Perioperative care of the pediatric cardiac surgical patient requires a multidisciplinary team that includes cardiologists, cardiothoracic surgeons, anesthesiologists, and intensivists as well as multiple other support staff. A well-functioning system requires coordinated effort and communication. This chapter focuses on common perioperative critical care challenges encountered in the care of pediatric cardiac surgical patients, with emphasis on physiologic basis, diagnosis, and treatment.
Management of children with congenital heart disease (CHD) requires an understanding of neonatal/pediatric cardiopulmonary physiology and how this differs from the adult. First, neonatal and pediatric cardiac output (CO) is significantly more reliant on heart rate (vs stroke volume) than in the adult patient. As such, these patients will maintain a “normal” blood pressure despite impending shock by increasing heart rate to maintain CO. The neonatal and pediatric myocardium is less compliant (compared to an older child or adult) and is less tolerant of changes in preload and afterload. Oxygen consumption is also increased compared with adult patients, and lung volumes are obviously smaller. In order to maintain oxygen supply in the face of increased consumption with smaller lung volumes, minute ventilation is increased with higher respiratory rates. In addition, infants breathe below their functional residual capacity (FRC), and lung units are collapsed and reopened with each breath. This unique physiology results in low tolerance to apnea and very rapid desaturation.
Neonates also have potential for labile pulmonary vascular resistance (PVR) as they transition from fetal to newborn circulation in the first few days of life. Furthermore, neonatal pulmonary physiology differs from that of an older child because of the increased chest wall compliance, reliance on the diaphragm for optimal mechanics, and increased propensity for atelectasis. The preoperative management of neonates with significant unrepaired CHD requires an understanding of both general neonatal cardiopulmonary physiology as well as the specific impact of the particular lesion(s).
Although the majority of preoperative care of the pediatric patient with CHD is in the outpatient setting, the neonate with significant unrepaired CHD may require intensive care assessment and monitoring. Goals of preoperative care include optimization of hemodynamics to ensure adequate CO and normal end-organ function while awaiting corrective or palliative neonatal surgery.
In general, infants requiring neonatal surgery can usually be classified into three preoperative “states”: single ventricle (SV) physiology, transposition physiology, and ductal-dependent pulmonary or systemic blood flow.
Many complex congenital heart lesions have SV physiology, where there is complete mixing of systemic and pulmonary venous return and thus aortic (AO) saturation equals pulmonary artery (PA) saturation. The total ventricular output is divided between two competing parallel circuits whose flow (pulmonary/systemic) is determined by the resistances of each system. Hypoplastic left heart syndrome (HLHS) typifies SV physiology. Acceptable balance between pulmonary and systemic outputs will provide enough pulmonary blood flow for adequate oxygen delivery without excessive volume load on the SV as well as adequate systemic output and end-organ function. However, since the two circuits are in parallel, too much blood flow to one system occurs at the expense of the other. The neonate with HLHS requires an unrestrictive atrial-level communication to unload the left atrium and ductal patency [via prostaglandin (PGE1) infusion] to maintain systemic outflow. Too much pulmonary blood flow, manifested as “high saturations,” will occur at the expense of systemic output; these infants are at risk for renal insufficiency (due to decreased renal perfusion) and necrotizing enterocolitis (due to decreased splanchnic perfusion).
Transposition of the great arteries (TGA), where the aorta arises from the right ventricle (RV) and the PA arises from the left ventricle (LV) (PA saturation is greater than the AO saturation) mandates mixing. This intercirculatory mixing can occur at any of the following levels: intracardiac (via a patent foramen ovale, atrial septal defect or ventricular septal defect) and/or extracardiac (via patent ductus arteriosus). Effective pulmonary blood flow (net volume of blood flow passing from systemic circulation to pulmonary circulation, the anatomic right-to-left shunt) and effective systemic blood flow (net volume of blood flow passing from pulmonary circulation to systemic circulation, the anatomic left-to-right shunt) is the intercirculatory mixing upon which survival depends. Extracardiac intercirculatory mixing is maintained by ductal patency with a PGE1 infusion, while inadequate intracardiac mixing is palliated with a balloon atrial septostomy (BAS) when needed.
Finally, lesions where either pulmonary or systemic blood flow depends on ductal patency require a PGE1 infusion until palliative or corrective neonatal surgery takes place. Ductal-dependent pulmonary blood flow lesions include tetralogy of Fallot with severe right ventricular outflow obstruction, tetralogy of Fallot with pulmonary atresia, and critical pulmonary stenosis. Critical coarctation of the aorta is another example of a lesion associated with ductal-dependent systemic blood flow.
Indications for preoperative mechanical ventilation in the neonate with unrepaired CHD are commonly due to pulmonary and/or cardiac issues. Causes of respiratory failure are many and may include prematurity with respiratory distress syndrome, meconium aspiration, or infectious etiologies. Cardiac indications are less common, but may include severe hypoxemia due to inadequate intracardiac mixing (neonate with TGA in need of a BAS) or low CO due to increased pulmonary blood flow at the expense of systemic output in the SV patient.
In addition to optimizing pulmonary and cardiac systems, preoperative care of the neonate with CHD should include evaluation of other associated anomalies, including a genetic evaluation as well as head, abdominal, and renal ultrasound where indicated. Blood analysis for genetic abnormalities (including fluorescence in situ hybridization – FISH test) is best performed prior to blood transfusion associated with cardiopulmonary bypass (CPB).
All children undergoing surgical repair for CHD are admitted to an intensive care unit (ICU) postoperatively. Upon admission, the patient will be quickly assessed by an intensivist for hemodynamic stability including heart rate, heart rhythm, clinical CO, perfusion, adequacy of sedation and/or pain control, and respiratory dynamics. Blood work is sent on admission to assess ventilation and oxygenation, electrolytes, hematocrit, platelet count, and coagulation. A chest x-ray is taken to ensure adequate lung inflation, normal heart size and to confirm proper placement of all lines, tubes, and wires.
Verbal report is given by cardiac surgeons and anesthesiologists to the ICU team upon transfer of care. Report should include the patient’s preoperative cardiac anatomy; other significant medical problems; preoperative medications; intraoperative findings and procedure done, including duration of CPB, cross-clamp and deep hypothermic circulatory arrest (DHCA); location of arterial and venous lines; medications required intraoperatively; complications; occurrence of arrhythmias; transesophageal echocardiographic findings; plan, including vital sign goals and anticoagulation plans when applicable (see Table 60-1, sign out components). Patient handover systems and checklists have been studied during transfer of care of cardiac patients and are encouraged to enhance quality of communication and patient safety.1
Patient name | ||
---|---|---|
Age | ||
Weight | ||
Allergies | ||
Surgical procedure | ||
Preoperative data | Intraoperative Data | Postoperative Data and Plans |
|
|
|
|
|
|
|
|
|
|
|
Intensive care of neonatal and pediatric patients relies on both indirect assessments of CO and oxygen delivery as well as direct hemodynamic monitoring. Continuous monitoring of heart rate, respiratory rate, blood pressure, and pulse oxymetry is standard. Depending on patient age and anatomy, peripheral (radial, ulnar) or central (umbilical artery or femoral artery) arterial blood pressure monitoring is performed. Central lines are usually placed in the internal jugular, subclavian, or femoral vein after induction of anesthesia, while direct atrial lines may be placed intraoperatively. In neonates, umbilical venous lines are often placed postnatally. Central lines are used for administration of vasoactive infusions, fluids, parenteral nutrition and for measurement of central venous pressures. If the central line tip is positioned at the superior cavo–atrial junction, it can be used to sample mixed venous saturation as well.
Hypotension in the postoperative period is common and multifactorial. The more common causes are listed in Table 60-2, along with changes in hemodynamics that are unique to each etiology.
Low cardiac output syndrome (LCOS) is one of the most important predictors of poor outcome after surgery for CHD.2 LCOS is a fall in CO with a commensurate increase in systemic vascular resistance (SVR) and PVR, typically occurring between 6 and 18 h after surgery.3 Clinical signs of LCOS include tachycardia, oliguria, delayed capillary refill, metabolic acidosis, hypotension, and low mixed venous oxygen saturation. The mainstay of treatment of LCOS is anticipation and early identification. With predictable decrease in CO and increased PVR and SVR, afterload reduction (milrinone) and inotropic support oppose this trend.4
Inotropic support is a mainstay of postoperative care. Table 60-3 lists the most common vasoactive medications used for the postoperative pediatric cardiac surgical patient.
Drug | Mechanism | Inotropy | Chronotropy | SVR | PVR | Dose | Side Effects |
---|---|---|---|---|---|---|---|
Amiodarone | Class III antiarrhythmic, but with class IB, II and IV effects | ↔ ↓ | ↔ | ↔ | ↔ |
| Slows repolarization and AV conduction, prolongs QTc |
Epinephrine | α1– and β1,2-agonist | + | + | ↑ |
| Hypertension, tachycardia, arrhythmias, myocardial necrosis | |
Dopamine | Dopaminergic1-, α1– and β1-agonist | ↔ | ↔ | ↑ | ↑ |
| Arrhythmias |
Dobutamine | β1-agonist | + | ↔ | ↔ | ↔ |
| Arrhythmias |
Isoproterenol | β1,2-agonist | + | + | ↑ | ↓ |
| Arrhythmias |
Milrinone | Phosphodiesterase- 3 inhibitor | + | ↔ | ↓ | ↓ |
| Hypotension |
Norepinephrine | α1– >>> β1-agonist | + | + | ↑ | ↑ |
| Arrhythmias, hypertension |
Sildenafil | Phosphodiesterase- 5 inhibitor | ↔ | ↔ | ↔ | ↓ |
| Hypotension |
Vasopression | V 1a agonist | ↔ | ↔ | ↑ | ↔ |
| Bradycardia, hyponatremia |
Arrhythmias are a common complication after surgical repair of CHD, with a reported incidence of 15 to 30 percent.5–7 Postoperative arrhythmia prolongs ICU stay and increases morbidity and mortality. Various studies have looked at risk factors for arrhythmias and found age, underlying anatomy and type of repair, duration of CPB, and cross-clamp time to be possible risk factors.5–7 The most common postoperative arrhythmias are junctional ectopic tachycardia (JET), complete heart block, ventricular tachycardia (VT), and supraventricular tachycardia.
The first step in managing a patient thought to have an arrhythmia is to obtain a 12-lead electrocardiogram (EKG). This should be reviewed by a pediatric intensivist or cardiologist to determine the precise type of rhythm present. If the rhythm is complex, a pediatric electrophysiologist may be consulted. If the patient is hemodynamically unstable as evidenced by poor perfusion on exam, low blood pressure, change in mental status, and metabolic acidosis, prompt diagnosis should be made so that correct treatment may be immediately initiated.
JET is one of the most prevalent postoperative arrhythmias. It is an automatic tachycardia that should be suspected when a patient has a gradual increase to heart rates out of range for age, usually greater than 170 beats/min, over minutes to hours. It typically occurs in the first 24 postoperative hours, but may have a later onset. The exact cause is unknown but it is thought to be due to direct or indirect atrioventricular (AV) node injury, including shear forces and edema. Surgery near the AV node is not required as evidenced by the fact that JET may occur after repair of total anomalous pulmonary venous return, Blalock-Taussig shunts, and Glenn and Fontan procedures.
The EKG shows a narrow complex tachycardia with AV dissociation as evidenced by a ventricular rate faster than atrial rate. P waves may or may not be visualized on the EKG depending upon the speed of ventricular rate. If a central venous line is present, cannon A waves are noted on waveform analysis, representing atrial contraction against a closed tricuspid valve. Differential diagnosis of this narrow complex tachycardia includes sinus tachycardia and supraventricular tachycardia. If the diagnosis is unclear and temporary pacing wires are present, an atrial EKG often aids in the diagnosis. The key pathophysiologic finding in JET is loss of AV synchrony. Postoperative patients have decreased CO secondary to cardioplegia, inflammatory response to CPB, and ischemia-reperfusion injury. Loss of atrial synchrony will further decrease CO and may lead to hypotension. Treatment focuses on decreasing catecholamine infusion, as JET is catecholamine-sensitive. Sympathomimetic drugs, such as epinephrine and dopamine, should be quickly discontinued as they may sustain arrhythmia and cause further hypotension. Patients should be sedated and hyperthermia should be aggressively treated. Active cooling may be necessary in refractory and symptomatic cases. Medical therapy includes amiodarone or procainamide to decrease conduction and control ventricular rate. Both drugs should be administered carefully as hypotension may occur. Atrial pacing may also be used to restore AV synchrony. JET is usually self-limited, resolving within the first postoperative week.
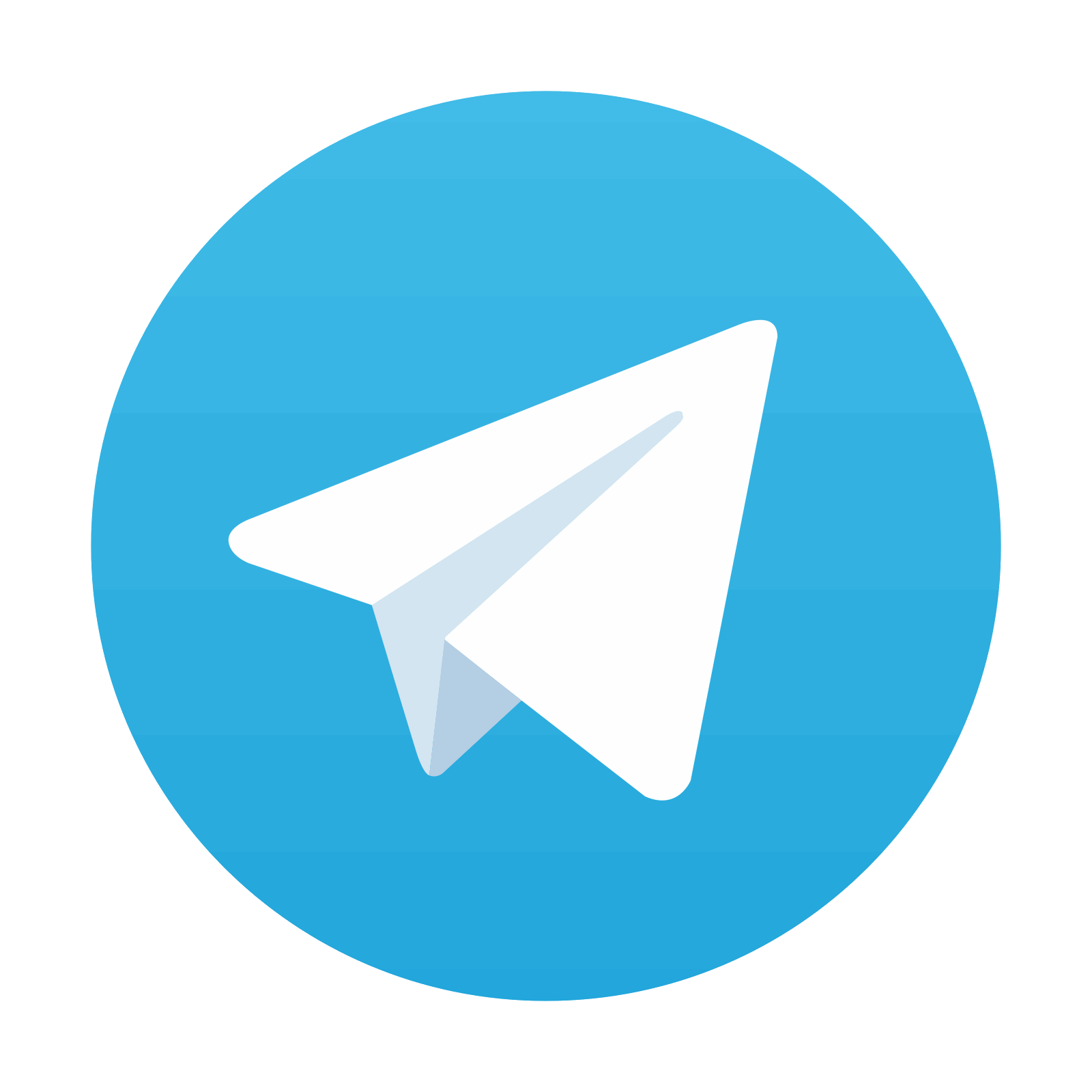
Stay updated, free articles. Join our Telegram channel

Full access? Get Clinical Tree
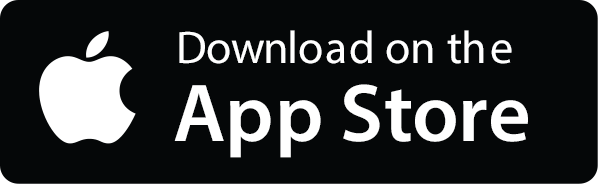
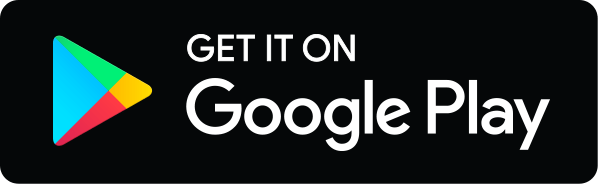