by PIN retain a layer of basal cells. It constitutes the preinvasive stage of prostatic acinar adenocarcinoma [82]. The incidence of isolated PIN in prostate biopsies is on average 9% [83]. Both the incidence as well as the extent of PIN increases with age [6, 84]. Based on the nucleoli prominence, PIN can be either low grade or high grade. PIN carries a predictive value for cancer in repeated biopsies ranging from 18 to 58% [85, 86]. The predictive value is especially high if PIN is of high grade, multifocal, and present in multiple (>4) core biopsies [87]. The recent use of extended biopsy techniques and more thorough prostate sampling is leading to a reduced positive predictive value of PIN as more cancers are picked up on initial biopsy [88, 89]. Atypical small acinar proliferation (ASAP) is a term used for small foci of atypical prostatic acini in prostate core biopsies that are suspicious for cancer but fall below the diagnostic threshold. ASAP often represents undersampled cancer, with about 60% positive for cancer on repeat biopsies [41, 90, 91]. Repeat biopsies are required if high‐grade, multifocal PIN or ASAP are detected. The majority of cancers arising within the prostate are adenocarcinomas, most displaying acinar histologic features (Figure 28.7). These acinar adenocarcinomas comprise an extremely heterogeneous group of malignancies and can range from well‐differentiated cancers, with appearances very similar to benign prostatic glands, to poorly differentiated tumours with no resemblance to prostatic tissue [92]. Figure 28.7 Histopathology of prostatic adenocarcinoma. (a) PIN, (b) foamy PIN, (c) acinar adenocarcinoma, (d) mucinous adenocarcinoma, and (e) foamy adenocarcinoma. PIN, prostatic intraepithelial neoplasia. Other variants of adenocarcinoma include ductal, foamy, mucinous, atrophic, pseudohyperplastic, oncocytic, signet ring cell, and lymphoepithelioma‐like adenocarcinoma; the latter two are associated with a poor clinical prognosis. The recognition of histologic variants of PCa is important. Some types are cytologically very bland and may be mistaken for benign changes, while other types are associated with a distinct clinical outcome [92, 93]. Some adenocarcinomas show focal neuroendocrine differentiation. This transformation to a neuroendocrine phenotype is one possible mechanism of resistance to androgen receptor–targeted treatments because neuroendocrine cells lack androgen receptors and secrete various neuroendocrine peptides that stimulate androgen‐independent proliferation [1, 94–96]. Squamous cell and adeno‐squamous carcinomas are rare, aggressive tumours that can arise after radiation or hormonal therapy [92, 97]. Neuroendocrine tumours comprise small cell carcinomas and extremely rare large cell neuroendocrine carcinomas and carcinoids. These neuroendocrine carcinomas can be pure or mixed with adenocarcinomas [98, 99]. Small cell carcinomas (Figure 28.8) are poorly differentiated neuroendocrine carcinomas. They are rare and extremely aggressive tumours. They have often already metastasised at presentation but show low PSA. They can also arise after radiation or hormonal therapy [41, 92]. Figure 28.8 Histopathology of prostatic small cell neuroendocrine Carcinoma. (a) H&E, (b) Chromogranin, (c) Synaptophysin, (d) CD56. H&E, Haemotoxylin and eosin. Carcinosarcoma or sarcomatoid carcinomas are rare biphasic tumours that consist of a malignant glandular and stromal component and carry a poor prognosis [93, 97]. Prostatic stromal sarcomas are rare tumours and can arise in patients of all ages with prognosis depending on grade and stage [92, 100]. Other sarcomas (e.g. leiomyosarcoma, rhabdomyosarcoma, and synovial sarcoma) are exceedingly rare [87, 96]. Lymphomas in the prostate can be either primary or secondary [92, 101, 102]. The histologic tumour grade is an important prognostic factor, with impact on treatment choices and patient outcome. Most tumour grading systems assess features of the tumour cells (cytology) or the growth pattern of the tumour (architecture). The Gleason grading system is used in prostatic adenocarcinoma (Figure 28.9). It is primarily based on the tumour architecture. This method was devised in the 1960s and 1970s by Dr. Donald F Gleason [102]. Figure 28.9 Gleason’s grading pattern. Source: Adapted from National Institue of Cancer (http://training.seer.cancer.gov/prostate/abstract‐code‐stage/morphology.html) public domain licence. Prostatic adenocarcinoma is often multifocal and different tumour grades may be present in the same patient [103–105]. As a result, the tumour is assigned a primary pattern for the dominant grade and a secondary pattern for the non dominant grade. The Gleason score is derived by adding these together. The Gleason grades range from 1 to 5, with 5 being very poorly differentiated. Gleason grades 1 and 2 (well‐differentiated cancer which requires nodular circumscription as a diagnostic criterion) cannot be easily diagnosed in the limited tissue of core biopsies. For practical purposes, Gleason grades 1 and 2 (Gleason scores 2–5) are not reported in core‐biopsy specimens but only in prostatectomy specimens [106]. In prostate core biopsies, the Gleason score is the sum of the most prevalent two grades. A tertiary grade can be attributed if a third grade is present, mostly small foci of Gleason grade 5. If only one pattern is present, this will be doubled to reach the Gleason score. In the context of abundant high‐grade cancer, lower‐grade patterns should not be incorporated in the Gleason score. Conversely, any amount of high‐grade tumour should be included because it often reflects more significant high‐grade tumour in the gland [106]. In prostatectomy specimens, the Gleason score is the sum of the most prevalent grade and the less prevalent grade. In 2014, the International Society of Urologic Pathology (ISUP) endorsed modifications to the Gleason grading system by defining grade groups 1–5 based on the Gleason score described previously. This new grading sy stem was initially described in 2013 by Johns Hopkins Hospital and subsequently validated in a multi‐institutional study of 20 845 patients who underwent radical prostatectomy with a mean follow‐up period of three years [107]. The new system includes five distinct grade groups based on the Gleason scoring system described previously. Gleason sum scores of 3 + 3, 3 + 4, 4 + 3, 8, and 9/10 directly translate to Grades 1, 2, 3, 4, and 5, respectively. These grade groups correspond to a five‐year biochemical risk‐free survival based on radical prostatectomy grade of 96% (grade 1), 88% (grade 2), 63% (grade 3), 48% (grade 4), and 26% (grade 5) (Table 28.4). The new grade groups are more accurate at predicting biochemical relapse than Gleason risk stratification groups (≤6, 7, 8–10), aligned with contemporary management strategies including AS. The new system also simplifies explanation to patients. For instance, grade 1 is the lowest grade and >95% of patients will have no detectable recurrence up to five years after radical prostatectomy. In 2016, this new system was adopted by the World Health Organisation (WHO) and will be phased in via side by side reporting to eventually replace the Gleason scoring system [108]. Table 28.4 Translating from the Gleason scoring system to new Gleason grades 1–5 including their corresponding five‐year biochemical relapse‐free survival after radical prostatectomy. Prostatic core biopsy underestimates tumour grade in up to 45% of cases and overestimates in up to 32% of cases. Important factors for this significant divergence are: (i) the number of cores taken because more cores lead to a greater concordance, (ii) the size of tumour because a larger tumour reduces concordance as the small core may not be representative of the whole tumour, (iii) the use of a tertiary grade in prostatectomy specimens, and (iv) interobserver variability [109, 110]. Radiation therapy and androgen‐deprivation therapy (ADT) lead to significant histological changes of benign and malignant prostatic glands, making Gleason grading potentially misleading [111, 112]. In the post radiotherapy setting, some cancer recurrence shows profound treatment effect that precludes grading. Occasionally, both types of cancer (with and without treatment effect) will be seen in the same biopsy. Significant treatment effect can make the diagnosis of residual tumour challenging and often the use of immuno histochemical stains will be required [112]. The use of immunohistochemical staining has increased rapidly in all areas of pathology, and there are many indications in prostate pathology where different antibodies are used to reach a diagnosis (Figure 28.10) [41, 113–115]. Figure 28.10 Immunostains. (a) H&E prostatic adenocarcinoma, (b) AMACR, (c) CK 5/6, (d) p63, (e) 34BetaE12. b highlights invasive adenocarcinoma and PIN. c, d, and e are basal cell markers, which negativity shows invasive adenocarcinoma. AMACR, alpha‐methylacyl‐CoA racemase; H&E, Haemotoxylin and eosin; PIN, prostatic intraepithelial neoplasia. In the presence of small amount of atypical glands, markers for basal cell are used to diagnose invasive malignancy in which the basal cell layer is missing (Figure 28.10c–e). Antibodies that demonstrate a complete absence of basal cells can help diagnose invasion. Basal cell antibodies include p63 (a member of the p53 gene family), 34betaE12 (a high molecular weight cytokeratin), and CK5/6 (another high molecular weight cytokeratin). P504S is a gene primarily expressed in PCa (and PIN) but not usually in benign prostate tissue. It was discovered using a combination of cDNA subtraction and high throughput microarray screening in 2000 [116]. AMACR is often used in combination with basal cell markers to detect invasive prostatic glands (Figure 28.10b) [41, 117]. Interestingly, AMACR is negative in benign neuroendocrine cells but positive in malignant neuroendocrine cells [118]. PSA and prostate‐specific acid phosphatase (PSAP) are used to confirm a prostatic acinar cell origin. Both stains are used to determine the origin of metastatic disease (e.g. in pleural fluids or lymph nodes biopsies) or to rule out the presence of secondary tumours within the prostate when the tumour has an unusual morphology. Prostate cancer is a complex heterogeneous malignancy with a wide range of histopathological features and clinical outcomes. Further histological or molecular markers are needed to better risk‐stratify patients in selecting appropriate treatment [119–121]. In contrast to other tumours where genetic testing or immunohistochemistry is performed routinely to plan the appropriate treatment, molecular tests are currently not available for PCa in a routine clinical setting [122]. In the absence of routinely used molecular markers, the following histological markers are currently included in pathology reports of prostatic core biopsies to predict tumour behaviour: Ki67 is a marker of cell proliferation and is expressed by cells in late G1, S, G2, and M phases, but not in resting cells in G0. It is used to estimate the proliferation index of a tumour. Studies have shown that measuring of the proliferation index can predict PCa outcome. It also appears predictive for biochemical relapse following radical prostatectomy and radiotherapy [123–127]. p27 is a cyclin dependent kinase inhibitor. Loss of p27 has been associated with progression for many carcinomas. Many studies have evaluated the role of p27 in PCa and found that a loss correlates with more aggressive disease [127–129]. The oncogene c‐MYC encodes a transcription factor and is amplified in approximately 70% of PCa. Gain of c‐MYC was found predictive for tumour recurrence after radiotherapy [130, 131]. Epigenetic alterations include gene methylation, microRNA (miR) expression, and histone modification. Gene promoter hyper‐methylation is a molecular mechanism of gene silencing, commonly observed in many cancers. Several genes are known to be methylated in PCa. One of them, PITX2, was shown to be a prognostic marker following radical prostatectomies [132–134]. MicroRNAs (miRs) are small, noncoding RNAs that interfere with gene expression. Studies have shown that higher levels of a specific microRNA (miR‐96) were predictive of earlier biochemical relapse [41, 135–137]. Risk stratification is essential to guide the management of PCa. All men with newly diagnosed PCa are assigned a risk category, and treatment algorithms (Figure 28.11) are based on this classification (Table 28.5) [40]. However, nothing creates more debate amongst clinicians (i.e. urologists and oncologists) than the optimal therapy for localised PCa (T1–2). This is due to the uncertainty of the relative efficacies of the various management options, namely deferred treatment [AS], radical prostatectomy [RP], and radical radiotherapy [RRT]). Treatment is currently based on age (i.e. life expectancy), tumour characteristics (grade and stage), patient performance status, and associated morbidity of the treatment as well as patient and physician preferences (or biases). Figure 28.11 Schematic algorithm of the management of prostate cancer. +/‐, with or without; ADT, androgen deprivation therapy; EBRT, external beam radiation therapy. Source: Adapted from NICE guidance algorithms [40]. Table 28.5 Risk stratification for men with localised prostate cancer. (Please refer to ongoing adoption of a new system of tumour grade grouping described in text.) PSA, prostate‐specific antigen. In general, PCa which is localised can be of low risk to the patient and managed with AS until disease progression at which point definitive treatment can be commenced, thus reducing the period of treatment and side effects. Localised but high‐risk PCa requires definitive management with surgery or radiotherapy therapy because of its higher risk of invasion and metastasis. Locally advanced PCa has already spread beyond the prostate capsule but without evidence of detectable macroscopic metastasis; however, it may be managed either with local radiotherapy, surgery or systemic hormone manipulation as with overt metastatic disease depending on the patient and tumour factors. There is a big difference between the incidence of PCa and death rate attributed to this disease. The incidence of small, localised, well‐differentiated PCa is increasing, mainly as a result of PSA screening and multicore prostatic biopsies [138]. Evidence suggests that up to 45% of men with PSA‐detected PCa are candidates for conservative management [139]. Furthermore, in men with comorbidities and a limited life expectancy, treatment of localised PCa may be deferred to avoid loss of quality of life from treatment, especially because the PCa will not affect their life expectancy. Watchful waiting (WW) (Table 28.6), also known as symptom‐guided treatment, is typically considered for individuals not indicated for radical intervention. This concept was popularised in the pre‐PSA era (pre‐1990) and refers to a conservative approach of PCa until the development of local or systemic (symptomatic) progression. At this stage the patient would be treated symptomatically (TURP for obstruction and hormonal or radiotherapy for palliation of metastasis). Table 28.6 Definitions of Active Surveillance and Watchful Waiting. Source: Adapted for EAU Guidelines on Prostate Cancer. DRE, digital rectal examination; MRI, magnetic resonance imaging; PSA, prostate‐specific antigen. AS or monitoring (Table 28.7) is a recent concept in deferring treatment with a curative intent. Men with early stage, low‐ to intermediate‐risk disease are followed up with serial PSA measurements, DRE, and TRUS‐guided biopsies to ensure stability of the tumour. A variety of AS regimes exist [33]. Popularised in the last decade, it includes an active decision not to treat the patient immediately; instead the patient is kept under close follow‐up. In young patients, it might mean delaying treatment by years. Cancers are usually treated on the first signs of subclinical progression (Table 28.7) [140, 141], with 20–41% of men on such regimes requiring treatment at three to five years of follow‐up. Importantly, treatment at progression appears to be as effective as if it were delivered at the time of diagnosis for most men. Several patient and tumour characteristics were found to be predictive of later biopsy progression and deferred treatment. Men with positive confirmatory biopsies, a higher PSA density, and a higher number of positive cores were at increased risk of progression [142, 143]. Table 28.7 Example protocol of active surveillance adapted from NICE guidelines (January 2014). AS, active surveillance; DRE, digital rectal examination; MRI, magnetic resonance imaging; PSA, prostate‐specific antigen. The first prostatectomy was first performed via the perineal route by Hugh Hampton Young in 1904 and via the retropubic route by Terence Millin in 1945 [144]. However, the procedure remained unpopular because of frequent complications of incontinence and impotence. The popularity of RP followed radical improvements in our understanding of the surgical anatomy of the pelvis [145], including the dorsal venous complex, urethral sphincter, and the course of cavernous nerves, which collectively helped minimise surgical complication rates and improve functional outcomes with reduced blood loss, improved continence rates, and preservation of erectile function via nerve‐sparing techniques. More recently, minimally invasive laparoscopic radical prostatectomy (LRP) and robot‐assisted laparoscopic prostatectomy (RALP) have been developed, with RALP now displacing radical retropubic prostatectomy (RRP) as the gold standard in the US and increasingly worldwide. This has occurred despite the lack of evidence demonstrating superiority of RALP over RRP in terms of critical outcomes of cancer control, preservation of continence, and erectile function [146, 147]. Currently, RRP is the only treatment for localised PCa to show a benefit in overall survival (OS) and cancer‐specific survival (CSS) compared with conservative management at follow‐up of 15 years [34]. The number need to treat (NNT) to avert one death was 15 for all men and 7 for men <65 years of age [34]. Subsequently the Prostate Cancer Intervention Versus Observation Trial (PIVOT) study demonstrated that RP did not reduce all‐cause mortality or significantly reduce PCa mortality [148]. But in subgroup analysis of intermediate (significant) and high risk (non‐significant), there was a reduction in all‐cause mortality. Similarly there was also a significant reduction in all‐cause mortality in men with PSA >10 [148]. Patients with localised cancer undergoing radical surgery have 10‐year disease‐free survival (DFS) ranging from 70 to 85%. High‐grade tumours (Gleason sum >7) have a higher risk of progression. At 10 years, the DFS rates for patients with Gleason 2–6, 7, and >8 tumours are >70, 50, and 15%, respectively. The impact of positive surgical margins remains controversial. Neoadjuvant ADT reduces the risk of positive surgical margins, but it does not impact on long‐term biochemical relapse‐free survival [149], in addition makes surgical dissection more difficult. With the patient head‐down the bladder is catheterized. A long lower midline incision is made and a node dissection is now performed for patients with intermediate‐ and high‐risk disease. The bladder is retracted upwards and the fat cleaned from the retropubic veins. The pelvic fascia is incised on either side of the puboprostatic ligament which contains the dorsal vein (Figure 28.12). The leash of veins is carefully suture ligated, and the layer of pelvic fascia containing the neurovascular bundles are displaced laterally (Figure 28.13). A sling is passed around the urethra and pulled up. The urethra is opened just distal to the apex of the prostate and just cephalad to the membranous urethra and its intramural sphincter (Figure 28.14). The catheter is now used to pull up the prostate, and the tissue behind the prostate – the rectourethralis muscle – is divided in the midline and again displaced laterally and backwards away from the prostate on either side to preserve the penile neurovascular bundles (Figure 28.15). Medial to this bundle, there is a stout leash of inferior vesical vessels which must be suture ligated (Figure 28.16). Figure 28.12 Pelvic fascia is incised on either side of the dorsal veins which are carefully suture ligated and divided. Figure 28.13 The neurovascular bundles are displaced laterally and posteriorly. Figure 28.14 The urethra is opened just cephalad to the membranous urethra and its intramural sphincter. Figure 28.15 The catheter pulls up the prostate, and the rectourethralis muscle is divided in the midline, so that the neurovascular bundles can be again displaced laterally. Figure 28.16 The inferior vesical vessels are ligated. The bladder is opened at the bladder neck (Figure 28.17). Deflating the balloon of the catheter, and using the catheter as a sling, the prostate is lifted up and the bladder is dissected away from the prostate. When the bladder is finally cut across, its calibre is similar to that of the cut edge of the urethra. As the back of the trigone is dissected from the prostate, the seminal vesicles and vasa efferentia are displayed (Figure 28.18). Their arterial bundles are ligated and divided well medial to the penile neurovascular bundles (Figure 28.19). Figure 28.17 The bladder is opened at the bladder neck. The prostate is now slung up with the catheter. Figure 28.18 The bladder is dissected away from the prostate, displaying the seminal vesicles and vasa. Figure 28.19 The vascular leach of seminal vesicles is divided well medial to the penile neurovascular bundles. The bladder neck may have to be narrowed with one or two sutures before it is anastomosed over a 20‐Ch silicone catheter to the stump of the urethra. Great care is taken in making this anastomosis to get a precise urothelial junction and not to injure the intramural sphincter mechanism immediately distal to the site of division (Figure 28.20). It helps to place all the sutures before tying them. Figure 28.20 After narrowing the bladder neck, a precise mucosa‐to‐mucosa anastomosis is made between the bladder neck and the urethra over a 20 F catheter. Place all sutures prior to tying them. Morbidity associated with RP may be significant and is related to surgical experience. Intraoperative complications include blood loss requiring blood transfusion, rectal injury, and ureteral injury. Laparoscopic approaches decrease bleeding rates, but carry the additional risks of laparoscopic access and insufflation, as well as patient positioning (e.g. lower limb compartment syndrome). Perioperative complications include anastomotic leak, deep vein thrombosis, pulmonary embolus, and wound infection. Late complications include urinary incontinence, impotence or erectile dysfunction, and bladder neck stenosis. Extended lymph node dissection (eLND) provides important prognostic information, which surpasses any current imaging modality (Figure 28.21). There is, however, no consensus about when it is indicated and to what extent. According to nomograms, patients with PSA <10 ng ml−1 and biopsy Gleason <7 have a low risk of lymph node metastasis, and therefore, eLND may not be beneficial. However, it should be noted that most nomograms are based on limited eLND (i.e. obturator fossa and external iliac vein), resulting in an underestimation in the incidence of patients with positive lymph nodes [150]. The removal a greater number of nodes results in improved staging. Greater than two nodes involved is an independent predictor of poorer PC‐specific survival with a 1.9‐fold relative increase in risk of PC‐specific death [151]. Figure 28.21 The sleeve of nodes is teased off the internal iliac vessels. Haemostasis is obtained by ligation, clips, or diathermy. eLND includes removal of nodes overlying the external iliac artery and vein, nodes within the obturator fossa, and nodes medial and lateral to the internal iliac artery. Some templates include clearing the common iliac nodes to the ureteric crossing, suggesting 75% of all anatomical landing sites are cleared with this approach [152]. Some studies suggest a therapeutic impact of eLND in a subset of patients with limited lymph node metastases, with improvements in biochemical disease‐free survival (BDFS) in patients with intermediate‐ and high‐risk disease [153–155]. When comparing eLND to limited LND, threefold higher complication rates have been reported in some studies [156]. Complications include lymphocele, lymphedema (scrotal and or lower limb), deep vein thrombosis, and pulmonary embolism. Radiation treatment for PCa goes back for nearly a century. Two types of radiotherapy are used for PC: (i) external beam radiotherapy (ERBT) or (ii) interstitial irradiation (brachytherapy). With no high‐quality head‐to‐head comparative trials, radiotherapy is an important alternative to surgery for curative therapy. Standard EBRT regimes allow the delivery of 65–70 Gy to the prostate, in rectangular fields (as determined by bony landmarks from CT), with minimal to no blocking and small boost fields. Unsurprisingly up to 41% of patients may have inadequate coverage of the target volume. Improvements in imaging and treatment planning (three‐dimensional, conformal radiation therapy [3D‐CRT] and intensity‐modulated radiation therapy [IMRT]) has allowed for better targeting and conforming of the treatment to the prostate, facilitating the use of increased doses, whilst limiting the toxicity secondary to an excessive dosage to surrounding normal tissues. These innovations have resulted in dramatic improvements in both acute and late toxicity of radiotherapy and improved cancer control compared with standard EBRT. The use of dose escalation, whole pelvic radiation (including regional lymph nodes) and androgen deprivation (i.e. neoadjuvant, concurrent, and adjuvant) has improved the results of radiotherapy in intermediate and high‐risk locally advanced PCa. Short‐term (three to six months) neoadjuvant (three months prior) and concurrent (three month after) androgen deprivation is recommended for those with intermediate‐risk disease, whereas those with high‐risk disease should receive neoadjuvant (three months prior), concurrent, and long‐term adjuvant (24 months after) androgen deprivation [157–159]. Side effects of radiotherapy are related to urinary (e.g. urgency, frequency, and haematuria), bowel (e.g. diarrhoea, rectal bleeding, and tenesmus), and gradual onset sexual dysfunction. The impact on sexual function may not be apparent for upto two years and are often exacerbated with the concurrent use of androgen deprivation [160]. Long‐term risks include urethral stricture, recto‐urinary fistula, and radiation cystitis, which although uncommon can be severely debilitating for the patient and offer complex management challenges for the physician. As with all radiotherapy, there is an increase in secondary cancers (1 in 300); with prostate EBRT, there is a doubling of the risk of rectal cancer and bladder cancer starting 10 years after prostate radiotherapy [161]. Therefore, pelvic radiotherapy is contraindicated in patients who have had previous pelvic radiotherapy, severe LUTS, and inflammatory bowel disease. Standard radiotherapy delivers treatment for PCa over seven to eight weeks using 2 Gy per fraction. This is considered as conventional fractionation. The radiobiology of PCa clearly suggests that higher doses per fraction can potentially be more effective and improves on local control rates due to increased sensitivity to fraction size. Stereotactic ablative radiotherapy (SABR) refers to the use of higher dose per fraction over a much shorter period of time (hypofractionation), which enhances tumour control and is certainly more convenient to patients as well. This technique delivers highly conformal radiotherapy over shorter period, which can now be achieved safely with the use of better on‐treatment imaging and image‐guided radiotherapy [41, 162, 163]. In the past, free‐hand seed placement techniques were associated with high rates of treatment failure, resulting in this technique falling out of favour. The accurate placement of the radioactive seeds under TRUS guidance has coincided with an increase in the popularity of the procedure. Radioactive implants are either permanently placed in the prostate (I125 half‐life 60 days, or Pd103 half‐life 17 days [164]) or radiation can be delivered via hollow‐core catheters (Ir192) attached to temporary implants for the duration of hospitalisation. Permanent implants have a lower dose rate (LDR), but a higher total dose delivered, compared to temporary implants which have high dose rates (HDR) and lower total doses. Eligibility criteria for permanent seed implantation [165]: Some patients experience significant urinary complications, such as urinary retention (1.5–22%), with post implantation TURP required in up to 8.7%, and urinary incontinence (0–19%) (increased risk with previous TURP) [166]. The incidence of grade III toxicity is less than 5%. Erectile dysfunction develops in about 40% of patients after three to five years. Therefore, contraindications include moderate (IPSS 8–19) to severe (IPSS >20) LUTS (due to increased risk of retention), previous TURP, and >50 cm3 prostate volume due to difficulty in seed placement. Patients with low‐risk PCa are the most suitable candidates for lower‐dose rate brachytherapy. EBRT is often given to those with intermediate‐ and high‐risk cancers. As opposed to EBRT, androgen deprivation does not appear to improve the outcomes of men with low‐ and intermediate‐risk disease being treated with brachytherapy [167]. Androgen deprivation can be used to shrink the prostate prior to brachytherapy to facilitate seed placement, with the caveat of additional side effects (Table 28.8) [168]. For nonsurgical intervention, patients with high‐risk PCa tend to be managed by EBRT and adjuvant androgen‐deprivation therapy (ADT). Table 28.8 Side effects of ADT and their prevalence. ADT, androgen‐deprivation therapy; BMD, bone mineral density; DEXA, Dual X‐ray absorptiometry; PC, prostate cancer; PDE5, Phosphodiesterase type 5; SERT, Selective oestrogen receptor modulator; SSRIs, selective serotonin reuptake inhibitors; SSNRIs, serotonin‐norepinephrine reuptake inhibitors. A word of caution on monitoring patients after radiotherapy: while checking the PSA, one might notice a rise in PSA after the NADIR. This is not recurrence, but coined ‘PSA bounce’ which occurs around nine months post‐RT, but can occur anytime for the first two years. The PSA level is usually <1.5 ng ml−1. Men with PCa that is clinically staged as T3a (locally advanced), Gleason score 8–10, or PSA level greater than 20 ng ml−1 are considered at high risk of recurrent disease despite definitive radical treatment. Treatment for patients with locally advanced PCa should take into consideration patients’ comorbidities, general health, and life expectancy. After careful staging with pelvic MRI and bone scintigraphy, patients may be considered for the following treatment options. The preferred treatment option is 3D‐CRT (or IMRT) with daily image‐guided RT (IGRT) in conjunction with long‐term ADT for two to three years. Combining ADT with RT has been comprehensively demonstrated to improve disease‐specific survival and OS compared to single‐modality treatment: (i) Two randomised phase III trials evaluated long‐term ADT with or without radiation in mostly T3 patients [1, 36]; (ii) 415 patients were randomised to either EBRT alone or EBRT plus three‐year ADT [160]; (iii) In the RTOG 8531 study, 977 patients with T3 disease were treated with RT with either immediate adjuvant ADT or delayed ADT at disease relapse [161]. Furthermore, increasing evidence favours long‐term over short‐term adjuvant (following neoadjuvant or concurrent) ADT in patients with high‐risk disease. The European Organisation for Research and Treatment of Cancer (EORTC) 22 961 trial showed superior survival when 2.5 years of ADT were added to RT given with six months of ADT in 970 patients, mostly with T2c‐T3, N0 disease [173]. There are emerging data that associate lower biochemical failure rates with the addition of HDR brachytherapy boost to EBRT in patients at high risk [174, 175]. The practical principles for radiation therapy using IMRT and image‐guided RT techniques allow safer delivery of higher doses of radiation (74 Gy in 37 fractions most commonly used in the UK). For those with high risk of lymph node involvement (predicted risk of >15% using the Roach formula: 2/3 PSA + (10× [Gleason score‐6])), pelvic nodal irradiation using IMRT technique should be considered. Prostate RT accuracy is enhanced with the use of fiducial markers (such as implanted gold seeds) and tracking devices that facilitate image‐guided treatment to minimise radiation‐induced toxicity. Patients with high‐risk locally advanced PCa (cT3b–T4 N0 or any T, N1) have a significant risk of disease progression and cancer‐related death. The optimal treatment approach, therefore, often necessitates multiple modalities to achieve local control as well as controlling the highly likely microscopic disease. Neoadjuvant and adjuvant ADT with radiotherapy is commonly used in this setting but risk of recurrence is very high (>20% over approximately 10 years). Both ETORC [158, 169] and RTOG 92–02 studies evaluated long‐term OS and progression‐free survival (PFS) in such high‐risk localised disease with median follow up of 9.1 and 11.3 years, respectively. Both showed a benefit of combined ADT and radiotherapy with an increase in OS and PFS at follow up (40–58% increase in OS and 23–48% PFS for EORTC) [169]. ADT or RT of the primary tumour plus neoadjuvant, concomitant, or adjuvant ADT (of two to three years) are available options for patients with N1 disease on presentation [36, 41]. ADT as single modality (bicalutamide 150 mg daily) is considered to be an inferior and noncurative treatment option when compared to combination therapy (i.e. ADT and radiotherapy) and best suited for patients who are elderly and symptomatic or those unwilling to undergo EBRT [36, 41]. Surgical resection of locally advanced PCa is an option depending on the health status of the patient. There is no gold standard for locally advanced PCa because it requires multimodal therapy with either a curative aim or to improve local control, stay metastasis free, and for PC‐specific survival. RP for locally advanced PCa should ideally be combined with extended lymph node dissection in patients with a life expectancy of >10 years and with minimal comorbidities [170]. RRP for locally advanced PCa has recently shown promise and has reported equivalent outcomes to open surgery [171]. Relapse after radical therapy either radiation or surgery is a salvage treatment modality. Combined treatment at the time of radical therapy (usually within six months) is considered an adjuvant treatment modality. Despite the earlier nature of adjuvant radiotherapy compared to salvage radiotherapy after radical prostatectomy biochemical relapse‐free survival (BCR) is not significantly changed. Briganti et al. performed a randomised trial of adjuvant versus early salvage radiotherapy (eSRT) (SRT for PSA rising but ≤2 ng ml−1) and showed no significant difference between patients treated with observation and eSRT versus adjuvant RT (≤6 months post surgery) [172]. Therefore, eSRT for biochemical recurrence following surgery given before the PSA rises above 2 ng ml−1 is as effective as adjuvant radiotherapy in patients undergoing RP for locally advanced high‐risk PCa. However, current European Association of Urology (EAU) recommendations define a rising PSA cut‐off of ≤0.5 ng ml−1 to initiate eSRT post surgery for high‐risk locally advanced disease to provide approximately 80% chance of being progression free at five years [173]. There currently is no imaging modality that can effectively detect recurrence or relapse, post‐RP. Furthermore, biopsy of the pelvic prostatic bed is not common practice. Therefore, recurrence or relapse is considered as any rise in PSA >0.2 ng ml−1. Subsequent treatment options include observation, radiotherapy, or ADT. Good response to salvage radiotherapy is seen in patients with a low‐grade or low‐stage disease from the start, PSA rise >1 year after prostatectomy, doubling time >12 months, and PSA is <1 ng ml−1. Generally, a period of surveillance is offered, and radiotherapy is given when the PSA is rising but <0.5 ng ml−1. For a positive surgical margin, radiotherapy is given once PSA is between 0.5 and 1 ng ml−1 after a period of surveillance. If lymph node dissection yielded nodal involvement, options are either early or delayed hormonal therapy. Treatment failure is defined as a rise in PSA by 2 ng ml−1 from the nadir (the lowest recorded PSA after treatment). ADT is the mainstay of salvage therapy post‐RP, either immediate or delayed until symptomatic. However in selected patients, salvage radical prostatectomy can be considered. Alternatively, brachytherapy can be offered; however, repeat biopsies will be required to demonstrate viable cancer cells. Metastatic PC (mPC) currently has an incidence of approximately 10% at diagnosis from European Randomised Study of Screening for Prostate Cancer (ERSPC) data [174]; this depends on the level of PSA screening which is discussed further in Section 28.11.1. An autopsy study in the year 2000 of 19 316 routine autopsies in men older than 40 years detected PCa in 8.2% (1589). This study showed that 35% of PCa detected was metastatic at autopsy and in most (90%) cases in bone. The next most common sites were lung (46%) followed by liver (25%), pleura (21%), and adrenal glands (13%). Interestingly, an inverse relationship was seen between lung and bone metastasis, suggesting independent mechanisms of metastatic spread. More recently phylogenetic mapping of mPC has revealed the complexity of the evolution of mPC using deep genome sequencing, which is also discussed further in Section 28.11.5.7 [175]. As with normal prostate epithelium, mPC depends on dihydrotestosterone (DHT), the active form of testosterone. Testosterone is made in the Leydig cells of the testicles, but the adrenal glands secrete an additional amount of steroid precursors, which are converted to testosterone by the enzyme 5‐alpha reductase expressed in prostate cells (Figure 28.22). The active DHT interacts with the androgen receptor (AR) present in normal and PCa cells. The AR translocates to the nucleus after binding DHT and dimerises to act as a potent transcription factor directly binding to DNA at many androgen‐response elements (AREs) and driving the transcription of essential genes for growth and survival in normal and mPC cells. Figure 28.22 The pituitary secretes luteinizing hormone‐releasing hormone (LHRH), which governs the secretion of the testes and adrenals. In 1941, Huggins and Hodges reported the ‘dramatic clinical effects of suppressing serum testosterone levels’ by surgical (bilateral orchiectomy) or pharmacological castration (diethylstilboestrol [DES], which suppresses luteinising hormone‐releasing hormone [LHRH] production at the hypothalamus) (Figure 28.22) in men with advanced PCa [176]. They were awarded the Noble Prize in 1967 for establishing the concept of ADT, which remains universally accepted as the first‐line treatment for symptomatic mPC. National Institute for Health and Care Excellence (NICE) and the European Society for Medical Oncology (ESMO) guidelines also recommend ADT to be used as a neoadjuvant therapy for four to six months in patients receiving radical radiotherapy for high‐risk disease and should also be considered for intermediate‐risk disease. This should be followed with two to three years of adjuvant ADT in patients having EBRT for high‐risk disease. Neoadjuvant and adjuvant ADT is not routinely recommended for patients undergoing RP. In the 1970s, ADT was delivered as LHRH agonists, chronic use of which decreases luteinising hormone (LH) by desensitising pituitary LHRH receptors. The monthly depot of leuprolide was the first LHRH agonist shown to be as clinically effective as DES but with a lower incidence of cardiovascular toxicity. LHRH agonists are still the most commonly used method of ADT. Different preparations differ in their route of administration (i.e. intramuscular injection, subcutaneous injection, or implant) and frequency of administration (1–12 months). There is little difference in the side effect profiles between different LHRH agonists, with minimal survival differences between compounds [177]. ADT has numerous well‐recognised adverse effects, and it is essential that clinicians understand these side effects to limit treatment‐related morbidity (Table 28.8). Acutely, LHRH agonists stimulate the anterior pituitary gland to promote testosterone secretion from the testes (and to a lesser extent the adrenal glands), thus potentially resulting in initial ‘flare’ symptoms [178]. These symptoms may manifest, depending on the tumour burden of the individual patient. For example, if patient has spinal metastases, even a short‐term increase in tumour growth could have disastrous consequence of spinal cord compression. Anti‐androgens are, therefore, routinely given for a few weeks when starting LHRH analogues to prevent flare symptoms. Less acutely, side effects due to castration levels of testosterone include hot flushes, loss of libido, and erectile dysfunction. ADT decreases lean body mass and increases subcutaneous fat mass. Observational studies associate ADT with increased insulin resistance, cardiovascular disease, and development of the metabolic syndrome [179, 180]. Several studies show increased cardiovascular risk unrelated to the duration of ADT therapy [181, 182], yet a recent meta‐analysis found no increased risk of cardiovascular death [183]. Cardiac disease need not preclude the use of ADT; however, appropriate secondary preventative measures to lower cardiac risk should be considered [184]. Increased bone loss following ADT results from impaired bone remodelling. ADT causes up to 3–5% in the annual decrease of bone mineral density (BMD) [185], in a time‐dependent manner, resulting in sixfold increase in fracture rate associated with ADT‐induced osteoporosis. Supplementation of calcium and vitamin D should be utilised, as well as quantifying BMD before commencing ADT. In patients with PCa and osteoporosis, bisphosphonates should be used to decrease osteoclast activity, with a recognised improvement in BMD of 7% in one year [186]. Denosumab, a human monoclonal antibody directed at RANK ligand (a mediator of osteoclasts), reduces vertebral fractures by half, when compared to placebo [187], and has been found to delay skeletal‐related events (SREs) for longer than zoledronic acid. However, neither agent has been shown to prolong survival. Side effects include hypocalcaemia and osteonecrosis of the jaw [188]. Newer ADT drugs, such as LHRH antagonists (e.g. degarelix) bind competitively to the pituitary LHRH receptors, thereby reducing LH and testosterone levels without the risk of an initial testosterone flare. Degarelix is equally effective in maintaining testosterone suppression than LHRH agonist over a 12‐month period [189] and has a role in patients with metastatic PCa and impending spinal cord compression. It is administered as a monthly subcutaneous injection, and side effects include local injection site redness, pain and swelling, and liver enzyme abnormalities. Nonsteroidal anti‐androgens (e.g. flutamide, bicalutamide) block the effects of adrenal androgens dehydroepiandrosterone (DHEA) and androstenedione at the androgen receptor by competitive ligand binding in PCa cells. Their main side effects are gynaecomastia, diarrhoea, nausea, and fatigue. Bicalutamide monotherapy is not as effective as LHRH treatment for mPC; however, it is equivalent of no‐metastatic disease. NICE and ESMO guidelines support the use of bicalutamide (150 mg daily) in metastatic PCa if a patient wishes to maintain sexual function and understands that there is limited data on survival and progression outcomes. This is because nonsteroidal anti‐androgens do not inhibit at the pituitary level; therefore, serum testosterone is normal or higher. However, if sexual function is not maintained on bicalutamide, individual patients should be changed to LHRH‐based ADT. There is peripheral conversion of testosterone to oestrogen with their use which can lead to gynaecomastia with or without breast pain. Therefore, patients should be offered prophylactic radiotherapy to breast buds to prevent gynaecomastia within the first month of starting bicalutamide monotherapy. If this is unsuccessful, weekly tamoxifen should be considered. Steroidal anti‐androgens also inhibit pituitary via negative feedback. Cyproterone acetate can also be used to prevent LHRH‐associated flares, but also help with side effects such as the hot flushes associated with castration. However, are associated with cardiovascular side effects. Scheduling of ADT has also been considered. Due to the adverse effects of ADT and the onset of castration resistance, intermittent therapy is believed to allow hormonal balancing during alternating androgen blockade and treatment ‘holiday’ (or recovery). Although there is no evidence of a survival inferiority in intermittent ADT compared to continuous ADT (mainly in metastatic cases), additional longer‐term outcome data is required [190]. In accordance with NICE guidelines, men considering intermittent ADT need to be counselled about the limited evidence for reduction in side effects and possible effect on progression of PCa. PSA should be measured every three months and ADT should be restarted if PSA ≥10 ng ml−1 or if symptomatic progression. The timing of commencing ADT remains unresolved. In locally advanced disease not amenable to local therapy, immediate ADT is justifiable in those with a high initial PSA (>50 ng ml−1) or a PSA doubling time of <12 months to delay progression complications [191]. After BCR following radical treatment, ADT should only be commenced with symptomatic local disease progression, any proven metastases, or PSA doubling time of less than three months (NICE guidelines) [40]. LHRH agonists (or antagonists) and AR antagonists can be combined to provide what used to be referred to as ‘maximal androgen blockade’ (MAB). Given the advent of the new drugs enzalutamide and abiraterone, it seemed unlikely that bicalutamide plus LHRH is ‘maximum’. Hence the terms ‘dual androgen blockade’ (DAB) and ‘combined androgen blockade’ (CAB) are considered more accurate descriptions of the practice. Nearly 25% of men will benefit from adding bicalutamide 50 mg to the LHRH injections for CAB. NICE guidelines do not recommend combined ADT as first‐line treatment for men with mPC. However, as a second‐line treatment, CAB improves survival at five years by 5% compared to LHRH agonist therapy alone in men with advanced disease [192]. The additional treatment is not without side effects, and patients may experience decreased quality of life due to impaired cognitive function, thermoregulation disruption, and sexual function [193]. It has been reported that in up to 25% men when their PCa stops responding to CAB, stopping the anti‐androgen drug, stops the tumour growth for a short time. This has been called anti‐androgen withdrawal effect for which the mechanism is not fully understood but is likely related to mutations in the ligand binding domain of the AR, thus switching the AR antagonists (such as bicalutamide) from inhibiting to activating the AR [194]. Corticosteroid such as dexamethasone (0.5 mg daily) can be offered as third‐line hormonal therapy after combination ADT. After a variable period of ADT, patients eventually progress to CRPC. The main driver for cancer progression in CRPC is persistent activation of the AR signalling axis. ADT should be continued in CRPC, and these patients should be offered further hormone therapies or if a poor response to initial hormone therapy, chemotherapy or clinical trials can be considered. A new generation of AR‐targeting agents has been developed, including abiraterone acetate (which inhibits CYP17, the rate‐limiting enzyme involved in androgen biosynthesis) and enzalutamide (which inhibits AR translocation into the nucleus). Abiraterone, in combination with prednisolone, was approved for treatment of asymptomatic and mildly symptomatic metastatic CRPC [184]. It has to be taken with prednisolone to avoid serious side effects associated with reactive mineralocorticoid excess (e.g. hypokalaemia, hypertension, and cardiac failure), and in combination with LHRH analogues. Alone abiraterone does not stop the testicular production of testosterone. Abiraterone treatment increased patient OS by 3.9 months following disease progression despite previous docetaxel treatment [195]. Enzalutamide (ENZ) acts as an AR inhibitor, with greater affinity to AR than bicalutamide [196]. For patients with mPC, ENZ improved the OS by 5.8 months, with additional improvements in time to PSA rise (biochemical progression) and skeletal events compared to placebo. Side effect profiles for ENZ and abiraterone are similar, with hot flushes, back pain, fatigue, nausea, and diarrhoea the most common complaints. Abiraterone also causes hypertension and peripheral oedema, whereas ENZ can cause dizziness and rarely, seizures. Both abiraterone and enzalutamide are oral medications. Compounds which imitate the action of LHRH agonists boost the output of LH by the pituitary until it is exhausted. For about a week the testicles and adrenals are in overdrive, and there is a surge in the level of testosterone in the blood which produces a flare of symptoms which can sometimes be serious (e.g. paraplegia) [41, 197]. The results of treatment with LHRH agonists are equivalent to orchedectomy. During the first week of treatment with LHRH agonists, the side effects of the surge in testosterone level may be prevented by androgen receptor blockers [41, 197]. LHRH antagonist (i.e. degarelix) [189] therapy is a new generation of hormonal therapies, which avoids the flare phenomena and does not require short‐term anti‐androgen cover. Orchiectomy is an equally effective option and should be considered as an option in metastatic disease, provided it is acceptable to patient. In a population‐based study, ADT with a LHRH agonist was associated with an increased risk for individuals to develop diabetes (hazard ratio [HR], 1.44; P < 0.001), coronary artery disease (HR, 1.16; P < 0.001), and myocardial infarction (HR, 1.11; P = 0.03) [183, 198, 199]. Compared to LHRH agonists, treatment with LHRH antagonist halved the number of cardiac events experienced by men with pre‐existing cardiovascular disease during the first year of ADT [200]. This is an irreversible and, compared with the other options, cheap form of ADT. Through a transverse scrotal incision one testis is delivered, the tunicae are incised, the testicular tubules are wiped away, and haemostasis is obtained by suture and diathermy (Figure 28.23). The same is done on the other side. Great care must be taken in regard to haemostasis to prevent a haematoma. It can be done under local anaesthesia using bilateral inguinal blocks and infiltration of the scrotal skin. Figure 28.23 Subcapsular orchidectomy. These compounds are known as anti‐androgens outlined previously. There are two main types. The first are the steroids cyproterone acetate and megestrol acetate. These prevent flare in the first couple of weeks of treatment with LHRH agonists and also subsequently prevent the tiresome side effect of hot flushes, seen either with orchedectomy or LHRH treatment. The second are nonsteroidal compounds such as flutamide, nilutamide, and bicalutamide. Used on their own, the anti‐androgens can have a therapeutic benefit that compares reasonably with orchiectomy, but when given to the patient whose metastases have escaped from the effect of orchiectomy or LHRH agonists, they have little effect. Orchiectomy lowers the plasma testosterone by more than 90%, but it only reduces the level of testosterone in the prostate by about 75%. The residual testosterone is derived from adrenal precursors dehydroepiandrosterone and androstenedione which are converted in the cell to testosterone. (For a time in the 1960s, adrenalectomy was widely used for clinical relapse after orchiectomy, but the results did not justify the morbidity of the operation.) DAB and CAB refer to the use of medical or surgical castration together with anti‐androgen. No survival advantage of this approach has been shown by any prospective randomised trials. However, meta‐analysis suggested that non‐cyproterone acetate anti‐androgens such as bicalutamide may provide an additional OS [201, 202]. Oral oestrogen (e.g. DES) was used previously as a method of ADT and is as effective as orchiectomy or LHRH agonists in producing castration levels of testosterone. It also avoids some of the side effects associated with other hormonal therapies such as osteoporosis, osteoporotic fractures, and hot flushes, but it is not used routinely as first‐line therapy because of the increased incidence of cardiovascular complications [203]. The Scandinavian Prostatic Cancer Group‐5 trial, involved slightly more than 900 patients randomised to either polyestradiol phosphate (PEP) intramuscular injection or ADT (orchiectomy or triptorelin + flutamide) [204–206]. Most recent analysis shows no difference in OS or disease‐specific survival and no difference in cardiovascular‐symptom mortality. This approach is currently been explored using oestradiol transcutaneous patches (PATCH trial MRC PR09 trial) [207]. Combined use of new agents like Abiraterone or ENZ in patients with hormone‐sensitive disease has recently been evaluated within the context of clinical trials (CHAARTED, USA and STAMPEDE, UK) [208, 209]. These studies assessed the role of chemotherapy (Docetaxel) in conjunction with ADT in hormone‐sensitive disease and have shown that upfront treatment with Docetaxel provides an OS over standard of care (ADT alone) of 13.6 months further increased to 17 months in a subgroup analysis of patients with high volume mPC. Such a significant increase in survival prompted recommendations that upfront Docetaxel with ADT has now becomes the new standard of care for high volume mPC. CRPC occurs in response to ADT with castrate serum levels of testosterone (i.e. testosterone <50 ng dl−1 or <1.7 nmol l−1). It can be defined as three consecutive increases in PSA, at least one week apart, resulting in two 50% increases over the lowest PSA. As PSA is an androgen‐responsive gene, this clinical definition of CRPC relies on reactivation of AR signalling. Anti‐androgens such as bicalutamide can activate AR signalling in the case of mutant AR expression [194]. The initial response in DAB is withdrawal of the anti‐androgen for at least four weeks for flutamide and for at least six weeks for bicalutamide. PSA progression, despite consecutive hormonal manipulations or progressive metastatic lesions on bone scan or soft‐tissue lesions (nodes >2 cm in diameter), are all indicators of progression to CRPC. There are no clear prospective data from randomised trials to guide treatment for the group of patients with castration‐resistant nonmetastatic PCa (n‐mCRPC). The only randomised controlled trial in this setting evaluated the role of Denosumab. In a phase III randomised trial involving 1432 patients with n‐mCRPC, Denosumab was reported to delay bone metastasis by four months compared to placebo [210]. However, OS did not improve. The group with metastatic castration‐resistant PC (mCRPC) is more prevalent than the n‐mCRPC group with more than 85% of men who die of PCa having mCRPC to the bone [211]. The management of this group of patients has changed quite significantly over the last few years with a number of new agents demonstrated to positively impact OS (Table 28.9). Table 28.9 Timeline of clinical trials of therapy in advanced prostate cancer. Clinical trials of therapy in advanced metastatic prostate cancer showing how this area of clinical research has progressed rapidly in the last 10 years. AR, androgen receptor; CRPC, castration‐resistant prostate cancer; DES, Diethylstilbestrol; HC, hydrocortisone; LHRH, luteinising hormone‐releasing hormone; OD, once daily; PAP, prostatic acid phosphatase; QoL, quality of life. These options include: Radiation is an effective means of palliation for bone metastases. A short course consisting of a single 8 Gy is as effective as and less toxic than 30 Gy in 10 fractions [230], providing adequate therapy for most patients. Beta‐emitting radiopharmaceuticals are an effective and appropriate option for patients with widespread metastatic disease, particularly if they are no longer candidates for effective chemotherapy [231]. Because many patients have multifocal bone pain, systemic targeted treatment of skeletal metastases offers the potential of pain relief with minimal side effects. Unlike the alpha‐emitting agent radium‐223, beta emitters confer no survival advantage and are palliative. Radiopharmaceuticals agent that are available for the treatment of painful bone metastases are strontium‐89 (89Sr) and samarium‐153 (153Sm) [232]. Advanced disease can present with an obstructive uropathy which might require stent insertion. Nephrostomy of the resultant hydronephrosis, followed by antegrade stenting is the usual treatment path. Renal function should be checked and consideration for dialysis where applicable. Of course, taking into consideration whether invasive treatment is the best option for the patient (i.e. frailty and multiple comorbidities with or without dementia) as it might be best for complete supportive care with the understanding that the patient will die of their renal failure. Spinal cord compression, on the other hand, will need urgent treatment. Initial treatment includes analgesics and high‐dose steroids: dexamethasone 16 mg followed by 4 mg every six hours is given, while a more definitive treatment is organised. Spinal MRI is the investigation of choice. Either radiotherapy or surgical decompression should be performed within the first 24 hours of presentation. Treatment of PCa in this situation is in line with that of metastatic disease; however, urgent subcapsular orchedectomy or degeralix can be used to ensure castration levels of testosterone are reached sooner rather than later. Invariably signs of cord compression or imminent compression are the first presentation of PCa. These include, new onset back pain, which can worsen by straining or coughing, alteration of sensation below the compression root with lower limb muscle weakness affecting mobility, incontinence of stool or urine or both, or acute urinary retention. Supportive care is an essential part in managing metastatic and treatment‐resistant PCa. With improving treatment options and better survival for these patients, more and more patients are living longer with PCa and the delayed or long‐term side effects of the treatment they have received. The most common needs are fatigue, pain, nausea, vomiting, nutritional issues, and breathlessness. Palliative radiotherapy and radiopharmaceuticals are good options for pain control. Bisphosphonate, as indicated previously, can provide further improvement in quality of life through prevention of SREs. Other therapeutic options should be considered including physiotherapy and social and psychological supports are all an important part of a comprehensive package of care that is often required. A limited or ‘channel’ TURP can be offered for bothersome LUTS. Many patients have severe outflow obstruction, and even though there is evidence of widespread metastases, TURP is needed to give symptomatic relief. In such cases, the technique is somewhat different from that used in BPH. When there are widespread metastases, there may be abnormal circulating fibrinolysins, leading to clotting disorders, and if there is any suggestion of spontaneous bruising, it is wise to obtain expert haematological advice. Tranexamic acid may be required. The prostatic urethra may be so stiff and rigid that passing the resectoscope risks creating a false passage. A useful trick is to pass a filiform guide first to guide the resectoscope sheath through the rigid cancer. Once the first few chips have been removed, the resectoscope becomes mobile. The aim of the operation is not to take out all the tumour down to the capsule because most cancers originate in the peripheral part of the prostate. The aim is to cut out a generous cone of tissue with its apex at the verumontanum (Figure 28.24). Keep well away from the region of the sphincter, which may already be invaded by cancer. Resecting in its vicinity may bring on incontinence, which is more commonly seen after TURP in patients with PCa. Figure 28.24 The aim of transurethral resection of prostate (TURP) in prostate cancer is to cut out an even cone. Screening for a disease is only worthwhile if the benefit outweighs the risk of harm associated with diagnosis and proposed intervention. PSA screening for PCa diagnosis remains highly controversial. The natural history of PCa is also highly variable, ranging from an indolent nature to an aggressive life‐shortening disease. In 1968, Wilson and Junger developed 10 guiding principles for WHO for overseeing a national screening programme (Table 28.10). Table 28.10 Wilson & Junger WHO criteria for disease screening [233]. PCa only meets one of these principles: The condition is an important health problem. In the UK, as in many countries worldwide, PSA screening is not routinely offered to men. However, early detection through the primary care–based Prostate Cancer Risk Management programme is considered beneficial. Introduced in 2002, it provides patients with clear information regarding investigations, treatment, and the long‐term implications of a diagnosis to allow patients to make an informed choice [234]. The 2014 EAU guidelines advise an individualised strategy with early testing of those who are at risk (i.e. increasing age, family history, Afro‐Caribbean ethnicity) [173]. The European Randomised Study of Screening of Prostate Cancer studied 160 000 men and found at 11 years screening reduced mortality by 21%. Screening was particularly useful in men ages 55–69. However, there was a high level of overtreatment of cancer. The number needed to screen to prevent one death from PCa at 11 years of follow‐up was 1055 men. An additional 37 cancers would need to be detected to prevent one death from PCa [235]. In 2012, the Prostate, Colon, Lung and Ovarian (PLCO) study published their results into randomised screening or standard review of over 76 000 men. At 13 years, there was no evidence of mortality benefit from screening PC; however, the results were potentially flawed as many of the nonscreened arm had their PSA checked out with the study [236]. One reason screening has not been introduced is that we do not know which treatment modality is best suited for individual patients. The Prostate Testing for Cancer and Treatment (ProtecT) study was set up to evaluate treatment options for early PCa. Its primary aim is to assess the clinical effectiveness, cost‐effectiveness and acceptability of active monitoring, radiotherapy or radical prostatectomy in men with localised PCa identified through population based PSA testing [237]. Furthermore, Research Plan CAP (Comparison Arm for ProtecT) is a current Cancer Research UK randomised controlled trial evaluating population‐based screening for localised PCa in the United Kingdom; 587 general practice providers in eight centres in England and Wales have been allocated to either population‐based PSA testing (screened) or standard practice (unscreened) and the results are awaited [238]. A 2013 Cochrane review of screening showed that PSA testing increased diagnosis and identification of localised PCa with an unknown impact on quality of life. The review was first undertaken in 2006, but there was insufficient evidence to either support or refute screening. A 2010 update of the review looked at five randomised controlled trials, totalling 321 342 men between 45 and 80 years old, all of whom received a PSA test with or without DRE and were followed up for between 7 and 20 years. Meta‐analysis showed no statistically significant difference in disease‐specific mortality between the screened and control groups. It concluded that the diagnosis of PCa was greater in the screened group and that screening overall does not significantly reduce disease‐specific death [239]. In 2014, the findings of a Swedish population‐based cohort study was published which showed men with a PSA >2 ng ml−1 at 60 years old benefited from ongoing PSA screening, whereas men with PSA <1 ng ml−1 had no benefit from screening [240]. In summary, PSA screening continues to be a controversial topic within urology with ongoing research needed to fully assess the risks versus benefits. Prostate biopsies are required to obtain tissue for histological diagnosis. They are usually performed with local anaesthetic but can also be performed under general anaesthesia (Figure 28.25). Since the 1980s, TRUS‐guided prostate biopsy has been widely accepted in clinic practice and is recommended as first‐line biopsy technique by NICE [40]. Controversy arises around the low diagnostic yield of the initial biopsy (35% maximum) and significant yet low 1 in 500 risk of death post‐TRUS biopsy from associated sepsis. Increasing requirement for biopsies due to PSA testing has made this side effect a necessary consideration to be discussed with patients prior to their consent [173]. Figure 28.25 Diagram showing the different routes of prostatic biopsy and the ease of sampling the anterior prostate with a transperineal template biopsy compared to a transrectal biopsy. To avoid false‐negative biopsy results, there has previously been a trend to repeat TRUS biopsies if earlier biopsies were negative. Such repeat biopsies often have reduced detection rates [62]. The 2014 PCa NICE guidelines advise the consideration of multiparametric MRI (T2W and DWI) for men with a negative TRUS biopsy to determine if another is needed [40]. Further biopsies should only be performed if the MRI is positive or if there is still a risk that cancer is present; for instance, if there has been previous high‐grade PIN (HGPIN), ASAP, or an abnormal DRE. If subsequent biopsies are warranted, then transperineal template biopsies can obtain a greater number of cores in a systematic manner, sampling the anterior prostate easily. The transperineal route predates the transrectal route for prostate biopsy, but in the late 1990s, it was combined with the template technique for insertion of brachytherapy seeds and found to be a precise technique to systematically sample the whole gland. The biopsies are performed under general anaesthetic with antibiotic cover via a template guide on the perineum that correlates to an ultrasound image (Figure 28.10). Up to 50 systematic biopsies can be taken of the prostate via the template grid at 5‐mm intervals. This can aid diagnosis of PCa, or offer reassurance to patients if histology remains negative. The drawback of such a large number of biopsies is the subsequent inflammation or swelling with risk of acute urinary retention (1.9%) and significant fibrosis, making future RP difficult. On the other hand, the transperineal route has a lower risk of sepsis than the transrectal route [241]. Functional MRI parameters such as DWI are increasingly incorporated as part of the routine MRI staging protocol, leading to increased diagnostic accuracy [52]. Multiparametric MRI (mpMRI) appears to be able to exclude clinically significant cancer with a high negative predictive value and specificity of more than 90% [242–244]. This has led to recent recommendation by NICE that it can be used to determine whether repeat biopsy is necessary in patients with an initial negative prostate sampling [40]. mpMRI has also been suggested as the method of AS [245, 246] with the potential of minimising overtreatment and facilitating cost‐effective management of these patients [247, 248]. The validity of these assumptions still has to be assessed by ongoing studies. Whatever the outcome, the addition of a large number of MRI examinations will have important financial impact. The accepted standard 10‐ to 12‐core TRUS‐guided biopsy has a good negative predictive value for cancer but can also lead to the detection of a number of low‐volume low‐risk disease. In addition, the standard biopsy approach is poor at sampling tumours at the anterior, midline, and apex of the prostate, leading to underdiagnosis of clinically significant disease. MRI, as the best imaging modality for demonstrating the prostate anatomy, is most suited method for image‐guided biopsy. This can be done as MRI‐guided TRUS biopsy, in‐gantry real‐time MRI‐guided biopsy, or MRI‐TRUS fusion‐guided biopsy. The advantage of the first method is that it does not require any specialised equipment except access to an MRI machine or change in biopsy technique. The disadvantage is the uncertainty of obtaining the biopsy from the suspicious region. The in‐gantry method is the most accurate, but it is time consuming and resource intensive. The MRI‐TRUS fusion technique is a hybrid of the two previous methods. A designated software is used to co‐register the MRIs onto the corresponding ultrasound images which are then used to guide a real‐time TRUS biopsy. This technique produces fairly accurate sampling, although error can be introduced as a result of deformation of the prostate during TRUS imaging. A recent systematic review has shown that MRI‐guided TRUS biopsy does not just increase the cancer detection rate, it also reduces the number of cores required to make the final diagnosis and at the same time, favours the detection of clinically significant cancer [249]. A biomarker (or biological marker) is broadly a measurable response to a biological process [250]. For PCa, this is the progression to adenocarcinoma from normal prostatic epithelium. Since the advent of PSA, a number of biomarkers have been proposed as clinically relevant for various stages of PCa. PSA has been the major clinical biomarker used in early PCa since the 1990s. The abundance of various forms of PSA becomes easier to understand with knowledge of the normal physiology of PSA. PSA can be bound to serum proteins (complexed) or in its free form (fPSA). PSA or KLK3 is part of the Kalikrein family of proteins which cleave peptide bonds. PSA exists in an inactive proPSA form with additional amino acids that prevent its folding into active PSA. When cleaved by KLK2, PSA becomes active. Forms of proPSA are denoted by the number of extra amino acids (i.e. [−2]proPSA). This name was later shortened to p2PSA as it was the isoform found in high amounts in peripheral zone PCa and was also inactive (iPSA). p2PSA measurement provides the basis of the prostate health index (PHI) which is a formula including fPSA, tPSA, and p2PSA (PHI = p2PSA/fPSA × √tPSA). The PHI value has been suggested to outperform the standard tPSA in detecting PCa (Table 28.11) [251]. Table 28.11 Serum and urine biomarkers in prostate cancer and their description including a comparison of receiver operating characteristic (ROC) analysis by area under curve (AUC) for each test or biomarker. PSA, prostate‐specific antigen. C‐reactive protein (CRP) has been recognised as a marker of poor prognosis in high‐risk and locally advanced disease treated with radiotherapy independent of Gleason grade and PSA [253]. Interleukin‐6 (IL‐6) levels have also been suggested as a biomarker in later high‐grade disease as a marker of cancer associated inflammation and the anti‐IL6 monoclonal antibody siltuximab is in phase II trials [254]. Prostate cancer antigen 3 (PCA3) is a long noncoding RNA gene found to be specific for PCa. Urine detection tests have shown some promise in predicting biopsy result [252]. The detection of the TMPRSS2:ERG fusion gene (a common translocation event in PC) in urine has also been suggested as a potentially additive biomarker in early PCa [255]. Neutrophil‐to‐leucocyte ratio (NLR) rises in late mCRPC and can predict outcome along with a number of standard clinical tests [256]. The presence of liver metastasis, Hb <12 g dL−1, ALP >2 × upper limit of normal (ULN), LDH > 1.2 × ULN and NLR >3 have been combined into a prognostic score for mCRPC [195]. Combining standard parameters such as lactate dehydrogenase (LDH) with the presence of circulating tumour cells (CTCs) has been shown as a surrogate marker for survival for mCRPC in the COU‐AA‐301 Abiraterone trial [257]. Circulating tumour‐specific DNA (i.e. genetic materials released by tumour cell into the peripheral circulation) can be used as a potential biomarker in later stage disease. DNA hypermethylation is commonly seen in PCa (and all cancers) as a mechanism of epigenetic silencing of tumour suppressors [134]. Methylated glutathione S‐transferase 1 DNA (mGSTP1) levels have shown promise as a prognostic biomarker for mCRPC [258]. Mechanistically, mGSTP1 can result in hypermethylation of CpG islands (targets for methylation) within gene promoters, occurring in most PCa [259]. These are phospholipid encapsulated vesicles released from tumour cells into blood and urine and contain a variety of lipids, proteins, and miRNA. Their detection can be exploited as potential prognostic markers in PCa [260]. Immunohistochemistry (IHC) staining of biopsy samples has become increasingly used in routine clinical practice (see Section 28.6.3). There have also been a huge number of proposed prognostic biomarkers published, but none have significantly added to clinical practice as yet. A recent study identified 2900 PubMed hits for ‘prostate cancer prognostic marker’, amongst which 28 IHC markers were further evaluated in a retrospective cohort of 238 RP samples with median follow up of 72 months. Out of the 28 markers, only 4 were verified to be statistically independent predictors of PSA relapse (AKT1, Stromal AR, EZH2 and PSMA) [122]. AKT1 also known as Protein Kinase B is a serine/threonine kinase constitutively active in many human cancers which plays a key role in PCa growth and survival [261, 262]. The loss of stromal AR along with high nuclear AR in tumour epithelium portends a poorer prognosis with increased relapse in early stage PCa [263]. EZH2 (also known as lysine N‐methyltransferase, a protein involved in histone methylation and gene silencing) is upregulated in PCa. Prostate specific membrane antigen (PSMA) is a membrane glycoprotein with high prostate specificity [264]. Except for AR staining, none of these biomarkers are currently incorporated into routine clinical practice. The genetics of PCa has been extensively examined with the advent of next‐generation sequencing (NGS) techniques. This has given us a deeper understanding of this complex and heterogeneous disease and more recently has allowed us to follow its natural progression through metastasis as never before [265, 266]. The PCa genome has surprisingly few mutations compared to other cancers at approximately 1 mb−1 [267]. The genetic landscape of PCa is that of genomic instability resulting in significant chromoplexy (e.g. the abnormal joining of chromatin from one or more chromosomes), resulting in some cases in a ‘gain of function’ fusion gene product, which provides survival advantage for certain cell clone(s). The most common fusion event in PCa is the TMPRSS2:ERG fusion resulting in ERG (an ETS family transcription factor) overexpression mediated by the androgen‐responsive element of TMPRSS2 [268]. ERG in this context is thought to mediate the invasive phenotype of PCa distinguishing it from high‐grade PIN (HGPIN) and may also play a role in activating biosynthetic pathways leading to CRPC [269]. Activation of the WNT/beta‐catenin pathway along with mTOR may also drive growth and metastasis in PCa [270]. Alongside gain of function alterations, ‘loss‐of‐function’ alterations in PCa include deletion of PTEN (a common tumour suppressor involved in most human cancers) and loss of P53 function evading apoptosis. Loss of regulators of the tyrosine kinase P13K/AKT growth and survival pathway have also been shown to be lost in a large proportion of localised disease and to a greater extent in metastatic disease [271]. NKX3.1 is a homeodomain transcription factor and androgen‐responsive element which has been shown to oppose the TMPRSS2:ERG fusion, which occurs in most PCa and its tumour suppressor role is also lost in most PCa [272]. The upstream cause of the genomic instability in PCa is thought to be a collection of epigenetic modifications including aberrant DNA hyper‐ and hypomethylation, histone remodelling, and miRNA expression, over time leading to the genetic alterations characteristic of PCa [273]. To date, the most common point mutation occurring in up to 15% of all PCa is in the substrate binding site of SPOP, a ubiquitin ligase which interacts with histone associated proteins [274]. Phylogenetic mapping of PCa has recently shown that the heterogeneity in localised tumour and metastasis is greater than previously hypothesised. In the case of localised multifocal disease, it has been shown that separate tumours arise spontaneously and regions surrounding the tumours may also bear characteristic driver mutations despite histologically appearing normal. Such field effects may have a profound impact on the use of focal therapy for PCa [175]. Metastatic lesions also have been shown to arise from parental metastatic lesions in a stepwise fashion rather than originating from the primary site [265]. There are multiple gene expression panels for risk stratification in PCa. These genetic tools have the potential to predict future outcome based on the retrospective transcriptional profiling of thousands of prostate samples using NGS techniques. Candidate genes, which correlate with outcome in such retrospective studies, have been incorporated into a number of screening panels which can be applied to initial prostate biopsies. Depending on the expression profile of the tumour, a theoretical risk of progression can be used to guide future management. These powerful tools, though expensive, provide a pathway to personalised management of PCa from the initial biopsy [275]. At the time of writing this chapter, examples of the commercially available gene expression profiling tests include Prolaris, Oncotype DX, and Decipher. Prolaris uses an RNA expression profile, whereas the others use a genomic DNA profile to risk‐stratify PCa. ConfirmMDX is another commercial test that assesses gene methylation patterns to look for PCa in a histologically negative biopsy [276]. The use of specific groups of genetic mutations to predict outcome of patients with early disease is a powerful tool, which may have a greater role in the future but requires careful validation in a heterogeneous disease such as PCa. HDR brachytherapy involves temporary insertion of a radiation source into the prostate and provides a boost dose in addition to EBRT for patients at high risk of recurrence. Combining EBRT (40–50 Gy) and HDR brachytherapy allows dose escalation while minimising acute or late toxicity in patients with high‐risk localised or locally advanced cancer [277–279]. Studies have demonstrated reduced risk of recurrence with the addition of brachytherapy to EBRT [280–282]. Common boost doses include 9.5–11.5 Gy in two fractions, 5.5–7.5 Gy in 3 fractions, or 4.0–6.0 Gy in four fractions. Cryotherapy is an evolving minimally invasive therapy for PCa through local or focal freezing. The role of cryotherapy as a primary modality is still not well established and is currently not recommended as a routine primary therapy due to limited long‐term data and lack of randomised trials [283]. Salvage cryotherapy may be considered as an option in patients who have local disease relapse following primary radiation therapy. The current standard of care for patients with radiation recurrent PCa is deferred ADT, an essentially palliative treatment option, making randomisation between an active and deferred treatment challenging [284]. The Cryo On‐Line Data (COLD) registry is a registry of prostate cryotherapy cases which aims to address the questions plagued by the ongoing difficulties around performing a randomised controlled trial in the focal therapy arena [285]. Therefore, current treatment needs to be individualised and patients have to be carefully selected including those with positive biopsy and low suspicion of metastatic disease [286]. High‐intensity focused ultrasound (HIFU) is another evolving technique for treatment of localised PCa. HIFU therapy is usually targeted at the part of the prostate containing the cancer inducing tumour necrosis and cavitation by its thermal effect. This treatment is currently not recommended as a primary therapy due also to lack of randomised controlled trials and long‐term data [287, 288].
Prostate Neoplasm
Abstract
28.1 Epidemiology
28.2 Aetiology
28.2.1 Nonmodifiable Risk Factors
28.2.1.1 Age
28.2.1.2 Family History and Genetics
28.2.1.3 Race
28.2.2 Modifiable Risk Factors
28.2.2.1 Diet
28.2.2.2 Body Mass Index
28.2.2.3 Hormonal Factors
28.2.2.4 Drugs
28.3 Clinical Features
28.4 Prognosis
28.5 Investigations
28.5.1 Prostate‐Specific Antigen
Age
PSA (ng ml−1) triggering referral
40–49
>2.0
50–59
>3.0
60–69
>4.0
>70
>5.0
28.5.2 PSA Density
28.5.3 PSA Doubling Time
28.5.4 PSA Velocity
28.5.5 Digital Rectal Examination
PSA (ng ml−1)
<4
4–10
>10
Normal DRE
<20%
25%
50%
Abnormal DRE
<30%
50%
>75%
28.5.6 Diagnostic Investigations
28.5.6.1 Transrectal Ultrasound Imaging
28.5.6.2 TRUS‐Guided Biopsy
28.5.6.3 Repeat and Saturation Biopsy
28.5.6.4 Incidental Finding in Positron Emission Tomography–Computed Tomography
28.5.7 Staging Investigations
T (Primary tumour)
TX
Primary tumour cannot be assessed
T0
There is no evidence of a tumour in the prostate
T1
Tumours are too small to be seen on scans or felt during examination of the prostate; they may have been discovered by needle biopsy, after finding a raised PSA level
T2
Tumours are completely inside the prostateTumour >7 cm but <10 cm in greatest dimension
T3
Tumours have broken through the prostate gland but not involving adjacent organs
T4
Tumours have spread into other pelvic organs.
N (Regional lymph nodes)
NX
Regional lymph nodes cannot be assessed
N0
No regional lymph node metastasis
N1
The tumour has spread to one or more lymph nodes in the pelvis
M (Distant metastasis)
Mx
Distant metastasis cannot be assessed
M0
No distant metastasis
M1
Tumour has spread outside the pelvis
28.5.7.1 Local Staging
28.5.7.1.1 Spread of Prostatic Cancer
Capsule
Lymph Nodes
Bone Invasion
Prostate Imaging Reporting and Data System Version 2 (PI‐RADS v2)
28.5.7.2 Lymph Node Staging
28.5.7.2.1 Distant Metastases
28.5.7.2.2 Imaging Possible Disease Relapse
28.6 Pathology, Histopathology, and Molecular Pathology
28.6.1 Histopathology
28.6.1.1 Prostatic Intraepithelial Neoplasia
28.6.1.2 Atypical Small Acinar Proliferation
28.6.1.3 Adenocarcinoma
28.6.1.4 Other PCa
28.6.2 Grading
28.6.2.1 Gleason Grading
28.6.2.2 Translating to an Updated Grading System
Gleason Score
New Gleason Grade
5‐year biochemical relapse‐free survival
6
1
96%
7 (3 + 4)
2
88%
7 (4 + 3)
3
63%
8
4
48%
9 and 10
5
26%
28.6.2.3 Concordance of Biopsy and Prostatectomy Grade
28.6.2.4 Grading after Therapy
28.6.3 Immunostains
28.6.3.1 Basal Cell–Associated Markers
28.6.3.2 Prostate Cancer–Associated Markers
28.6.3.2.1 AMACR (P504S)
28.6.3.3 Prostate Lineage–Specific Markers
28.6.4 Molecular Pathology
28.6.4.1 Potential Molecular Markers
28.6.4.1.1 Ki67/Mib1
28.6.4.1.2 p27
28.6.4.1.3 c‐MYC
28.6.4.1.4 Epigenetic Alterations
28.7 Management, Treatments, and Patient Selection
Level of risk
PSA
Gleason score
Clinical stage
Low risk
<10 ng ml−1
and
≤6
and
T1–T2a
Intermediate risk
10–20 ng ml−1
or
7
or
T2b
High risk
>20 ng ml−1
or
8–10
or
≥T2c
28.7.1 Low‐Risk Localised Prostate Cancer
28.7.1.1 Active Surveillance and Watchful Waiting
Active Surveillance
Watchful Waiting
Intent
Curative
Palliative
Follow‐up
Predefined schedule
Patient‐specific
Assessment/markers used
DRE, PSA, Re‐biopsy, (MRI)
Not determined
Life expectancy
>10 years
<10 years
Aim
Minimise treatment‐related toxicity without compromising survival
Minimise treatment‐related toxicity
Stage
Low and subset of patients with intermediate risk only
Can apply to patients of all stages
Timing
Method of surveillance
Throughout active surveillance monitor PSA kinetics including PSA velocity and doubling time
Start
Multiparametric MRI
Year 1
3–4 month PSA
6–12 month DRE
1 year rebiopsy
Year 2–4
3–6 month PSA
6–12 month DRE
Year >5
6 month PSA
12 month DRE
If concern about clinical or PSA changes at any time during AS then reassess with multiparametric MRI or rebiopsy.
28.7.2 High‐Risk Localised PCa
28.7.2.1 Radical Prostatectomy
28.7.2.1.1 Technique of Radical Prostatectomy
28.7.2.1.2 Complications
28.7.3 Role of (Pelvic) Lymph Node Dissection
28.7.4 Radical Radiotherapy
28.7.4.1 External Beam Radiotherapy
28.7.4.2 Stereotactic Ablative Radiotherapy
28.7.4.3 Brachytherapy
Timing
Side effects
Prevalence if known
Possible monitoring and treatment of side effects
Immediate
Flare Phenomenon – Bony pain, spinal cord compression
Unknown
Acute
(Few weeks)
Hot flushes (vasomotor flushing) – low testosterone interrupts negative feedback on hypothalamic noradrenaline production which resets hypothalamic thermoregulatory centre.
up to 80%
Sexual dysfunction – Loss of libido, shrinkage of genitalia
80–90%
Chronic (months – years)
Skeletal related events – loss of BMD is associated with length of ADT
20%
Decreased cognitive function – decline in memory and executive functioning
Unknown
Low mood and depression
Unknown
Metabolic / cardiovascular dysfunction – obesity, insulin insensitivitym and alters lipid profiles (increase cholesterol)
14–70%
Anaemia – reduced erythrogenesis
90%
Fatigue & lean muscle wasting
40%
28.7.5 Locally Advanced PCa
28.7.5.1 Radical External Beam Radiotherapy
28.7.5.2 Radical Prostatectomy
28.7.6 Recurrence after Curative Intent Treatment
28.7.6.1 Adjuvant versus Salvage Treatment
28.7.6.2 Salvage Radiotherapy Post‐Radical Prostatectomy
28.7.6.3 Salvage Therapy Postradical Radiotherapy (Androgen‐Deprivation Therapy or Salvage Prostatectomy)
28.7.6.4 Metastatic Prostate Cancer
28.8 Androgen‐Deprivation Therapy
28.8.1 Anti‐Androgens
28.8.2 Dual and Combined Androgen Blockade
28.8.3 Second‐Generation Anti‐Androgens: Enzalutamide and Abiraterone
28.8.4 Androgen‐Deprivation Therapy Options
28.8.4.1 First‐Line Androgen‐Deprivation Therapy
28.8.4.1.1 Luteinizing Hormone‐Releasing Hormone Analogues
28.8.4.1.2 Luteinizing Hormone‐Releasing Hormone Antagonist
28.8.4.1.3 Bilateral Subcapsular Orchiectomy
28.8.4.1.4 Androgen Receptor‐Blockers
28.8.5 Second‐Line Androgen‐Deprivation Therapy
28.8.5.1 Dual and Combined Androgen Blockade
28.8.6 Third‐Line Androgen‐Deprivation Therapy
28.8.6.1 Oestrogenic Therapy
28.8.6.2 Combined and Upfront Chemohormonal Therapy
28.9 Castration‐Resistant Prostate Cancer
28.9.1 Castration‐Resistant Nonmetastatic Prostate Cancer
28.9.2 Metastatic Castration‐Resistant Prostate Cancer
Year
Treatment
Types
Overall survival benefit (months)
Clinical trial major outcomes
1941
Bilateral orchidectomy
Surgical
–
Reduced PAP for mean 180 days in metastatic PCa [176, 212]
1967
Veterans Administration Cooperative Urological Research Group (VACURG) DES vs Orchidectomy
Surgical Castration vs Oestrogens
–
DES (1 mg or 5 mg) vs orchiectomy – 3 mg DES recommended to achieve castrate levels of testosterone and reduce cardiotoxicity
Reduced cardiovascular death with 1 mg DES [213].
1984
Leuprolide Study Group first monthly depot
LHRH analogue
–
Leuprolide vs DES 3 mg – equal efficacy for reducing serum testosterone to castrate levels but less cardiotoxicity [214].
1989
Goserelin (Zoladex) + Flutamide (EORTC 30853)
Maximum androgen blockade
–
1995
Bicalutamide vs Castration
AR antagonist
–
50 mg Bicalutamide OD vs castration – monotherapy improved QoL outcomes [215].
2003
Triptorelin vs Leuprolide
2nd‐generation LHRH analogue
–
Triptorelin 97% vs Leuprolide 90.5% survival at 9 months [177].
1999
Mitoxanthrone + HC vs HC alone for CRPC (cancer and leukaemia group B 9182 study)
Chemotherapy and palliation
–
Mitoxanthrone with or without HC for CRPC showed improvement in palliative end points but no survival benefit [216].
2004
Docetaxel (TAX 327)
Chemotherapy
2.9 m
Docetaxel + Prednisone vs Mitoxanthrone + Prednisone [217].
2010
Cabazitaxel (TROPIC)
Chemotherapy
2.4 m
Cabazitaxel + Prednisone vs Mitoxanthrone + Prednisone [218]
2010
Sipuleucel‐T (IMPACT)
Immunotherapy
4.1 m
Leucophoesis of antigen presenting cells and in vitro immunisation with recombinant PAP [219].
2013
Abiraterone (COU‐AA‐302)
Androgen synthesis blockade
4.4 m
Abiratarone + Prednisone vs Prednisone alone [220].
2013
Radium‐223 (ALSYMPCA)
Alpha emitter
3.6 m
Radium‐233 injection vs placebo for men who had received, were not eligible for or declined docetaxel [221].
2014
Enzalutamide (AFFIRM)
2nd‐generation AR antagonist
4.8 m
For docetaxel‐resistant CRPC enzalutamide treatment vs placebo, delayed time to first skeletal event by 3.4 m [222].
2015
ARN‐509 (SPARTAN) – recruiting
2nd‐generation AR antagonist
–
ARN‐509 (non‐ligand binding domain AR antagonist) vs placebo.
28.9.3 Palliative Management of Metastatic Castration Resistant Prostate Cancer
28.9.3.1 Palliative Radiotherapy
28.9.3.2 Emergency Management
28.9.3.3 Supportive Care
28.10 The Role of TURP for Voiding Symptoms Associated with PCa
28.11 Controversies, Cutting‐Edge Developments, and Hot Topics
28.11.1 PSA Screening
Criteria for disease screening (Wilson & Junger)
28.11.2 Biopsy Approach: Transrectal versus Transperineal
28.11.3 Role of Multiparametric MRI and MRI Fusion Biopsy
28.11.4 MRI‐Guided Biopsy
28.11.5 Emerging Biomarkers
28.11.5.1 Serum Biomarkers
Name
Site
Stage
Description
ROC (AUC)
tPSA
Serum
Early
Standard total PSA
0.55 [251]
%fPSA
Serum
Early
>25% fPSA less likely to have positive biopsy
0.65 [251]
p2PSA
Serum
Early
Splice variant of PSA
0.55 [251]
PHI
Serum
Early
Calculated Index
0.70 [251]
PCA3
Urine
Early
Non coding mRNA
0.74 [252]
TMPRSS2:ERG
Urine
Early
Fusion gene
0.68 [252]
28.11.5.2 Urine Biomarkers
28.11.5.3 Whole Blood Biomarkers
28.11.5.4 Circulating Tumour DNA
28.11.5.5 Exosomes
28.11.5.6 Tissue Biomarkers
28.11.5.7 Genetic Mutations and Gene Expression Profiling
28.11.5.7.1 Gene Expression Profiling
28.11.5.8 Focal Therapies
28.11.5.8.1 High‐Dose Rate Brachytherapy
28.11.5.8.2 Cryotherapy
28.11.5.8.3 High‐Intensity Focused Ultrasound
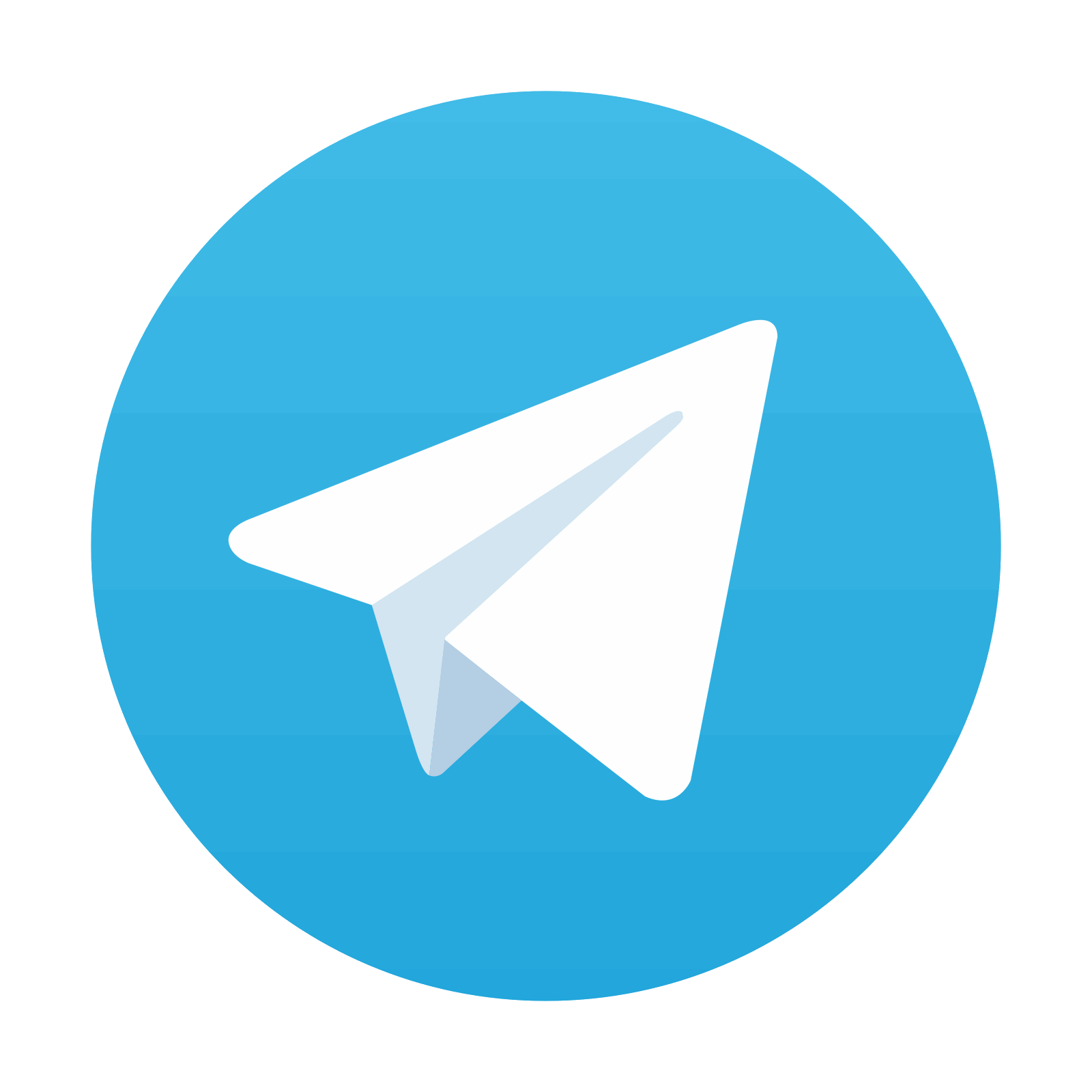
Stay updated, free articles. Join our Telegram channel

Full access? Get Clinical Tree
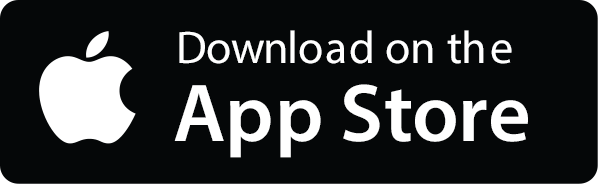
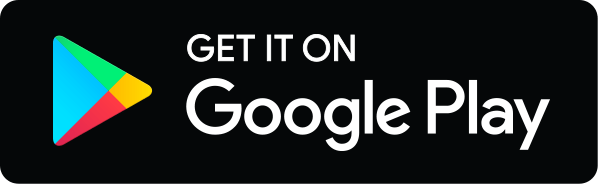