13
Percutaneous Nephrolithotomy Access Under Fluoroscopic Control
Norberto O. Bernardo1 & Maximiliano Lopez Silva2
1 Hospital de Clínicas José de San Martín, Universidad de Buenos Aires, Buenos Aires, Argentina
2 Clínica San Camilo, Buenos Aires, Argentina
The last years of the 1970s and the early 1980s will probably be remembered by urologists as a time of tremendous changes, in particular the whole concept of minimally invasive surgery and the development of percutaneous surgery, which have shown spectacular clinical results and reduced the morbidity of open surgery [1]. Percutaneous stone extraction was first described more than 40 years ago and has become an increasingly common intervention for patients with stone disease [2, 3], evolving into a safe and effective treatment for patients with large or otherwise complex stone disease. However, despite the increasing use of percutaneous renal surgery, Lee et al. reported that only a minority of urologists (27% of those who have trained in percutaneous access) actually gain their own access for percutaneous nephrolithotomy (PCNL) [4]. One of the more common reasons given by respondents for not doing so was inadequate skills in the techniques of access.
The placement of percutaneous access into the intrarenal collecting system is one of the most critical aspects of percutaneous renal surgery. Image guidance is a critical factor for the performance of percutaneous minimally invasive procedures, which are being used with ever increasing frequency. Procedures such as PCNL are not performed without image guidance. The puncture of the kidney, insertion of guidewires, establishment of the percutaneous tract, and the disintegration and removal of stones are based on appropriate image guidance. For percutaneous renal surgery using fluoroscopy, access must be gained: different forms of access have been developed, all with the indispensable assistance of the image intensifier [5].
The first percutaneous nephrostomy to decompress an obstructed kidney was described by William Goodwin in 1955 [6]. However, removal of a renal calculus via a percutaneous tract established specifically for that purpose was not performed until 1976, when Fernstrom and Johansson used the technique successfully in three patients [7]. In their series, the tract was slowly dilated under fluoroscopic control over a 7‐day period to a size sufficient for stone extraction. Similarly, Alken et al. in their experience with PCNL established access to the renal collecting system over the course of weeks, by repeatedly dilating it with hand‐shaped polyethylene tubes of increasing size [8]. He subsequently developed metal telescope dilators to solve the problems met during the treatment of his first 21 patients, which accelerate the procedure and allow percutaneous stone removal to be performed in a single session with a delicate set of instruments. In 1982, Smith et al. described the rapid dilation of the nephrostomy tract in minutes with no untoward effects, which revolutionized the field of percutaneous stone surgery and contributed to the demise of open surgery [9]. Since that time the percutaneous approach has generated wide interest among the pioneers of endourology, and they developed and popularized most of the basic principles in this area in the late 1970s.
Fluoroscopy
Imaging equipment in percutaneous renal surgery typically uses radiation for image formation and guidance during access and tract dilation.
Fluoroscopy is useful during the advancement of guidewires, tract dilation, stone removal, and nephrostomy placement, providing real‐time depiction of the collecting system and the stones therein. Percutaneous renal surgery is performed with a combination of fluoroscopic and endoscopic visualization of the collecting system. Fluoroscopy is a two‐dimensional method and provides limited information regarding the surrounding soft tissue. Nevertheless, it has proven to be an invaluable tool for the performance of percutaneous procedures of the kidney and collecting system.
Radiation exposure
All parameters of fluoroscopy affecting image quality, reproducibility, and radiation output from each radiographic unit must be evaluated routinely to ensure optimal image quality while minimizing the radiation dose [10]. The endourologist can improve their imaging techniques and minimize both their and the patient’s radiation exposure with no concurrent loss of image quality.
When attempting to obtain a diagnostic‐quality image and the image is underpenetrated, and given the choice between increasing the total number of X‐rays (mA) or the penetrability of the X‐rays already present (kVp) to improve the image, the kVp should be increased initially as this will not increase the radiation output [11]. Collimating the image to the minimum size necessary for performing the work will reduce the amount of unnecessary radiation to which the patient and anyone else in the room is exposed. The radiation output from the X‐ray tube should have been evaluated within the past year by a radiologic physicist, who determines whether the unit’s radiation output is within legal limits as well as optimal for each examination.
There have been various reports evaluating the typical radiation exposure from fluoroscopy during PCNL, with an estimated effective dose between 7.63 and 8.66 mSv. Certain risk factors increase radiation exposure during PCNL. These include high body mass index, increased stone burden, and increased number of access tracts [12]. In a prospective study of PCNL and obesity, total operative time and radiation exposure increased as patients’ body mass index increased, in part due to technical challenges. Obesity increased fluoroscopy time by 36% and mean effective dose by 177% [13].
Radiation “spreads out” in a three‐dimensional (3D) space with a discrete or fixed number of photons spreading out into successively larger spaces. As a result, the area increases geometrically as a function of distance from the source. Increasing the distance from the source is one of the least expensive and most dramatic ways to reduce the dose of radiation to which operating personnel are exposed. By doubling the distance from the source, the radiation is reduced to one‐quarter of its original intensity because the same number of photons is in a space that is four times larger. Similarly, by tripling the distance, radiation is reduced to one‐ninth. Moving 1 m further away from an initial distance will reduce the dose by 89%.
The principle of radiation exposure to be as low as reasonably achievable (ALARA) should be followed during procedures that require fluoroscopy. A drape placed over or under the patient helps reduce scatter radiation [12]. Shielding, whether provided by lead aprons or thyroid shields, is a method of last resort. It provides excellent protection and should always be worn by those who work near the fluoroscopy table to limit the dose of radiation to which they are exposed. When fluoroscopy is performed, the radiation dosimeter badge is worn on the collar, outside the apron. As a result of this technique, the actual effective whole‐body exposure is up to 99% lower than the dose measured by the badge [14].
Component positioning on the C‐arm can significantly influence scattered radiation fields. The image intensifier should be as close to the patient as possible and the image should be collimated as much as possible over the area of interest. When the image intensifier is placed above the patient, and the tube is shielded by the table, both leakage and scatter radiation are minimized. Foot control must be entrusted to the operator and not to a third party, allowing better coordination, and audible alarms with a fluoroscopy locking system are very useful.
The introduction of digital radiography has contributed to the reduction of radiation exposure as well as to the improvement of image quality [15]. The combination of digital radiography with image storage systems and use of image processing systems resulted in the prevention of unnecessary image duplication and facilitated preoperative planning. A new generation of equipment has been designed with patient and operator dose reductions central to its development. Pulsed fluoroscopy and digital imaging have become available that reduce the dose of radiation delivered to the patient. Pulsed fluoroscopy should be set at the lowest possible frames per second that provide an image quality adequate for performing PCNL. Digital equipment eliminates the need for film; the information is electronically captured and manipulated on computer. As a result, the image can be enhanced, providing a sophisticated tool for dynamically evaluating patient organ function using editing techniques.
Radiation exposure can be a deleterious problem in percutaneous surgery, especially for the surgeon [16, 17]. There are two types of generalizable effects from radiation exposure: deterministic and stochastic effects. Deterministic effects are dose‐related; therefore, if you have received a high‐enough dose equivalent, certain types of effects will appear. For example, if dose exceeds 2 Gy, skin erythema will be observed. Stochastic effects are characterized by the absence of a threshold dose. Increased levels of exposure do not affect the type or severity of the effect, but do increase the probability of an effect. Risk of malignancy from radiation exposure is a stochastic effect. Common malignancies include leukemia, multiple myeloma, and thyroid, bladder, breast, lung, ovarian, and colon cancers. The National Council on Radiation Protection and Measurements has recommended an annual occupational limit of 50 mSv [18]. In medicine, there are no suggested limits for patient exposure. Instead, the risks from radiation must be balanced with the clinical necessity and benefit of the imaging study or procedure [12]. The use of ultrasonography can eliminate the side effects of radiation exposure during fluoroscopy‐guided PCNL, and it can be used as a reliable method for localization of renal stones, especially nonopaque stones that are not visible with fluoroscopy. Also B‐scan sonography can be used as a tool for localization of intrarenal arteries and avoidance of puncture by a Chiba needle.
Ultrasound versus fluoroscopy‐guided access
The advantages of ultrasound‐ over fluoroscopy‐guided access into the collecting system include reduction of exposure to radiation for the urologist and operating room personnel (see also Chapter 16 in this volume). In pregnancy and in patients with transplanted, horseshoe, or ectopic kidneys, ultrasound represents the modality of choice [19, 20]. Another advantage is proper localization of the adjacent organs for prevention of injury. The main disadvantage of this modality is the difficulty and the need for greater care when the collecting system is only mildly dilated.
Ultrasound has been used by several groups for the guidance of PCNL, especially during the puncture of the collecting system [21].The performance of puncture with ultrasound guidance and without use of fluoroscopy has also been reported [22]. While ultrasound can be a useful complement to access the kidney, it should be emphasized that fluoroscopy is an indispensable component of safe percutaneous surgery.
Preoperative images
Conventional computed tomography (CT) has been used for diagnosis of urologic diseases for many years. Recently, unenhanced helical CT has become a serious alternative to intravenous urography [23]. For preoperative planning, helical CT depicts the extent, orientation, and location of renal calculi, which are useful for access selection in percutaneous procedures. In addition, the anatomic relationships of the collecting system with surrounding organs are delineated, and the performance of a safe puncture is possible [24]. Nevertheless, the inability to provide real‐time imaging capability has prevented wider application of CT in interventional procedures [25].
The 3D reconstruction of CT images for planning of percutaneous procedures has been reported to be feasible and accurate. With the use of 3D rendering software, the anatomic relationships of the collecting system are provided, and access selection is facilitated. The usefulness of 3D‐reconstructed CT images, however, is not widely accepted [26]. 3D CT‐rendered images that combine axial CT, CT urography, and angiography have been used for preoperative planning of urologic procedures, such as nephron‐sparing surgery. Renal lesions and vessels can be accurately detected [27, 28]. Recent improvements in CT technology provide rapid and continuous reconstruction of CT raw data and provide real‐time CT imaging (CT fluoroscopy) [29, 30].
Systems as Uro Dyna‐CT (Siemens Healthcare Solutions, Erlangen, Germany) installed in the endourologic operating suite provides not only standard X‐ray and fluoroscopy but also interventional 3D imaging and cross‐sectional image reconstructions. It also offers a 3D planning and laser‐guiding puncture tool called the syngo iGuide. It may be an additional instrument that allows the urologist to handle complex punctures [31].
Magnetic resonance imaging (MRI) provides better depiction of the soft tissue in comparison with fluoroscopy and CT, but remains unreliable for the identification of stones in the collecting system or ureter. MRI can be obtained without exposure to ionizing radiation. Thus, the technique may be considered as an alternative to ultrasound in selected cases such as the pregnant patient. Nevertheless, the strong magnetic field used interferes with ferromagnetic foreign bodies or electronic medical devices that are frequently carried by patients, although improvements in electronic medical devices have minimized this problem [32]. Newly introduced multisensor detector and faster image processing systems, as well as more advanced MRI scanners, have rendered real‐time imaging a reality (MR fluoroscopy). The latter technique has been used in interventional cardiology [33]. Percutaneous nephrostomy placement has been performed with the use of open configuration MRI. MR urography represents an evolving imaging modality that allows optimal noninvasive evaluation of many abnormalities of the urinary tract. The application of several technical modifications provides adequate information for preoperative planning and postoperative assessment [34].
Each of these new technologies offers several potential advantages over the traditional percutaneous approach under fluoroscopic control. It should, however, be stated that all of these technologies are in a nascent stage of development. For that reason it is necessary to reinforce the basic concepts governing the realization of a conventional procedural approach to the kidney under fluoroscopic control. While this conventional approach is appealing, only a small percentage of urologists are familiar with it [4], and various training models are essential for consolidating the use of this surgery [35, 36].
Percutaneous renal access under fluoroscopic control
The information provided by preoperative helical CT is very valuable at the time of puncture under fluoroscopic control [37], as it identifies the most suitable place to set the path of the needle from the skin to inside the calyx that has been chosen for tapping. A CT scan can assess the presence of adjacent organs brought into the path of the needle. In this case, there is the option to change that path at the time of puncture under fluoroscopic control or to decide that percutaneous access is contraindicated.
When deciding where to make the puncture, areas of parenchyma should be considered that are thick enough to maintain a stable needle path and prevent subsequent development of a fistula. Also, it is desirable to identify those calyces for which surrounding thickness of parenchyma will promote their spontaneous closure of the puncture. Areas of kidney with an extremely thin parenchyma should be avoided.
Also, the information provided by helical CT will allow paths to be planned that avoid simple cysts, which sometimes are present in the renal parenchyma and are frequently not picked up on fluoroscopy.
The collecting system is opacified with direct injection through the ureteral catheter of contrast. A posterolateral transparenchymal puncture minimizes the chance of injury to the major renal vessels. The chosen posterior calyx is visualized with the C‐arm fluoroscopy unit in the posteroanterior direction initially. The posterior calyces are positioned 20° posteriorly to the frontal plane of the kidney in most right kidneys, and 70° posterior to the frontal plane of the kidney in most left kidneys. In a normally rotated kidney, the frontal plane of the kidney is 30° posterior to the coronal plane of the body (Figure 13.1). In general, all patients undergoing any percutaneous renal procedure are given a general anesthetic.

Figure 13.1 Schematic view of both kidneys. Right kidney: posterior calyces positioned 20° posteriorly to the its own frontal plane. Left kidney: posterior calyces positioned 70° posterior to its frontal plane.
PCNL in prone position
The patient is placed in a lithotomy position for cystoscopy, with insertion of a 6 Fr open‐end ureteral catheter under fluoroscopy guidance. A retrograde urogram then delineates the ureteral anatomy, as well as the exact stone location, degree of hydronephrosis, and the image of the selected calyx in order to plan the approach to the collecting system if this is deemed necessary [38].
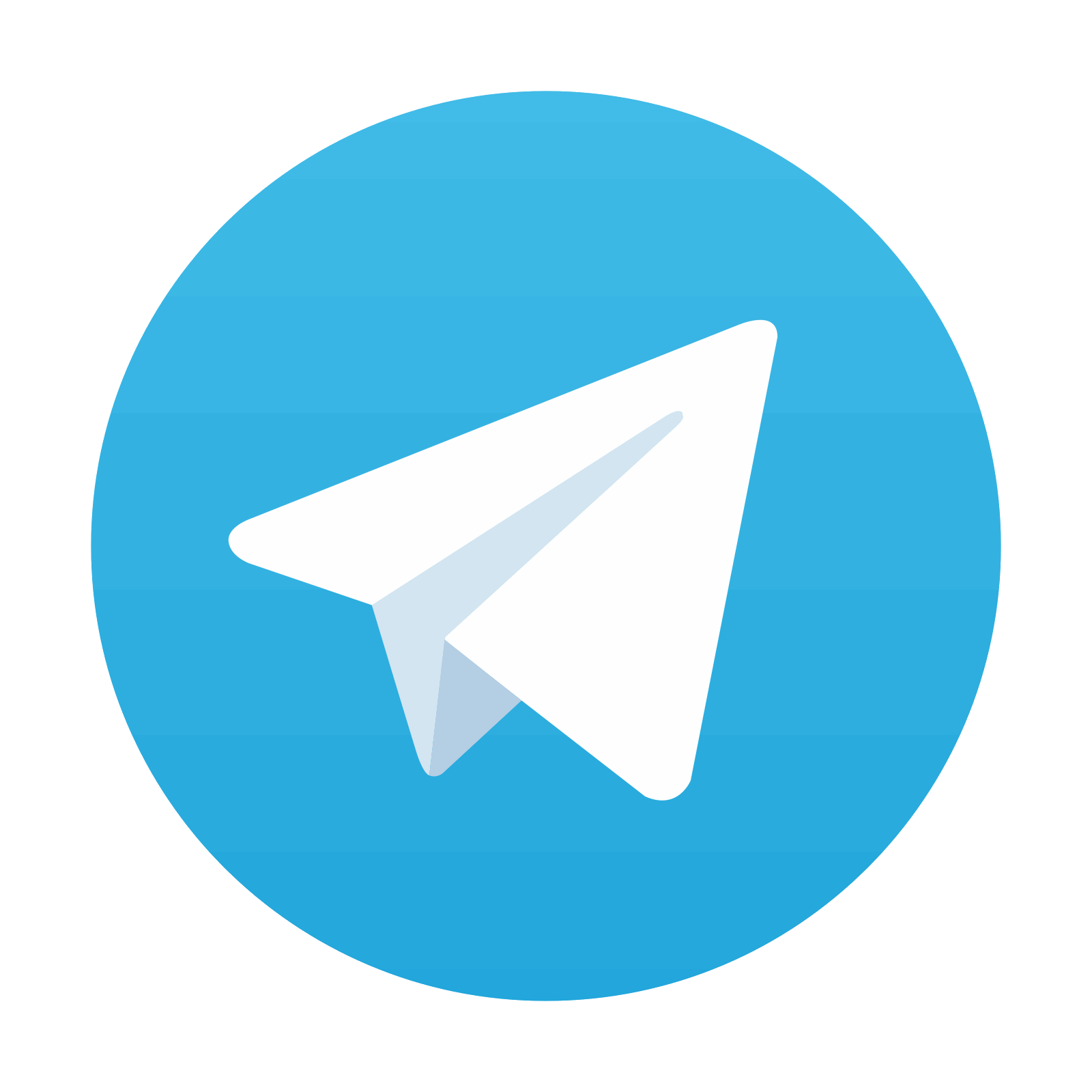
Stay updated, free articles. Join our Telegram channel

Full access? Get Clinical Tree
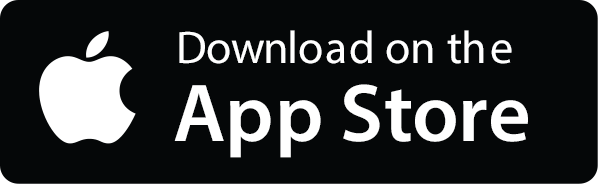
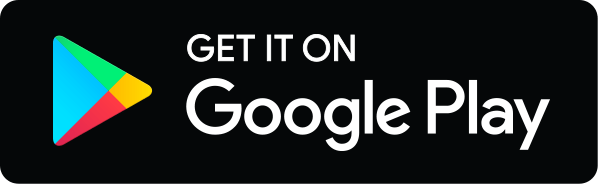