Cholesterol gallstone formation is a complex process and involves phase separation of cholesterol crystals from supersaturated bile. In most cases, cholesterol hypersecretion is considered the primary event in gallstone formation. The sterol is transported through the hepatocytic canalicular membrane by ABCG5-G8. Expression of this transport protein is regulated by transcription factor Liver X Receptor-α, which may be responsible for biliary hypersecretion. Hydrophobic bile salt pool, bile concentration, excess pronucleating mucin, and impaired gallbladder and intestinal motility are secondary phenomena in most cases but nevertheless may contribute to gallstone formation.
Gallstone disease is very common in the Western world with an estimated prevalence of 10% to 15% in white adults, leading to significant morbidity, mortality, and considerable health care costs. In the Western world, approximately 70% of gallstone carriers exhibit cholesterol gallbladder stones (cholesterol content >50%), and 30% exhibit black pigment gallbladder stones. In East Asia, there is a high prevalence of brown pigment stones residing in the bile ducts, and causing potentially devastating cholangitis. Nevertheless, also in these countries, prevalence of cholesterol gallstones increases, supposedly caused by the introduction of Western diet. Because of increased prevalence of overweight or a higher proportion of elderly subjects in the population, the prevalence of gallstone disease may further increase in the near future. This article focuses on the pathogenesis of cholesterol gallstones. Cholesterol crystal nucleation is considered the earliest step in cholesterol gallstone formation. Various conditions affecting the crystallization process are discussed, such as biliary cholesterol supersaturation, excess pronucleating proteins, or shortage of nucleation-inhibiting proteins, and factors related to the gallbladder, such as hypomotility. Pigment gallstone pathogenesis is briefly discussed.
Physical-chemical aspects of biliary cholesterol solubilization and cholesterol crystallization
Although solubility of cholesterol in aqueous solutions is extremely limited, in gallbladder bile a relatively large amount (approximately 20 × 10 −3 M) of the sterol can be kept in solution. This significant increase in solubility is explained by incorporation of cholesterol in mixed micelles, together with bile salts and phospholipids (mainly phosphatidylcholine). Supersaturation occurs when either too much cholesterol or not enough solubilizing bile salt and phosphatidylcholine molecules are secreted to allow complete micellar solubilization of all cholesterol. Excessive cholesterol may be kept in vesicles (ie, spherical bilayers of cholesterol and phospholipids, without bile salts) or in cholesterol crystals. Cholesterol crystal nucleation is thought to occur in general from vesicles supersaturated with cholesterol (ie, vesicular cholesterol/phospholipid ratio >1). First, small unilamellar supersaturated vesicles aggregate or fuse into larger multilamellar vesicles (“liquid crystals”), with subsequent phase-separation of cholesterol crystals. Pivotal information on the process of cholesterol crystal nucleation, the earliest step in cholesterol gallstone formation, has been obtained from in vitro studies in model bile systems. Wang and Carey found that cholesterol crystallization pathways and sequences in human gallbladder biles are identical to model biles matched for all relevant physical-chemical conditions, underlining the relevance of the model bile data. Based on these model bile data, the equilibrium bile salt–phospholipid–cholesterol ternary phase diagram was constructed, which allows one to predict behavior of mixtures of the three biliary lipids when present in various proportions. As shown in Fig. 1 , the phase diagram contains a bottom one-phase zone (only micelles); a left two-phase (micelles and cholesterol crystals containing) zone; a central three-phase (micelles, vesicles, and cholesterol crystals containing) zone; and a right two-phase (micelles and vesicles containing) zone. Going from baseline to top in the phase diagram the relative percentage of cholesterol increases, with progressive tendency of cholesterol crystallization as a result. Second, a shift from left to right in the phase diagram increases relative amounts of phospholipids compared with bile salts, allowing more solubilization of cholesterol in vesicles, lower vesicular cholesterol/phospholipid ratios, and less cholesterol crystallization as a result. If gallstones are present in supersaturated bile, competition may occur between the gallstone surface (gallstone growth) and the surrounding bile for available cholesterol molecules. Three factors strongly affect the ternary equilibrium bile salt–phospholipid–cholesterol ternary phase diagram, with potential consequences for in vivo cholesterol crystallization: (1) increased bile concentration, (2) increased bile salt hydrophobicity, and (3) phospholipids containing unsaturated acyl chains all strongly promote cholesterol crystallization. Corresponding effects on the ternary equilibrium bile salt–phospholipid–cholesterol ternary phase diagram are in all three cases an increase of the bottom one-phase (micellar) zone, an expansion of the cholesterol crystal–containing zones to the right, and a decrease of the vesicles-containing zones (see Fig. 1 ).
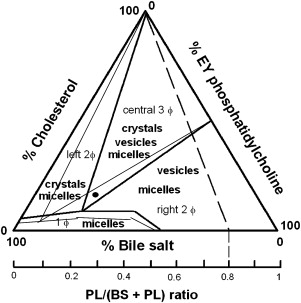
Bile concentration
Water is a major component of bile. Significant net water absorption occurs during bile transfer through the bile ducts and during prolonged storage in the gallbladder. As a result, bile water content decreases from 97% weight in the bile ducts to 90% weight in the gallbladder. This threefold to fourfold concentration of bile enhances cholesterol crystallization and gallstone formation considerably. During the process of bile formation, detergent bile salt monomers first induce formation of nascent cholesterol-phospholipid vesicles in the bile canalicular space. These vesicles are stable because they are relatively cholesterol-poor (cholesterol/phospholipid ratio <1), and cholesterol crystallization does not occur. During bile concentration in the bile ducts and gallbladder, mixed cholesterol–phospholipid–bile salt micelles are increasingly formed, because bile salt concentrations now progressively exceed critical micellar concentrations required for micelle formation. Cholesterol and phospholipid transfer then occurs from vesicles to these mixed micelles. Because solubilizing capacity of micelles for phospholipids is much higher than for cholesterol, however, there is preferential phospholipid transfer. Although fewer vesicles remain, they are now cholesterol supersaturated (ie, cholesterol/phospholipid ratio >1) and may nucleate cholesterol crystals. This sequence explains why gallstones are generally formed in the gallbladder rather than in the bile ducts.
Bile concentration
Water is a major component of bile. Significant net water absorption occurs during bile transfer through the bile ducts and during prolonged storage in the gallbladder. As a result, bile water content decreases from 97% weight in the bile ducts to 90% weight in the gallbladder. This threefold to fourfold concentration of bile enhances cholesterol crystallization and gallstone formation considerably. During the process of bile formation, detergent bile salt monomers first induce formation of nascent cholesterol-phospholipid vesicles in the bile canalicular space. These vesicles are stable because they are relatively cholesterol-poor (cholesterol/phospholipid ratio <1), and cholesterol crystallization does not occur. During bile concentration in the bile ducts and gallbladder, mixed cholesterol–phospholipid–bile salt micelles are increasingly formed, because bile salt concentrations now progressively exceed critical micellar concentrations required for micelle formation. Cholesterol and phospholipid transfer then occurs from vesicles to these mixed micelles. Because solubilizing capacity of micelles for phospholipids is much higher than for cholesterol, however, there is preferential phospholipid transfer. Although fewer vesicles remain, they are now cholesterol supersaturated (ie, cholesterol/phospholipid ratio >1) and may nucleate cholesterol crystals. This sequence explains why gallstones are generally formed in the gallbladder rather than in the bile ducts.
Bile salt hydrophobicity
More hydrophobic bile salts strongly promote cholesterol crystallization, by affecting the ternary phase diagram in a similar way as bile concentration (increased micellar cholesterol solubilization, shift of crystal-containing zones to the right, cholesterol supersaturated vesicles, promotion of cholesterol crystallization ). In the animal kingdom, human bile exhibits the most hydrophobic bile salt composition, with strong propensity to gallstone formation. Nevertheless, there is considerable variation in hydrophobicity of human bile salt composition (especially amounts of deoxycholate). In gallbladder bile of cholesterol gallstone patients, increased amounts of the hydrophobic bile salt deoxycholate are associated with fast crystallization. The primary bile salts cholate and chenodeoxycholate are synthesized from cholesterol in the liver, and secondary bile salts (mainly deoxycholate) are formed from primary bile salts in the intestine by bacterial 7α-dehydroxylase activity. Interestingly, gallstone patients exhibit larger amounts of bacteria and more 7α-dehydroxylase activity in cecal aspirates in conjunction with higher colonic pH values and prolonged small and large bowel transit times, all favoring solubilization and absorbtion of deoxycholate into the enterohepatic circulation. Because of the presence of Lith genes, male C57L inbred mice are highly susceptible to cholesterol gallstone formation during lithogenic diet, provided that a hydrophobic biliary bile salt composition (quite hydrophilic at baseline) is obtained by dietary measures (15% fat, 1% cholesterol, 0.5% cholic acid), supporting a role for bile salt composition in gallstone formation. Modulating biliary bile salt composition may have therapeutic consequences. In selected patients with cholesterol gallstones, treatment with the hydrophilic bile salt ursodeoxycholate may dissolve their stones. Under these circumstances, 30% to 60% of the total bile salt pool consists of ursodeoxycholate, with the result that cholesterol crystallization is inhibited.
Biliary phospholipid composition
In in vitro studies with model biles, phospholipid class and phospholipid acyl chain composition exert profound effects on cholesterol crystallization. Similar to increased bile concentration and increased bile salt hydrophobicity, phospholipids with more unsaturated acyl chains affect the ternary equilibrium bile salt–phospholipid–cholesterol ternary phase diagram by increasing the bottom one-phase (micellar) zone, expanding the cholesterol crystal–containing zones to the right and decreasing the vesicles-containing zones, with the result that cholesterol supersaturated vesicles and cholesterol crystallization occur (see Fig. 1 ). The underlying physical-chemical explanation for these findings is that phospholipids with saturated acyl chains by their “ trans ” configuration fit easily in the vesicular cholesterol-phospholipid bilayer, which is not the case for phospholipids with cis -unsaturated acyl chains with a bend in the molecule (leading to preferential micellar containment). Human biliary phospholipid composition is tightly regulated, however, and almost exclusively composed of phosphatidylcholine with unsaturated acyl chains (mainly 16:0 acyl chains on sn -1 position, 18:2 > 18:1 > 20:4 acyl chains on sn -2 position), contributing to human vulnerability for gallstone formation. Although modification of biliary phospholipids toward a more saturated acyl chain composition is in theory attractive, dietary modifications to accomplish this have not been successful.
Biliary nucleation promoting and inhibiting proteins
During the last decades, numerous biliary proteins have been suggested to enhance or inhibit cholesterol crystallization in gallbladder bile, based on their in vitro or ex vivo effects. Immunoglobulins M and G, haptoglobin, α 1 -acid glycoprotein, aminopeptidase-N, α 1 -antichymotrypsin, and mucin are regarded as pronucleating proteins. By contrast, human apolipoprotein A-I and IgA have been postulated to exert antinucleating activity. Cholesterol crystallization often occurs much faster in bile of patients with (especially multiple) cholesterol stones than in bile of patients with pigment stones or subjects without stones, or in model biles, even in case of comparable relative lipid composition. Excess biliary pronucleating compared with crystallization-inhibiting proteins could contribute to this phenomenon. Nevertheless, in more recent years, a growing number of publications have marshaled experimental evidence arguing against a role of most of these biliary proteins in cholesterol gallstone formation. In a recent study on a large number of gallstone patients, cholesterol saturation was an independent predictor of speed of crystallization, which was not the case for biliary protein, immunoglobulins, α 1 -acid glycoprotein, or aminopeptidase-N content. Also, Wang and coworkers showed that after the extraction of biliary lipids from human bile and their reconstitution in buffer solution, the resulting model system displayed the same speed and pattern of crystallization as the original bile sample. Of note, whereas subsequent addition of purified concanavalin A–binding glycoprotein fraction did not affect speed of cholesterol crystallization, the nucleation process was markedly enhanced by adding purified mucin. Furthermore, in the inbred mouse model, most pronucleating proteins in bile (again with the exception of mucin) were found to decrease during the earliest stages of gallstone formation, arguing against an appreciable role of these biliary proteins in gallstone pathogenesis. Mucin remains one of the few candidate proteins with a potential role in human gallstone formation. Marked hypersecretion of mucin occurs in the earliest stages of human and experimental gallstone formation. Several MUC genes are expressed in human gallbladder mucosa, including MUC1, MUC2, MUC3, MUC4, MUC5AC, MUC5B, and MUC6. Upregulation of these MUC genes could lead to the observed increased gallbladder mucin concentrations. Mucin may increase bile viscosity leading to the formation of a gel matrix that can entrap cholesterol crystals in the gallbladder. Mucin may also enhance cholesterol crystallization by offering low-affinity binding sites for cholesterol. Indeed, Lith genes have been identified that control mucin accumulation, cholesterol crystallization, and gallstone formation in the mouse model. Also, decreasing biliary mucin content with aspirin decreases risk of gallstones in the prairie dog model and risk of gallstone recurrence after nonsurgical treatment in humans. Ursodeoxycholic acid also decreases biliary mucin contents.
Gallbladder and intestinal motility
Meal ingestion induces considerable gallbladder emptying (up to 70%–80% of fasting gallbladder volumes) by releasing the hormone cholecystokinin from the upper intestine. Impaired gallbladder emptying may prolong residence of bile in the gallbladder, allowing more time for nucleation of cholesterol crystals from supersaturated bile. Furthermore, in case of adequate emptying, cholesterol crystals that have nucleated may be ejected to the duodenum, whereas in case of impaired gallbladder emptying, these crystals may aggregate into macroscopic gallstones. Several studies have shown that gallstone patients may be divided into a group with severely impaired or even absent postprandial emptying (“bad contractors”) and a group with good postprandial gallbladder emptying (“good contractors”). Patients with good postprandial contraction often have increased fasting and residual gallbladder volumes compared with normal controls. Prospective studies also indicate that impaired postprandial gallbladder motility is an independent risk factor for gallstone recurrence after successful treatment with extracorporeal shockwave lithotripsy. It is less well appreciated that significant periodic gallbladder emptying also occurs during the fasting state (20%–30% emptying in the fasting state vs 70%–80% emptying after a meal) at 1- to 2-hour intervals, associated with the cycle of the intestinal migrating motor complex and with a rise of plasma motilin levels. It has been found that gallstone patients show a pattern of less frequent migrating motor complex cycles, with absent interdigestive gallbladder emptying and altered motilin release compared with controls. A similarly prolonged migrating motor complex cycle has been found in the ground squirrel model of gallstone formation. The fasting state (ie, the night) seems to be the most vulnerable period for gallstone formation. During this period, biliary cholesterol saturation is highest, because of relatively low bile salt secretion and relatively high cholesterol secretion. There is also a progressive concentration of gallbladder bile during this period, which is partially counteracted by periodic interdigestive gallbladder contraction in association with antral phase 3 of the migrating motor complex of the intestine.
There is increasing insight into pathogenesis of impaired gallbladder motility. Significant absorbtion of cholesterol seems to occur from supersaturated bile in the gallbladder. Excess cholesterol is then incorporated within the sarcolemmal plasma membrane of the gallbladder smooth muscle cell, with decreased membrane fluidity, impaired contractility, and impaired relaxation as a result. In addition, the gallbladder wall is exposed to detergent bile salts, unesterified cholesterol, and bacteria. As a result, a proinflammatory Th1 immune response may occur, which contributes to hypomotility. Although impaired motility could be in many cases secondary to biliary cholesterol supersaturation, it may still facilitate the process of gallstone formation. Gallbladder motility is often impaired in high-risk situations for gallstone formation, such as pregnancy, obesity, diabetes mellitus, gastric surgery, treatment with the somatostatin analogue octreotide, very low calorie dieting, and total parenteral nutrition.
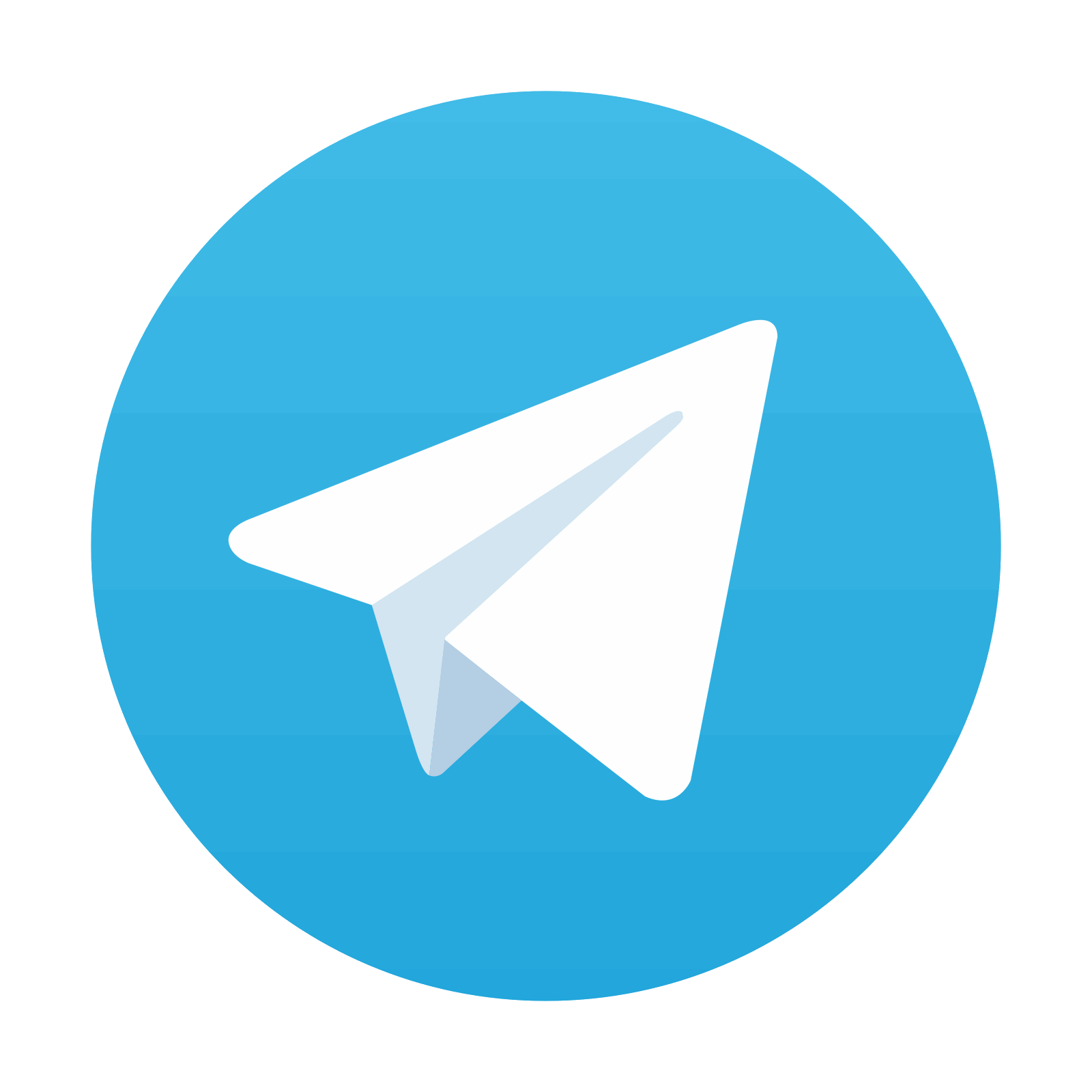
Stay updated, free articles. Join our Telegram channel

Full access? Get Clinical Tree
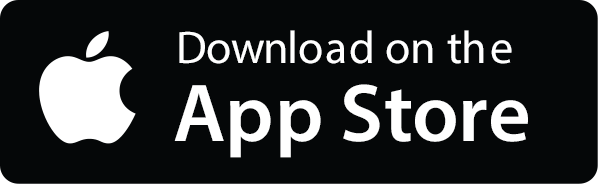
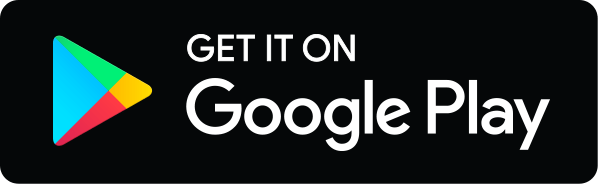