Bioavailability is the percentage or portion of administered drug that reaches systemic circulation.
Intravenous (IV) route: Bioavailability is 100%.
Non-IV route: Bioavailability depends on:
Ease of absorption (i.e., through gut, skin, or mucosal membrane)
First-pass metabolism (i.e., hepatic metabolism prior to release into systemic circulation)
Increased salivary urea in uremia increases salivary pH, which reduces absorption of drugs that are better absorbed in acidic pH.
Nausea/vomiting causes loss of oral drugs.
Gastroparesis/reduced peristalsis slow drug absorption and delay attainment of plasma peak concentration.
The use of antacids and proton-pump inhibitors (PPIs) can increase stomach pH, which reduces absorption of drugs that are better absorbed in acidic pH (e.g., iron, mycophenolate mofetil [MMF; Cellcept], but not mycophenolate sodium [Myfortic]).
Phosphate binders can form nonabsorbable complexes with some drugs (e.g., quinolones).
Bowel wall edema (e.g., nephrosis) reduces gut absorption.
The total amount of drug in the body, expressed as an imaginary volume with a uniform drug plasma concentration:
Drugs that are highly protein bound stay in the vascular compartment and have a limited Vd.
Vd is low for drugs with high water solubility and high plasma protein binding.
Primary drug-binding proteins include albumin and α1-acid glycoproteins:
Organic acids generally bind to albumin.
Organic bases generally bind to glycoproteins. Increased synthesis of α1-acid glycoproteins may occur in inflammatory states and may alter free drug levels.
Reduced serum proteins due to renal loss (e.g., nephrotic syndrome), malnutrition, and/or reduced synthesis
Competitive binding of uremic toxins to plasma proteins displaces protein-bound drugs into plasma, resulting in increased free (unbound) drug concentrations.
Organic acids that accumulate in renal failure compete with acidic drugs for protein binding. Therefore, a larger fraction of acidic drugs will exist in the unbound active state (e.g., salicylates, warfarin, sulfonamides, phenytoin).
Basic drugs bind more readily to nonalbumin proteins.
Alteration in blood pH
Predicting the effect of changes in protein binding on the kinetic parameters of drugs may be difficult. Although higher free drug concentrations are available at the site of action/toxicity, more is also available for metabolism or renal excretion.
Increased Vd: edema, ascites/effusions, adsorption onto apparatus (dialysis filters, extracorporeal membrane oxygenation [ECMO] circuits), volume expansion due to extracorporeal machinery (an extra 2,000 to 2,500 mL)
Reduced Vd: older age, muscle wasting, amputations, loss of body fat, volume depletion
Effect of reduced plasma protein binding on Vd: Predicting the effect of reduced plasma protein binding on the Vd may be difficult. Although more free drug is available for redistribution into other extravascular compartments that can result in increased Vd, more is also available for metabolism or renal excretion. The latter can result in reduced Vd.
Loading dose is directly proportional to Vd:
where bioavailability = 1 for IV drug infusion.
In the absence of a loading dose, maintenance doses alone will not achieve steady-state level until five drug half-lives later. Thus, for some drugs, a loading dose is given to reduce the time to steady state when it is critical to rapidly achieve therapeutic concentrations (e.g., antibiotics in the setting of sepsis or septic shock).
Most drugs are cleared/metabolized by the liver and/or kidneys. Plasma clearance is the sum of clearance of a drug by both renal and nonrenal routes.
There is evidence that renal impairment may also reduce nonrenal (i.e., hepatic) metabolism of drugs, presumably via an increase in a circulating inhibitor of hepatic metabolic pathways (cytochrome P450). This factor is thought to be dialyzable.
Drug clearance determines maintenance dose.
Dose adjustment based on altered drug clearance in a patient with kidney failure:
Example 1: A drug is 100% excreted by the kidneys. Normal maintenance dose is 100 mg. If kidney function is now 30% of normal, what should be the new maintenance dose?
Maintenance dose should be reduced to 30% of normal dose, 30 mg.
Example 2: A drug is 40% cleared by liver and 60% by kidneys. Normal maintenance dose is 100 mg. If kidney function is now 40% of normal and assuming liver clearance is preserved, what should be the new maintenance dose?
Clearance of the drug is still 40% by liver, but now clearance by kidneys is only 40% of 60%, which is 24%. Total drug clearance would now be 40% by liver plus 24% by kidneys, or 64% total clearance. Maintenance dose should be reduced to 64% of normal dose, 64 mg.
A drug t½ is the time needed for the plasma level of the drug to decrease by 50%.
Generally, for an oral drug, 4 to 5 half-life duration are needed to reach steady state. Example: For a drug with t½ of 12 hours, it takes up to 5 × 12 hours = 60 hours (2½ days) to reach steady state. If an immediate therapeutic level is needed, a loading dose must be given.
Half-life determines dosing frequency.
Renal drug clearance = UV/P of drug (and metabolites if applicable), where UV = total amount of drug in urine and P = plasma concentration of drug.
Renal drug clearance is dependent on the drug characteristics (molecular size, charge, protein binding), glomerular filtration rate (GFR), tubular secretion and reabsorption, and renal epithelial cell metabolism.
Dose reduction in patients with chronic kidney disease (CKD) is generally indicated when ≥30% of a drug or its active metabolite is cleared by the kidneys. Similarly, when a drug is cleared by ≥30% with dialysis, the drug is considered “dialyzable.”
Estimation of kidney function for dose adjustment is traditionally done with the Cockcroft-Gault equation. GFR estimation using the Modification of Diet in Renal Disease (MDRD) or Chronic Kidney Disease-Epidemiology Collaboration (CKD-EPI) formulas can lead to dosage recommendations different from those obtained by the Cockcroft-Gault equation, particularly if not corrected for body surface area. Read drug information provided by the pharmaceutical company regarding method of kidney function estimate used for dose adjustment.
Acute and severe kidney injury: Assume <10% kidney function and dose accordingly.
Rapidly improving kidney function: dose drug as if patient had normal kidney function
Decrease dosage: This is the preferred method when maintaining constant drug level is more critical than achieving high peak levels for therapeutic effect (e.g., anticonvulsants, antiarrhythmics). Modified maintenance dose for renal insufficiency may be calculated as the (ratio of patient’s to normal creatinine clearance) × standard maintenance dose. Example: A patient with CrCl 69 mL/min (normal CrCl 120 mL/min) on a standard maintenance drug dose of 100 mg q8h. Modified dose = (69/120) × 100 = 58 or ˜60 mg q8h.
Reduce drug administration frequency: Preferred method if peak level is critical for therapeutic effect (e.g., antimicrobials such as AGs). Modified drug administration frequency for reduced kidney function may be calculated as the (ratio of normal to patient’s CrCl) × current frequency. The modified frequency for the above example would be (120/69) × 8 hours = 14 hours. For simplicity, the drug can be given as 100 mg q12h.
Combination of above: Preferred method for drugs with narrow therapeutic index (e.g., digoxin). For same patient above, the drug can be given as 80 mg q10h.
Many medications have very specific CrCl cutoffs for dosing adjustments. Consult the manufacturers’ drug information for details.
Dialysis drug clearance may occur via both diffusion and convection.
Clearance is dependent on the characteristics of the drug and dialysis.
Drug characteristics: molecular size (<500 Da for hemodialysis [HD], up to 5,000 Da for continuous renal replacement therapy [CRRT]), water solubility, protein binding, Vd, plasma clearance.
Dialysis characteristics: membrane pore size, blood and dialysate flow rates, dialysis frequency and duration, ultrafiltration rate, replacement solution location in CRRT (predialysis vs. postdialysis)
Drug clearance by dialysis is considered clinically significant if drug clearance is increased by ≥30% with dialysis.
Whenever feasible, close therapeutic drug monitoring is recommended.
Drug clearance is dependent on dialysis membrane characteristics, blood and dialysate flow rates, and dialysis frequency and duration.
Clearance = drug extraction ratio × blood flow, where extraction ratio = (predialysis concentration – postdialysis concentration) ÷ predialysis concentration
“High-efficiency,” “high-flux,” and “high-permeability” dialysis membranes can lead to significantly higher drug clearance, particularly for highly water-soluble drug. Example: Vancomycin clearance is significantly increased with high-flux membranes. “High-permeability” membrane is defined as having an ultrafiltration coefficient Kuf > 12 mL/mm Hg/h.
Protein-bound drugs may be better cleared with PD compared with HD due to larger peritoneal membrane pore size.
Drug clearance by PD is typically approximated at 10 mL/min.
Drug clearance by PD may increase with the following:
Peritonitis due to increased blood flow
Increase number of daily exchanges
Literature on drug removal with plasmapheresis is lacking.
Plasmapheresis may significantly remove drugs with high plasma protein binding and/or low Vd.
Intravenous immunoglobulin (IVIG), rituximab, and antithymocyte globulin (used for the treatment of acute antibody-mediated and acute vascular rejection) are removed by plasmapheresis. Although the extent of drug removal by such procedure is unknown, as much as a 50% dose loss during plasmapheresis has been described. These agents should be administered immediately after plasmapheresis if possible.
AGs are mostly excreted unaltered by glomerular filtration and, to a much lesser extent, by tubular secretion. Tubular reabsorption can lead to high renal cortical tissue concentrations even in advanced renal impairment.
AGs can cause nephrotoxicity and ototoxicity due to intracellular accumulation and associated injury to lysosomes, Golgi apparatus, mitochondria, and endoplasmic reticulum in proximal tubular cells and inner ear hair cells, respectively. AG nephrotoxicity is typically evidenced by a rise in serum creatinine within 7 to 10 days of exposure.
Electron microscopy reveals “myeloid bodies” in proximal tubular cells. Myeloid bodies are thought to arise from drug trapping, followed by a gradual accumulation of drug-phospholipid complexes within the internal lysosomal membranes. The increase in undigested materials interferes with normal membrane activity
and results in the accumulation of concentric multilamellar lipid layers known as myeloid bodies, which may have similar appearance as those seen in Fabry disease (see Chapter 7 Glomerular and Vascular Diseases).
Nephrotoxicity risks: older age, underlying CKD, diuretics, concurrent use of nephrotoxic agents or IV radiocontrast agents, hypokalemia, hypovolemia
Bactericidal activity of AG is dependent on the initial rapid intracellular accumulation, followed by significant tissue release postantibiotic administration. The latter is termed “postantibiotic effect.” Drugs with “postantibiotic effect” depend on the loading dose but require less frequent dosing.
In general, daily dosing or even q36h to q48h dosing of AG is thought to minimize nephrotoxicity in patients with GFR less than 60 mL/min.
Dosing for all AGs is based on ideal body weight and adjusted body weight for obese patients. Serum levels should be measured to ensure therapeutic levels and avoidance of toxicity.
In HD, predialysis dosing of AG allows for higher maximal concentration and theoretically better efficacy. Postdialysis dosing of half dose has also been suggested, but potential for higher toxicity must be noted.
AG drug removal is thought to be greatest with CVVHDF (significant solute removal by both diffusion and convection), followed by continuous venovenous hemofiltration (CVVH) (significant solute removal by convection) and intermittent HD (predominantly diffusion).
In peritonitis, AG should be given intraperitoneally.
Both are predominantly excreted by kidneys.
When given IV, both agents are nephrotoxic and ototoxic, but teicoplanin is less nephrotoxic.
Nephrotoxicity is thought to involve oxidative stress. Use of vitamin E and N-acetyl cysteine has been suggested to ameliorate vancomycin-induced nephrotoxicity.
Nephrotoxic risks include underlying kidney injury, concurrent use of nephrotoxic drugs, prolonged therapy, and high plasma levels.
Dialysis vancomycin dosing of 1 g can maintain plasma level above minimum inhibitory concentration (>15 µg/mL) for 3 to 5 days. Of note, trough levels >15 µg/mL are associated with greater nephrotoxicity. Random level monitoring may be used to determine the timing of subsequent doses among patients with renal replacement therapy or advanced kidney disease not on scheduled dosing.
Most (penicillins, cephalosporins, carbapenems, monobactams) require dose reduction in CKD. β-Lactams are commonly combined with β-lactamase inhibitors (e.g., clavulanate, sulbactam, tazobactam, avibactam) to minimize antibiotic resistance and improve activity spectrum. Dose adjustment for combination drugs must take into account different metabolism rates of both agents. Whereas rates of metabolism of both agents in ampicillin/sulbactam and piperacillin/tazobactam
are similar, clavulanate metabolism is much faster than that for ticarcillin in the ticarcillin/clavulanate combination.
Unlike AG, most β-lactams have short half-life and no postantibiotic effect. Dose reduction is generally preferred over frequency reduction.
Sulfonamides are typically combined with trimethoprim.
Both are renally excreted. However, alkaline urine enhances the excretion of sulfamethoxazole, whereas acidic urine promotes trimethoprim excretion.
Both accumulate in patients with reduced GFR and dosage adjustment is recommended.
Sulfonamides are excreted following acetylation. Acetylated sulfonamides may crystallize in tubular lumen and cause kidney injury, particularly with cumulative dose >84 g. Good hydration and alkalinization may be both preventive and therapeutic.
Sulfonamides may also cause tubulointerstitial nephritis.
Trimethoprim may inhibit tubular secretion of creatinine and may cause a rise in serum creatinine without actually causing kidney injury.
Trimethoprim may block the epithelial sodium channel (ENaC) and cause both hyperkalemia and metabolic acidosis.
Rifamycins are predominantly hepatically metabolized and do not require renal dose adjustments..
Rifamycins may cause orange-reddish discoloration of body fluids, such as urine, effusions, and PD fluids.
Rifampin may cause various renal lesions, including acute tubular necrosis (ATN), acute tubulointerstitial nephritis (ATIN), light-chain proteinuria, and even rapidly progressive glomerulonephritis (RPGN). Intermittent use (e.g., noncompliant patient) is associated with ATN, with or without interstitial infiltrations, hemolysis, and thrombocytopenia. Continuous use is associated with light-chain proteinuria and RPGN.
Rifampin is a potent CYP3A4 inducer and can cause subtherapeutic concentrations and treatment failure of various drugs, including calcineurin inhibitors (CNIs) (cyclosporine [CsA], tacrolimus [Tac]), mammalian target of rapamycin (mTOR) inhibitors (sirolimus, everolimus), corticosteroids, and statins. Significant dose increase of affected drugs is generally required.
Ciprofloxacin, norfloxacin, and gatifloxacin are significantly cleared by the kidneys and must be dose reduced for GFR below 30 mL/min.
Moxifloxacin is only 20% renally excreted and does not require dose adjustment.
Quinolones may cause nonspecific CNS symptoms, including headaches, dizziness, restlessness, tremors, and kidney injury from interstitial nephritis, crystalluria.
Quinolones absorption is reduced with metal-containing compounds and phosphate binders.
There are concerns for toxic accumulation in patients with advanced CKD and potential for pulmonary and hepatic toxicity and peripheral neuropathy. The Food and Administration (FDA)-approved labeling states that the use of nitrofurantoin is contraindicated in patients with a CrCl less than 60 mL/min. Nonetheless, data are lacking to support this recommendation. Retrospective studies have shown that short-term nitrofurantoin use is effective and generally well tolerated in patients with a CrCl of 30 mL/min or more, although higher adverse events have been noted in patients with renal impairment. However, the use of nitrofurantoin in the treatment of urinary tract infection in patients with low GFR is not recommended because the drug cannot accumulate to reach bactericidal concentrations in the urine, thus increasing the chance of treatment failure.
Doxycycline, tigecycline, and standard-dose minocycline: No dose adjustment needed. For CrCl <80 mL/min, minocycline maximum dose is 200 mg/d.
Linezolid: No dose adjustment needed.
Linezolid and tetracyclines can lead to type B lactic acidosis. See Chapter 2.
Metronidazole: Only 15% of the parent drug is renally cleared. Reduce to twice-daily dosing in dialysis patients.
Macrolides:
Azithromycin, clarithromycin, roxithromycin (hepatically cleared): No dose adjustment needed.
Erythromycin (renally cleared): Dose reduction is required.
Kidney failure may occur after 2 weeks of therapy and is associated with the cumulative dose received. Lipid formulations can allow additional doses to be given by delaying the onset of nephrotoxicity.
Risks: older age, underlying CKD, hypovolemia, hypokalemia
Amphotericin may induce distal tubular injury, distal RTA, magnesium and potassium loss, nephrogenic diabetes insipidus, and arteriolar vasoconstriction (afferent greater than efferent arterioles).
Other noted adverse effects: anemia with or without thrombocytopenia presumably due to high inorganic content in liposomal formulations and, possibly, measurement interference/error
Liposomal or lipid-based formulations confer lower electrolyte disturbances and are preferred in patients with any degree of residual kidney function. Acute allergic reaction may be seen with lipid-based formulations. Pseudohyperphosphatemia may be seen with liposomal amphotericin (see Chapter 3).
Amphotericin is highly protein bound (˜90% to 95%) with a large Vd and is thus not well dialyzable. The large Vd is thought to be due to uptake by tissues.
Management of IV amphotericin B-induced nephrotoxicity:
Routine preventive measures: normal saline, use of lipid formulations of amphotericin B, reduce administration frequency if possible
Continuous infusion may be less nephrotoxic compared with infusions given over 4 hours.
Theoretical benefits of low-dose calcium channel blockers (e.g., diltiazem) to reduce renal vasoconstriction may be considered if safely tolerated.
With the exception of fluconazole, most agents (keto-, itra-, vori-, posa-, and isavuconazole) are metabolized by the liver and do not require dose reduction.
Fluconazole is significantly excreted in the urine and is preferred for the treatment of UTIs. After an adequate loading dose, maintenance dose should be reduced in in patients with reduced GFR.
Most azoles are potent inhibitors of CYP3A4 and P-glycoproteins that are involved in the metabolism and absorption of various drugs, including CNIs (CsA, Tac), mTOR inhibitors (sirolimus, everolimus), and statins. Significant dose reduction of the affected drug is generally required.
Itraconazoles, voriconazole, and posaconazole are mixed with cyclodextrin in IV formulations. Accumulation of cyclodextrin in renal patients can lead to increased serum creatinine and CNS toxicity (e.g., agitation, myoclonus, visual and auditory hallucinations, colored or flashing light). IV formulations should be avoided for GFR <50 mL/min).
Flucytosine is predominantly excreted in the urine and requires dose adjustment for patients with CKD. Flucytosine has a high rate of fungal resistance and is typically not used as a sole agent, but in combination with amphotericin for severe fungal infections.
Echinocandins are inhibitors of the synthesis of β-D-glucan, a fungal cell wall component. These agents are effective against Candida species and azole-resistant Aspergillus.
No dose adjustment is necessary in patients with kidney failure.
Unlike azoles, echinocandins typically do not affect CNI levels. However, caspofungin has been shown to reduce Tac levels.
Terbinafine, an agent used to treat onychomycosis, is metabolized by CYP3A4, and 70% is renally excreted. Plasma clearance is reduced by 50% in patients with CrCl <50 mL/min. There are no safety data for terbinafine in CKD: 50% dose reduction is suggested in moderate-to-severe renal impairment.
Topical azoles, including clotrimazole, econazole, ketoconazole, miconazole, bifonazole, and tinidazole, are minimally absorbed and have no drug interaction.
Topical and oral nystatin is minimally absorbed. Their use in patients with renal impairment is safe.
Griseofulvin: No dose adjustment needed.
Telbivudine, adefovir, tenofovir, and entecavir are extensively renally excreted. Dose adjustment for CrCl < 50 mL/min is recommended.
Entecavir and telbivudine are dialyzable and should be administered postdialysis.
Entecavir does not induce drug resistance unless patient has had prior therapy with lamivudine (3TC) or related drugs.
Tenofovir does not induce drug resistance, irrespective of prior treatment. It can cause Fanconi syndrome and proximal tubular cell dysfunction and AKI.
Tenofovir alafenamide (Vemlidy©) is a novel prodrug of tenofovir with significantly reduced renal and bone effects (i.e., decline in bone mineral density) compared with tenofovir disoproxil fumarate (Viread©). FDA approved for use in patients with mild-to-moderate renal impairment (CrCl ≥ 30 mL/min); dialyzable, should be administered postdialysis.
Ribavarin and its metabolites are excreted by the kidneys. Its use should be avoided in patients with CrCl <50 mL/min. Ribavarin is associated with hemolytic anemia, thrombotic thrombocytopenic purpura, and red cell aplasia.
Pegylated interferons (INFs) α-2a and α-2b require dose adjustment for CrCl <50 mL/min. INFs can upregulate cell surface expression of human leukocyte antigen (HLA) class II, leading to acute allograft rejection. However, with the advent of direct-acting antiviral (DAA) agents, INF-based therapy has become obsolete.
DAA agents:
The use of elbasvir/grazoprevir, sofosbuvir/velpatasvir, or glecaprevir/pibrentasvir combination therapy has been suggested to be safe in patients with severe reduction in GFR. Safety data for other DAA agents in patients with advanced CKD are currently limited. Sofosbuvir can accumulate in severe reduction in GFR, but no dose reductions are provided for by the manufacturer.
Anti-influenza agents (neuraminidase inhibitors):
Oseltamivir (Tamiflu) and peramivir (Rapivab) are excreted by the kidneys and require dose reduction for reduced GFR. Oseltamivir should be given at half dose (30 mg) with CrCl <60 mL/min, and at half dose once daily with CrCl <30 mL/min.
Zanamivir (Relenza) is extensively renally excreted after IV doses. However, its bioavailability from inhaled doses is only ˜2%, which does not require dose reduction.
Renally excreted and may crystallize in tubules, leading to obstructive uropathy and acute kidney injury (AKI), especially when given in high dose via rapid infusion. Slow IV administration (1 to 2 hours) and hydration is critical if given in high doses IV.
Associated with leukopenia, neurotoxicity
Dose reduction based on kidney function is necessary.
Approximately 60% dialyzable.
Renally excreted, accumulation may lead to severe bone marrow toxicity.
Dose reduction based on GFR is necessary.
Highly dialyzable
Integrase inhibitors (raltegravir, elvitegravir, dolutegravir)
Fusion inhibitors (enfuvirtide)—Enfuvirtide may be associated with membranoproliferative glomerulonephropathy (MPGN).
CCR5 antagonists (maraviroc)
Dose adjustment not required for mild-to-moderate CKD.
For CrCl < 30 mL/min or dialysis dependent: Avoid
Protease inhibitors (atazanavir, darunavir, indinavir, nelfinavir, ritonavir, saquinavir, and tipranavir)
Non-nucleoside (or nucleotide) reverse transcriptase inhibitors (NRTIs) (delavirdine, efavirenz, etravirine, nevirapine)
Table 10.1 HIV drugs and the kidney | |||||||||||||||||||||||||||||||||||||||||||
---|---|---|---|---|---|---|---|---|---|---|---|---|---|---|---|---|---|---|---|---|---|---|---|---|---|---|---|---|---|---|---|---|---|---|---|---|---|---|---|---|---|---|---|
|
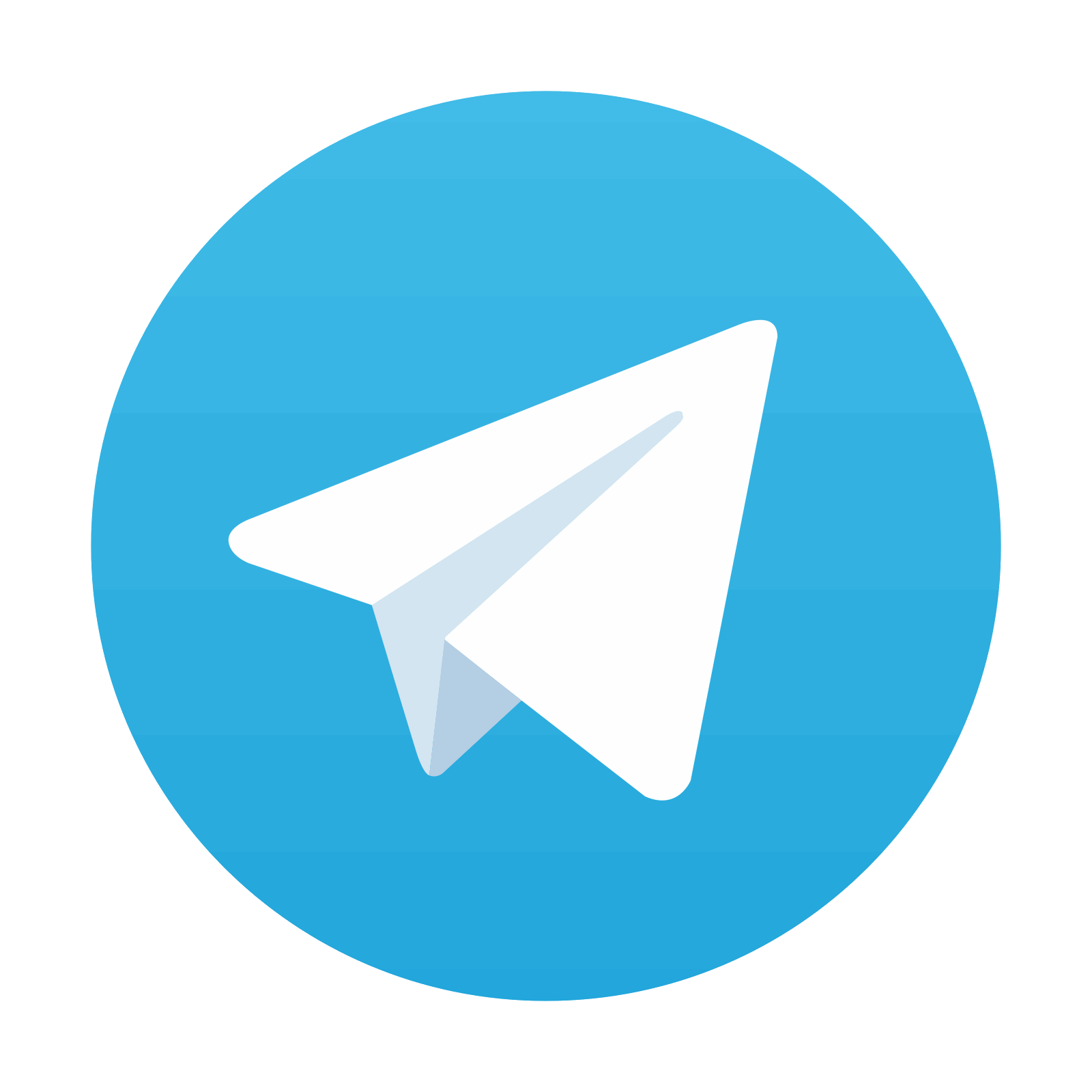
Stay updated, free articles. Join our Telegram channel

Full access? Get Clinical Tree
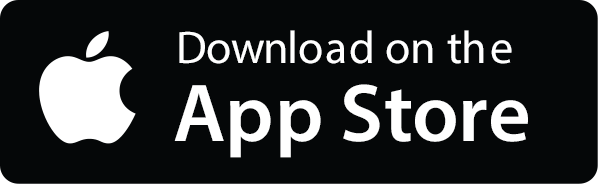
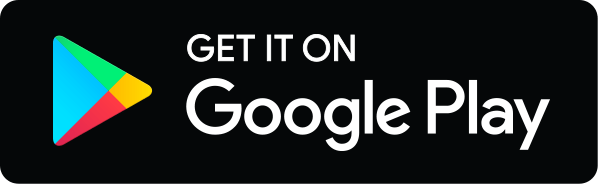
