Fig. 7.1
Two types of gene loading to HAC. The gene of interest, isolated in a circular vector, is introduced into the HAC by site-specific insertion (above). A megabase-size gene locus, which is above the capacity of circular cloning vectors, is introduced into the HAC by site-specific reciprocal chromosome translocation (below)
As next generation of construction of HAC vector, a novel HAC vector may be generated using the genome-editing technologies in human primary or iPS cells via top-down approach for safe gene and cell therapy, without using intermediate host cells such as DT40 cells although the technologies for transfer of the HAC from normal human cells to desired patient cells without using A9 or CHO cells need to be developed.
One application of HAC vector is transfer of multiple genes into target cells. Since the HAC vector possesses a single acceptor site (loxP), multiple genes should be unified in a donor vector such as BAC or PAC by conventional in vitro recombinant DNA technique. Processing of BAC or PAC for unifying multiple genes is, however, laborious because of their low copy number in host E. coli and of their large size beyond the fractionation range in gel electrophoresis.
An alternative to unifying multiple genes in a single donor vector is the increase of acceptor site on the HAC vector by utilizing other recombinase or integrase systems capable of site-specific insertion of donor vector. Application of several integrase systems derived from different microorganisms had been reported in mammalian cells [8]. Yamaguchi et al. made a HAC vector carrying 5 acceptor sites for utilizing FLP recombinase and phiC31, R4, TP901-1, and Bxb1 integrases [9], which was designated as MI-HAC (multi-integrase HAC) (Fig. 7.2a). In the MI-HAC, a pair of promoters for a selection marker gene and an acceptor site for an integrase were tandemly placed, while in a donor vector, a gene of interest was placed along with a promoter-less drug-resistant gene. Theoretically, up to five different genes of interest could be loaded onto the MI-HAC by reconstruction of the selection marker gene by site-specific integration of the donor vector. While the MI system enabled effective loading of the gene of interests, it requires multiple selection markers.
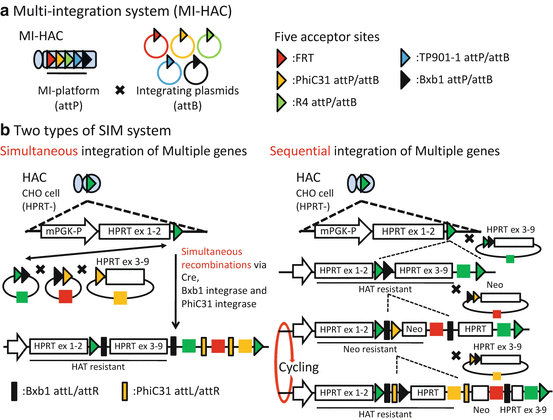
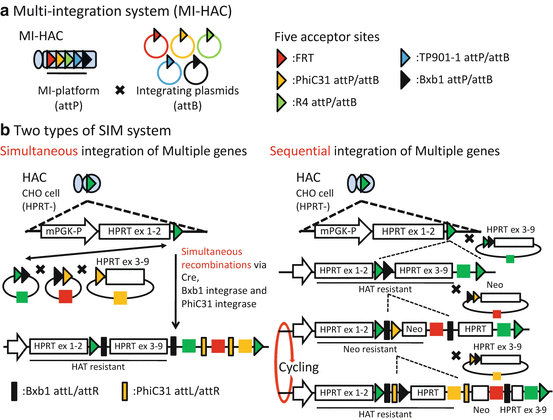
Fig. 7.2
Multiple-gene integration system with multiple-integrase system (MI system) and simultaneous or sequential integration of multiple-gene-loading vector system (SIM system). (a)MI-HAC can be inserted to each donor plasmid in each acceptor site. MI-HAC harbors five acceptor sites, which are called MI-platform and include FRT, phiC31 attP, and R4 attP. On the other hand, each donor plasmid contains a companion sequence like FRT or phiC31 attB. Thus, MI-HAC can be inserted to five plasmids in each acceptor site with site-specific recombinase or integrase, respectively. (b) There are two types of SIM system. A SIM system (left) can insert three plasmids in HAC, simultaneously. These three plasmids are combined via each recombinase and then integrated in HAC, simultaneously. Another SIM system (right) can insert several plasmids, sequentially. A first plasmid is inserted in HAC with loxP site via Cre. It also contains selectable marker gene and another acceptor site like Bxb1 attB. Thus, next donor plasmid can be inserted sequentially. Since the next donor plasmids cut in between a selectable marker gene and a promoter, this sequential integration system can need only two selectable marker genes and is theoretically possible to load unlimited number of genes [19]
To overcome the problem of this complicated gene-loading protocol on the HAC vectors, a simple method for the simultaneous or sequential integration of multiple-gene-loading vectors into a HAC vector, designated as the simultaneous or sequential integration of multiple-gene-loading vector (SIM) system, was reported [3, 10]. In the SIM system, simultaneous integration is attained by stepwise nested insertion of gene-loading vector by different integrases (Fig.7.2b, left). Sequential integration is attained by the shedding of formerly reconstructed marker gene, caused by targeted insertion of an ensuing donor vector (Fig. 7.2b, right). These are achieved by elaborate placement of target sequence for the integration and smart utilization of splicing acceptor and donor cassettes to splice out the acceptor site for the next reaction embedded between the front and rear half of the selection marker genes. A prominent feature of this system is that multiple-gene-loading vectors can be integrated by the cycling use of only two selection marker genes at most. Thus, the SIM systems on HAC vectors are very useful and are expected to expand the applicability of HAC vectors for multiple-gene expression study, because the SIM systems can be applied to any HAC with a 5′HPRT-type cassette.
7.2 Microcell-Mediated Chromosome Transfer (MMCT)
A chromosomal vector including HAC can be transferred into other desired cell lines by microcell-mediated chromosome transfer (MMCT). For MMCT, donor cells (normally mouse A9 cells and CHO cells) are induced to multinucleate their chromosomes (Fig. 7.3). Micronuclei are then forced through the cell membrane to create microcells by centrifugation in the presence of cytochalasin B which disrupts the cytoskeleton [11]. These microcells can be fused to a recipient cell line under appropriate conditions.
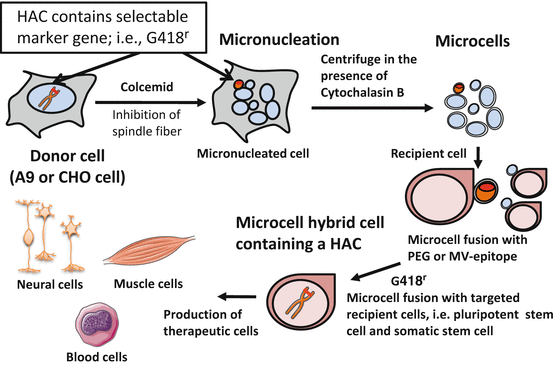
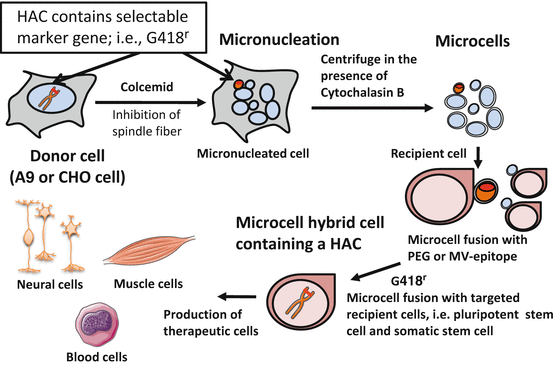
Fig. 7.3
Microcell-mediated chromosome transfer (MMCT) to recipient cells. The figure shows the introduction of the marked human chromosome from the donor A9 or CHO cells to the recipient cells. The procedure can be divided into several parts: micronucleation of the donor hybrids by colcemid treatment, enucleation in the presence of cytochalasin B, purification of the microcells, fusion with the recipient cells, drug selection of the microcell hybrids, identification of the transferred human chromosome by fluorescence in situ hybridization, and DNA analyses. This figure was produced using Servier Medical Art (http://www.servier.com)
MMCT comprises multiple steps: (1) induction of micronuclei in donor cells, (2) isolation of microcells from micronucleated donor cells, (3) fusion of microcells with recipient cells, (4) integration of the transferred chromosome into host cell nucleus, and (5) selection of microcell hybrid cells by drug selection (Fig. 7.3). Eventual efficiency is determined by the summation of contribution from each step. Established cell lines such as pluripotent stem cells including embryonic stem cells, induced pluripotent stem cells, and somatic stem cells have been preferably used as recipient cells. Efficiency of MMCT has been described by the ratio of drug-resistant colony number to recipient cells. Depending on the type of recipient cells, the efficiency is generally 10−5–10−6 when using polyethylene glycol (PEG) for the fusion of microcells and recipient cells. With the advent of stem cell studies, the target of MMCT has been extended to primary cells with finite life span, somatic stem cells, and induced pluripotent stem (iPS) cells. Improvement of the efficiency has emerged as an issue to be resolved.
The microcells conventionally have been fused to recipient cells in the presence of polyethylene glycol (PEG) which acts as a dehydrating agent and fuses plasma membranes. Recently, a more efficient MMCT procedure has been developed [12]. During the course of virus particle formation in the infected cells, viral fusogenic proteins are synthesized de novo, transported to the cell periphery, and presented on the cell surface, followed by extrusion of cell membrane as virus envelope [13]. This well-controlled budding mechanism of envelope virus prompted us to make “a fusogenic microcell” which carries a chromosome to be transmitted and is coated with fusogenic envelope proteins. We chose fusogenic envelope proteins from Measles virus (MV) for which accumulating data have been reported. MV has two envelope glycoproteins, hemagglutinin (H) and fusion (F) proteins, for infection into host cells. Virus particle specifically attaches to the surface of host cells by the interaction between H protein and its receptors on the host cell surface. Binding of the H protein to a receptor triggers the fusion of virus envelope with the host cell membrane by the mediation of F protein. To make “fusogenic microcells,” expression plasmids encoding H and F proteins were transfected into CHO cells carrying a HAC vector. Microcells isolated from the CHO donor cells showed fusion ability to recipient human cells that express a receptor protein CD46, leading to successful transfer of the HAC [12]. It was noted that the MMCT efficiency depended on the expression level of CD46 in recipient cells. CD46 belongs to the family of complement activation regulators that prevent self-cell destruction [14]. Overexpression of CD46 is frequently observed in cancer cells to overcome lysis by complement [15]. Indeed, in the case of fibrosarcoma cell line HT1080 which has high surface density of CD46, the MMCT efficiency was 2 orders of magnitude higher than that with PEG fusion. However, in the case of primary fibroblasts that have low surface density of CD46, the efficiency was comparable to that with PEG fusion. An issue with this method is the narrow range of recipient cells that can be used to obtain high-efficiency fusion. The preceding studies that describe the usage of MV for oncolysis by infection have proposed retargeting of MV by engineering the H protein, i.e., addition of single-chain antibody fragment against surface receptors other than CD46 [16, 17]. The addition of single-chain variable fragment (scFv) against transferrin receptor (TfR) improved fusion efficiency to primary fibroblasts [18]. Although high affinity of scFv to desired surface receptor is not always available with ease, retargeting of MV may be an alternative for PEG-sensitive cells.
7.3 HACs and Potential Medical Applications
Gene therapy has been envisioned to provide a direct and permanent correction of genetic defects. To achieve the desired effects, therapeutic genes need to be carried by safe and effective vectors that can deliver foreign genes to specific cells and thereafter sustain their expression in a physiologically regulated fashion. Gene delivery vectors with the following properties may further add to the applications for autologous gene and cell therapies: (1) high transfer efficiency; (2) long-term stable maintenance in host cells without integration into the host genome; (3) appropriate levels of spatial and temporal expression of therapeutic genes in specifically desired cells; (4) no risk of cellular transformation or stimulation of the host’s immune system; and (5) a system to safeguard against tumor formation. Although a number of different approaches have been attempted to achieve efficient gene transfer and long-term gene expression, this challenging task remains unfulfilled because all current methods have certain limitations, including transient expression, consequent toxicity, undesired immunological response, integration of target genes into the host cell genome, and transcriptional silencing.
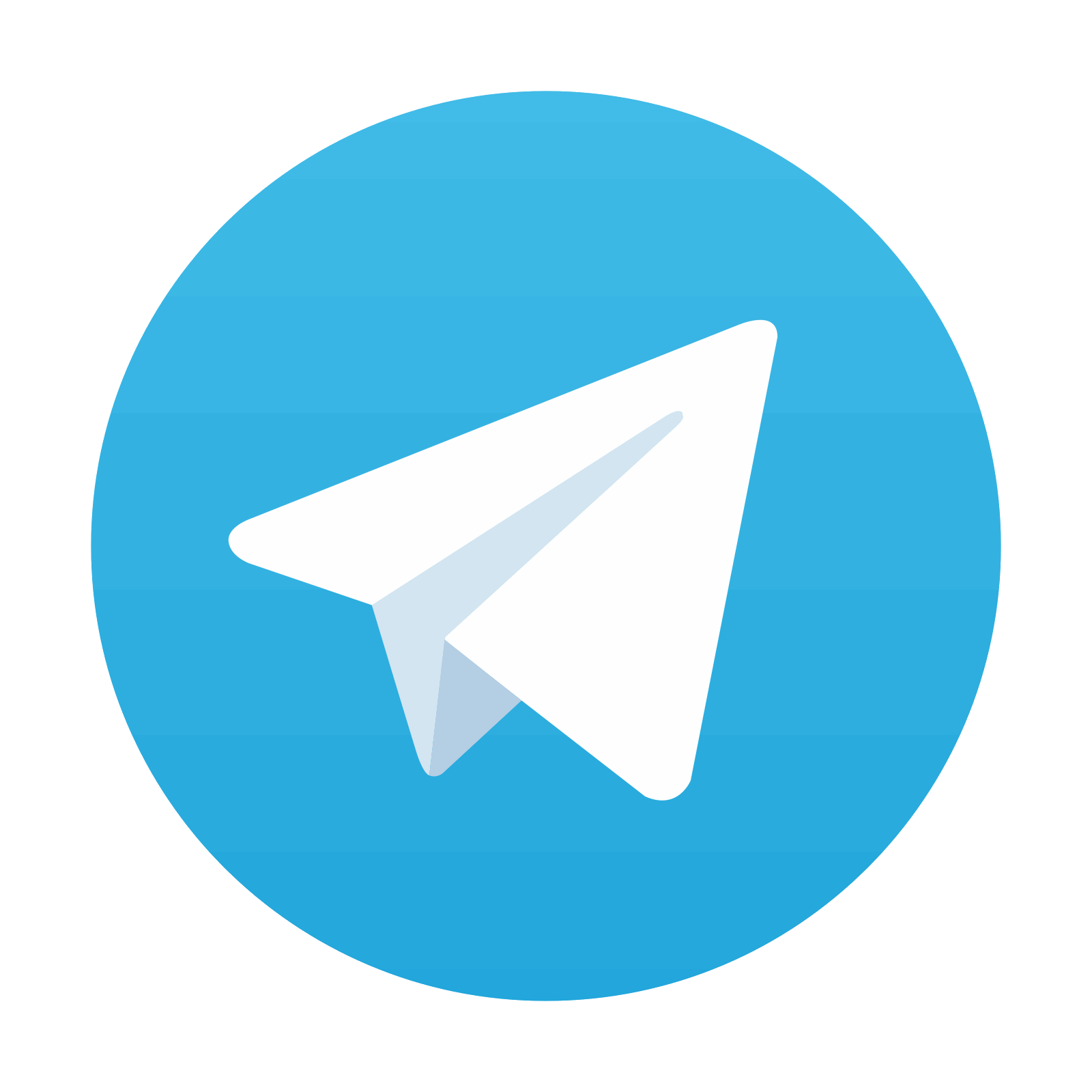
Stay updated, free articles. Join our Telegram channel

Full access? Get Clinical Tree
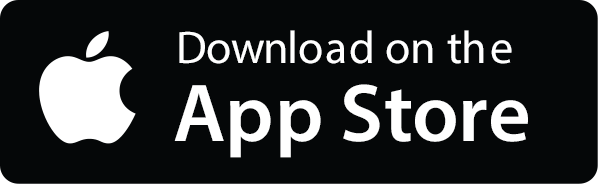
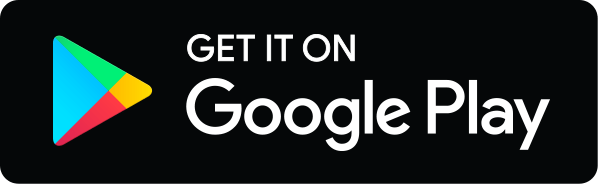