Fig. 4.1
A schematic diagram showing the structure and functional unit of the exocrine pancreas . The acinus is the functional unit of the exocrine pancreas, which is composed of contiguous pyramid-shaped glandular cells with their apex toward the lumen of the acinus. Acinar cells have well-developed Golgi and rough endoplasmic reticulum complexes, essential for synthesizing and storing large amounts of secretory proteins. Zymogens or storage granules can be found in the apical or luminal side of the cell
Mechanism of Protein Secretion by the Acinus
The primary function of the pancreatic acinar cell is to produce large amounts of digestive enzyme proteins that are eventually transported through the ductal system into the duodenum to be mixed with intestinal chyme. The cellular events involved in the synthesis and export of these proteins have been well-characterized. Proteins for export are synthesized on polysomes attached to the outer or cytosolic aspect of the rough endoplasmic reticulum located at the base of the acinar cell. A special signal sequence after the AUG initiation codon is translated into an amino-terminal extension called the signal peptide (Fig. 4.2). The signal peptide is avidly bound by a cytosolic protein called the signal–peptide recognition particle (SRP), which facilitates the binding of the mRNA-ribosomal complex to the endoplasmic reticulum (ER) membrane. It does so by recognizing and binding to a specific ER-membrane receptor or docking protein. Because of the hydrophobicity of the signal peptide, it enters the internal ER compartment. As translation continues, the rest of the protein traverses the ER membrane and enters the cisternal space. The signal peptide is then cleaved off by an enzyme called signal peptidase . The nascent protein then undergoes several post-translational modifications, including the formation of disulfide bridges, glycosylation, sulfation, and phosphorylation. Post-translational processing of secreting proteins is important in folding them into proper tertiary and quaternary configurations. Within 20–30 min of their synthesis, these proteins are transferred to the Golgi complex, where additional processing of the secretory proteins takes place. These modifications generally involve the removal of mannose groups from glycoproteins and progressive buildup to a complex glycosylated form by sequential additions of monosaccharides . These glycoproteins move from the cis to trans side of the Golgi complex and are eventually concentrated and packaged into storage granules. The secretory granules then move by an undefined mechanism to the apical portion of the acinar cell. Upon an appropriate neural of hormonal stimulus, zymogen granules move to the apical membrane, fuse with the plasma membrane, and discharge their contents into the luminal space by the process of exocytosis (Fig. 4.3).
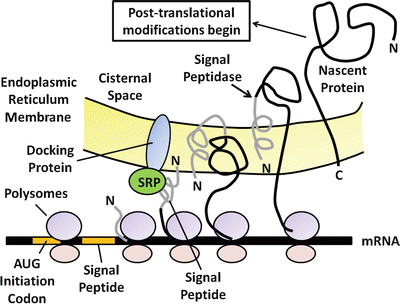
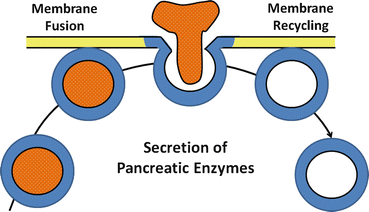
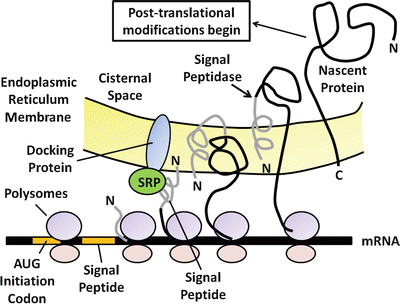
Fig. 4.2
The processing and synthesis of pancreatic digestive enzymes. Proteins for export are first synthesized on polysomes attached to the outer or cytosolic aspect of the rough endoplasmic reticulum at the base of the acinar cell. As translation continues, the nascent protein transverses the endoplasmic reticulum membrane and enters the cisternal space
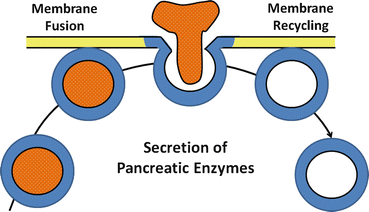
Fig. 4.3
The process of exocytosis of pancreatic enzyme secretion. After an appropriate neural or hormonal stimulus, zymogen granules move to apical membrane, fuse with plasma membrane, and discharge their contents into the luminal space by the process of exocytosis
Regulation of Digestive Enzyme Secretion
Several intracellular messengers appear to play a role in regulating the secretion of digestive enzymes. Increases in intracellular calcium are stimulated by agents such as cholecytokinin (CCK), acetylcholine , bombesin (gastrin -releasing peptide or GRP is the mammalian equivalent), and substance P. Although receptors on acinar cells for all these agents have been identified, it is likely that cholinergic muscarinic receptors are the major pathway for regulation (discussed below). These agents stimulate phosphatidylinositol (PI) metabolism, leading to the formation of inositol 1,4,5-triphosphate (IP3) and 1,2-diacylglycerol (DAG) (Fig. 4.4). IP3 activates IP3 receptors of calcium storage organelles, releasing Ca2+ and increasing cytosolic Ca2+. Though being transient, this stimulation serves to trigger a number of more sustained biochemical and functional events. Increased Ca2+ activates calcium-dependent, constitutively expressed nitric oxide synthase (NOS), which produces nitric oxide (NO) from L-arginine . The activity of this enzyme also appears to be sensitive to the level of intracellular calcium stores, as depletion of these stores can independently activate NOS activity. NO then appears to stimulate soluble guanylate cyclase activity to increase cellular cyclic guanosine monophosphate (cGMP) levels. cGMP stimulates increased plasma-membrane permeability to Ca2+ to sustain increased cytosolic Ca2+, possibly through activation of a cGMP-dependent cation channel or through the action of cGMP-dependent protein kinases. Increases in cytosolic Ca2+ stimulate exocytosis of zymogen granules, probably through calcium-calmodulin-dependent protein kinases. The activation of protein kinase C by DAG may also play a role in stimulating acinar cell secretion, particularly in sustaining the effect after receptor activation.
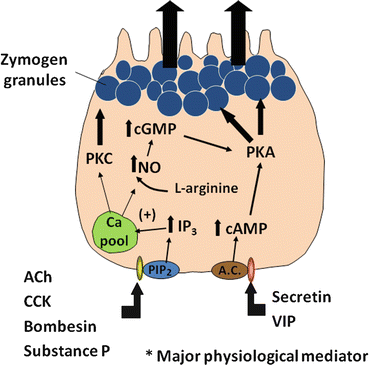
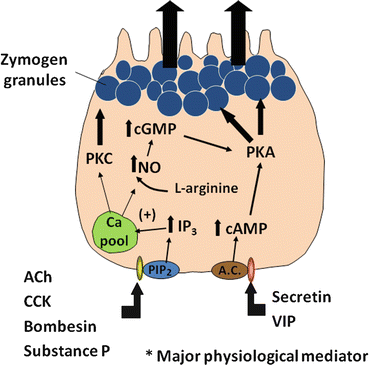
Fig. 4.4
A cellular model showing the regulation of protein secretion by a pancreatic acinar cell. Upon stimulation by agonists such as cholecystokinin (CCK), secretin , vasoactive intestinal peptide (VIP), and acetylcholine (ACh), signal transduction pathways are evoked in the pancreatic acinar cell. ACh and CCK stimulate acinar cell secretion by activating inositol triphosphate (IP3)/diacyl glycerol (DAG) signaling pathways, thus leading to increased cytosolic Ca2+ and protein kinase C (PKC). Secretin and VIP stimulate secretion by elevating intracellular cAMP, thereby activating protein kinase A (PKA)
Synchronization of Secretory Responses
Recently, the role of intercellular gap junctions in “synchronizing” acinar cells of a functional unit has become evident. Gap junctions provide points of low electrical resistance and for intercellular permeation by small-charge molecules such as IP3 or Ca2+. Local application of agonists to one region of an acinar unit, for example, stimulates a regional increase in cellular Ca2+. However, the activation of other cells of the unit soon becomes apparent, most likely through the propagation of an activating signal via gap junctions. Physiologically, this intercellular coupling mechanism provides an effective way to produce a unified secretory response by the acinar unit.
The relative importance of the above Ca2+-dependent agents for the physiological regulation of pancreatic functions is not entirely clear (see discussion at greater length later in the chapter). Experimentally, these agents do appear to have different effects on acinar cell function, even though they all appear to activate PI metabolism. This could be attributed to differences in their effects on PI metabolism or on receptor coupling with other transduction pathways. For instance, acetylcholine and CCK vigorously stimulate IP3 and DAG, the latter eventually desensitizing both receptors. Bombesin, on the other hand, has far less effect on DAG formation and does not cross-desensitize receptor activation by CCK or acetylcholine. As another example, CCK, but not acetylcholine, has trophic effect on pancreatic acinar cells . This may arise from CCK-receptor coupling with the phospholipase D pathway, which causes the formation of phosphatidic acid and choline, important for mediating CCK trophism.
Agents such as secretin and vasoactive intestinal peptide (VIP) also stimulate acinar cell secretion, apparently through the activation of adenylate cyclase and increased cyclic adenosine monophosphate (cAMP). Although the exact mechanisms that result in enzyme secretion are not well understood, cAMP-dependent protein kinases are probably involved. The physiological role of cAMP-mediated agonists in regulating acinar cell functions is also not well characterized, although it is believed to be less important than calcium-mediated agonists. However, these agents do appear to augment or potentiate the actions of Ca2+-mediated agonists (Discussion below).
Finally, it should be noted that microtubules or microfilaments of the actin–myosin system play an important role in the movement of secretory granules to the site of exocytosis. For example, cytochalasin B, an inhibitor of the microfilament system, inhibits the exocytic process. After discharge of the contents of the zymogen granules, excess membrane can be retrieved by the cell and reutilized. Several lines of evidence suggest that this reutilization process involves endocytosis and relocation of these internalized membranes to the trans side of the Golgi complex and to lysosomes.
2.2 Protein Secretion Essential for Digestion
The exocrine pancreas makes and secretes a variety of proteins, most of which are essential for digestion and absorption of ingested nutrients (Fig. 4.5). However, if these proteins were secreted as active enzymes within the parenchyma of the pancreas, the consequences would be potentially disastrous, as extensive tissue destruction would result (i.e. autodigestion). To prevent autodigestion, the pancreas protects itself in several ways. First, all potentially harmful enzymes are made in an inactive or proenzyme form and are packaged in zymogen granules within acinar cells . Secreted enzymes remain inactive until their reach the duodenal lumen (Fig. 4.6a). Second, in the duodenum, trypsinogen, a major proteolytic enzyme, is converted to active trypsin by an enzyme, called enterokinase , a brush-border enzyme expressed by duodenal mucosa . Trypsin catalyzes the activation of more trypsin through hydrolysis of the N-terminal hexapeptide of the trypsinogen molecule, thus rapidly accelerating the entire process. Active trypsin is also essential for activation of several other proteolytic and lipolytic pancreatic enzymes. Thus, the activation site for potentially destructive enzymes is geographically removed from the pancreas and compartmentalized within the duodenal lumen (Fig. 4.6b). Finally, acinar cells make trypsin inhibitor, which is packaged with trypsinogen in zymogen granules. Its role is to activate small amount of trypsin that may form within cells or the body of the pancreas.
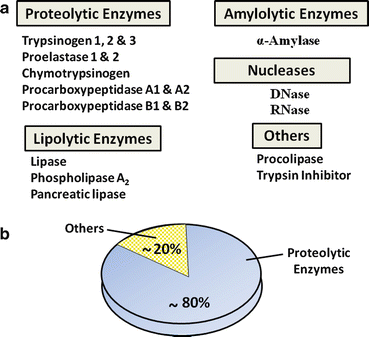
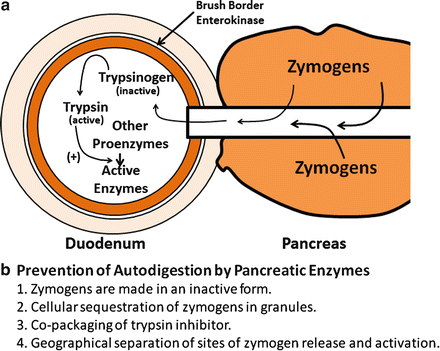
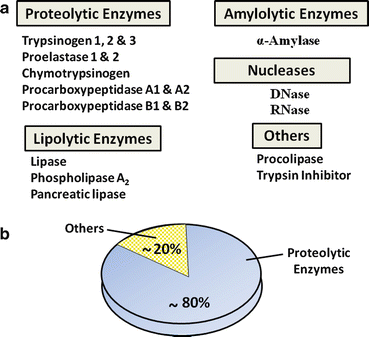
Fig. 4.5
Common proteins of human pancreatic juice. (a) These secreted proteins are essential for digestion and absorption of ingested nutrients. (b) Proteolytic enzymes make up the majority of proteins that are secreted by pancreatic acinar cells
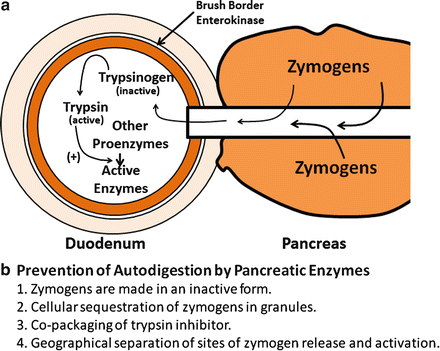
Fig. 4.6
Mechanisms and sites of activation of pancreatic zymogens. (a) Secreted enzymes remain inactive until they reach the duodenal lumen. Trypsinogen, a major proteolytic enzyme, is converted to active trypsin by brush-border enterokinase . Trypsin catalyzes the activation of more trypsin, thus accelerating the entire process. Active trypsin is also essential for activation of other proteolytic and lipolytic pancreatic enzymes. (b) Mechanisms for preventing autodigestion are shown
Pancreatic juice contains several kinds of hydrolytic enzymes capable of digestive micronutrients (proteins, peptides, fats, carbohydrates, and nucleic acids) into their basic subunits. Proteolytic enzymes make up almost 80 % of all protein found in pancreatic juice (Fig. 4.5b). These include endopeptidases (cleaving internal peptide linkages) such as trypsin and exopeptidases (cleaving from ends of proteins) such as carboxypeptidases . Other enzymes include nucleases, amylase (digestion of carbohydrates), and those involved in lipid digestion such as lipase , phospholipase A 2 , and carboxylesterase. The functional aspects of these enzymes will be discussed in greater depth in the sections on nutritional physiology.
3 Fluid and Electrolyte Transport of the Pancreas
The basal volume of pancreatic secretion is estimated to be 0.2–0.3 mL/min, although, when stimulated, pancreatic secretion can reach 4.0–4.5 mL/min. The daily output of pancreatic juices into the duodenal lumen in humans is approximately 2.5 L/day. The fluid secreted by the pancreas represents the combined secretions of both duct and acinar cells , but the compositions of these secretions differ significantly. Acinar cells secrete a plasma-like fluid that is predominantly sodium chloride (Fig. 4.7). The rate of acinar cell fluid secretion is dependent on the rate of enzyme secretion, and the maximal flow rate is much less than that seen in ducts. The primary function of acinar cell fluid secretion therefore is to transport secreted enzymes into the duct system.
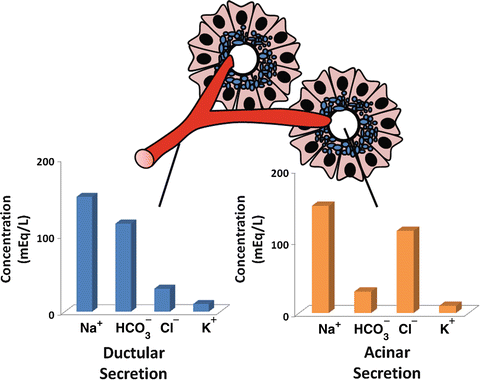
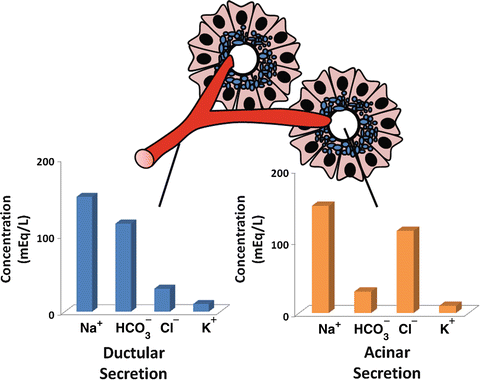
Fig. 4.7
Difference in ionic composition of ductular and acinar fluid. Acinar secretion is a plasma-like fluid that is predominantly sodium chloride. Ductular secretion is predominantly sodium bicarbonate
Duct cells , on the other hand, secrete a bicarbonate-rich fluid, at a considerably variable flow rate of 0–4.0 mL/min depending on the state of pancreatic stimulation. The purpose of the alkaline secretion is to neutralize gastric acid that enters the duodenum, a process essential for achieving optimal conditions for pancreatic enzyme activity. Inadequate bicarbonate secretion with failure to reach a neutral liminal pH, as occurs in chronic pancreatitis , contributes to maldigestion of ingested nutrients seen in this condition.
< div class='tao-gold-member'>
Only gold members can continue reading. Log In or Register a > to continue
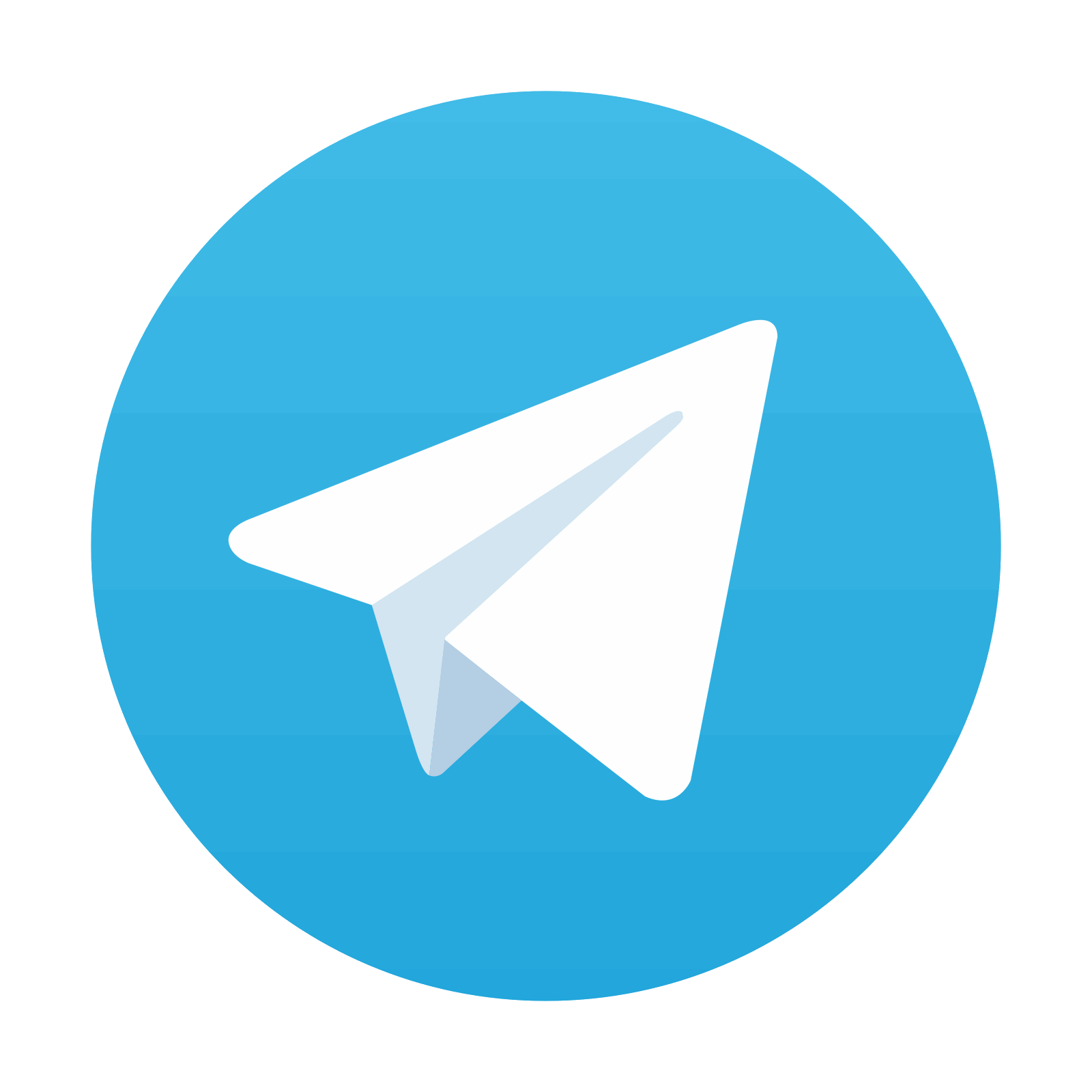
Stay updated, free articles. Join our Telegram channel

Full access? Get Clinical Tree
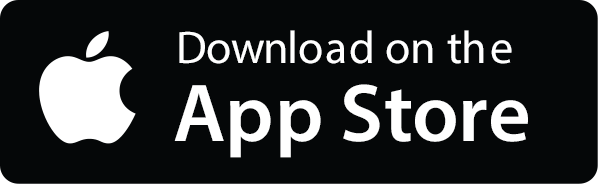
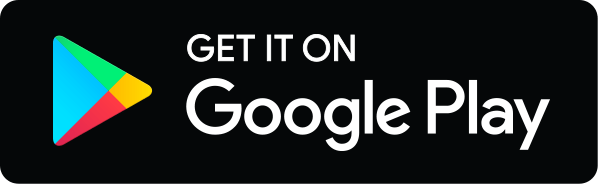