Condition
Affected gene(s)
Reference
Cystic fibrosis
CFTR gene
[1]
Shwachman–Diamond syndrome
SBDS gene
[2]
Johanson–Blizzard syndrome
UBR1 gene
[3]
Pearson marrow–pancreas syndrome
Mitochondrial DNA defects
[4]
Pancreatic agenesis
PDX 1 gene
[5]
Pancreatic agenesis and congenital heart defects
Heterozygous mutations in the GATA6 gene
[6]
Pancreatic and cerebellar agenesis
PTF1A gene
[7]
Pancreatic lipase deficiency
Unknown
[8]
Pancreatic co-lipase deficiency
Pancreatic lipase and co-lipase deficiency
Hereditary pancreatitis
PRSS1 gene
[9]
SPINK 1 gene
[10]
CFTR gene
[11]
Chymotrypsin C (CTRC) variants
[12]
Pseudohypoparathyroidism Type IA
GNAS 1 gene
[13]
CoQ-responsive Oxphos deficiency
Unknown
[14]
Exocrine pancreatic insufficiency, dyserythropoietic anemia, and calvarial hyperostosis
COX 412 gene
[15]
Pancreatic Insufficiency in Cystic Fibrosis
Cystic fibrosis (CF) is an autosomal recessive condition caused by defects in the CF transmembrane regulator (CFTR) gene. About 85 % of patients with CF are pancreas insufficient (PI) [16].
Pathophysiology of Pancreatic Insufficiency in Cystic Fibrosis
Mutations of the CFTR gene cause impaired chloride transport at the apical surface of epithelial cells [17] and also disturb chloride-coupled bicarbonate transport [18] and sodium channel activity [19]. Pancreatic secretion of chloride, bicarbonate, sodium , and potassium in response to combined cholecystokinin and secretin stimulation is impaired in all patients with CF, regardless of pancreatic function status [16]. Bicarbonate secretion is most impaired, and defective electrolyte secretion leads to reduced fluid secretion [20]. This defective bicarbonate secretion results in impairment in the luminal flow of pancreatic enzymes and proenzymes and impairment in the trafficking of zymogen granules [20]. This results in a severe block in acinar cell secretion followed by loss of cellular function, cell death, fibrosis, and eventual pancreatic insufficiency which leads to a decline in all the enzymes secreted by the pancreas [17, 20] .
In normal people, just 5–10 % of the normal post-meal pancreatic enzyme output is adequate for normal digestion, indicating the large reserve capacity of the pancreas [17]. This reserve capacity means that clinically significant malabsorption is not evident until at least 90 % of the exocrine cells of the pancreas are destroyed [21]. Again in normal individuals, the presence of free fatty acids in the proximal small bowel causes release of cholecystokinin which in turn stimulates pancreatic secretion [22]. When pancreatic insufficiency begins to develop, this feedback loop is impaired, and the site of maximal digestion shifts to the more distal bowel [17]. This results in larger amounts of nutrients being delivered to the distal bowel with changes in motor and secretory function of the more proximal bowel [23, 24]. These changes, in turn, lead to quicker intestinal transit and worsening of malabsorption [24].
The terms pancreas sufficient (PS) and PI have great clinical and prognostic significance in CF. PS does not mean normal pancreatic function, but that enough pancreatic function is present to avoid the need for pancreatic enzyme replacement therapy (PERT) . Patients who are PS are more prone to pancreatitis [25], while PI patients have more severe lung disease, malnutrition , and liver disease [26] .
Shwachman–Diamond Syndrome
Shwachman–Diamond syndrome (SDS) is an autosomal recessive disorder characterized by congenital anomalies, pancreatic insufficiency, bone marrow failure, and predisposition to myelodysplasia and acute myeloid leukemia (AML) [27]. Mutations in the Shwachman–Bodian–Diamond syndrome (SBDS) gene can be found in approximately 90 % of classically presenting patients with SDS [28]. These mutations usually result in reduced, but not absent, protein expression. In mice, targeted deletion of the gene results in embryonic death suggesting that some expression of this gene is necessary for survival [29]. The SBDS gene is involved in ribosomal function [30], and ribosomal subunit assembly is impaired in patients with SDS [31]. The exact mechanism by which this leads to pancreatic insufficiency is unknown . One study found that patients negative for mutations in the SBDS gene may have more severe hematological manifestations while having milder pancreatic disease [32].
The classic presentation of SDS is in infancy with failure to thrive, diarrhea, and neutropenia. SDS infants have an average birth weight at the 25th percentile [34]. Table 39.2 details the criteria for the diagnosis of SDS. Growth failure with malnutrition is common in the first year of life, and height falls and remains below the third percentile in 38–56 % of patients [35, 36]. After diagnosis, and with appropriate therapy, most children show normal growth velocity, and it appears that with current treatment they attain normal body mass indexes (BMIs) [35]. Steatorrhea is caused by decreased secretion of lipolytic and proteolytic enzymes, while ductular fluid and electrolyte secretion of the pancreas remain normal [37, 38] . Pancreatic insufficiency tends to be diagnosed within the first 6 months of life with 90 % of patients being diagnosed in the first year [37]. Spontaneous improvement in pancreatic function can occur in later childhood with 50 % of patients having normal fat absorption by age 4 years and no longer requiring pancreatic enzyme supplementation [37]. The pancreas in SDS exhibits a characteristic fatty replacement which can be visualized on ultrasound, CT scanning, and, perhaps, best by MRI scanning [39] .
Table 39.2
Diagnostic criteria for Shwachman–Diamond syndrome. (Reproduced with permission John Wiley and Sons/New York Academy of Sciences, from Ref. [34], © 2011 New York Academy of Sciences)
Clinical and molecular diagnostic criteria |
Diagnostic criteria |
Clinical diagnosis |
Fulfill the combined presence of hematological cytopenia of any given lineage (most often neutropenia) and exocrine pancreas dysfunction |
Hematologic abnormalities may include: |
a. Neutropenia < 1.5 × 109/L on at least two occasions over at least 3 months |
b. Hypoproductive cytopenia detected on two occasions over at least 3 months |
Tests that support the diagnosis but require corroboration: |
a. Persistent elevation of hemoglobin F (on at least two occasions over at least 3 months apart) |
b. Persistent red blood cell macrocytosis (on at least two occasions over at least 3 months apart), not caused by other etiologies such as hemolysis or a nutritional deficiency |
Pancreatic dysfunction may be diagnosed by the following: |
a. Reduced levels of pancreatic enzymes adjusted to age (fecal elastase, serum trypsinogen, serum (iso)amylase, serum lipase) |
Tests that support the diagnosis but require corroboration: |
a. Abnormal 72-h fecal fat analysis |
b. Reduced levels of at least two fat-soluble vitamins (A, D, E, K) |
c. Evidence of pancreatic lipomatosis (e.g., ultrasound, CT, MRI, or pathological examination of the pancreas by autopsy) |
Additional supportive evidence of SDS may arise from: |
a. Bone abnormalities |
b. Behavioral problems |
c. Presence of a first-degree family member diagnosed before with SDS |
Other causes of pancreatic insufficiency should be excluded, in particular when the SBDS gene mutation analysis is negative |
Molecular diagnosis: biallelic SBDS gene mutation |
Positive genetic testing for SBDS mutations known or predicted to be deleterious, for example, from protein modeling or expression systems for mutant SBDS |
Caveats: |
Many situations arise when molecular diagnosis is not confirmatory in the presence of clinical symptoms: |
No identified mutations (about 10 % of cases) |
Mutation on one allele only |
Gene sequence variations that have unknown or no phenotypic consequence: |
A novel mutation, such as a predicted missense alteration, for which it is not yet possible to predict whether it is disease causing |
SBDS polymorphisms on one or both alleles. Large popluation studies may be needed to exclude a sequence polymorphism as a bona fide irrelevant variant |
Hepatomegaly and raised serum liver enzymes are common in children with SDS [40]. These resolve by the age of 5 years and no long-term consequences have been observed [40].
Neutropenia is the most common cytopenia and can be persistent or intermittent and mild to severe [34]. Anemia with low reticulocyte counts [34] and elevation in fetal hemoglobin is each seen in 80 % of patients [41]. Thrombocytopenia can also be seen. Bone marrow biopsy is usually hypoplastic with increased deposition of fat [41, 42]. Patients with SDS have a propensity to developing infections due to the neutropenia and the occasional functional neutrophil deficits that are seen in SDS [43]. Patients with SDS develop clonal changes in the bone marrow which may or may not be associated with an increased risk of myelodysplasia or AML [23]. Due to the predisposition to myelodysplasia and AML, all patients with SDS should be referred to a pediatric hematologist. Based on data from several registries, the frequency of both myelodysplasia and AML increases with increasing age [32, 44, 45]. In general, early bone marrow transplantation in children with severe cytopenias prior to development of myelodysplasia or AML improves outcome.
The bony dysplasia of SDS manifests as short stature and delayed appearance but subsequent normal development of secondary ossification centers [34]. There is variable metaphyseal widening and irregularity that is most often seen in the ribs in early childhood and femurs later in childhood and adolescence [46]. Rarely, skeletal involvement may be extremely severe and generalized [34]. Usually, these metaphyseal changes become clinically insignificant, but rarely they may lead to limb deformities and fractures [46] .
A characteristic pattern of neurocognitive and behavioral difficulties has been described in SDS [47].
A high degree of suspicion may be needed to diagnose milder cases of SDS. A study of 37 children with SDS found that neutropenia (81 %), diarrhea (58 %), failure to thrive (73 %) lipomatous infiltration of the pancreas (~ 90 %), fecal elastase (82 %), skeletal (38 %), and congenital and endocrine malformations (65 %) were all inconsistently present [48].
Serum immunoreactive trypsinogen (IRT) and pancreatic isoamylase concentrations can be useful markers of the pancreatic phenotype in SDS [49]. In healthy children, serum IRT concentrations are at adult levels at birth, while pancreatic isoamylase concentrations are low at birth and reach adult levels by 3 years of age [49]. In contrast, in SDS, young children have low serum IRT concentrations which then rise with age, while serum pancreatic isoamylase activities are low at all ages. Serum IRT is generally low in PI patients with SDS, while a normal value does not rule out pancreatic insufficiency. Serum isoamylase concentrations are not useful in determining pancreatic sufficiency or insufficiency . Hence, when SDS is suspected, a serum IRT should be obtained in children < 3 years of age, while serum pancreatic isoamylase should be obtained in children ≥ 3 years of age [34] .
The diagnosis of SDS is made using the criteria shown in Table 39.3. The combination of exocrine pancreatic dysfunction and hematological abnormalities when other known causes of exocrine pancreatic dysfunction and bone marrow failure are excluded gives rise to a clinical diagnosis of SDS [34]. CF should be ruled out with a sweat test while Pearson marrow-pancreas syndrome can be differentiated by a bone marrow examination and imaging of the pancreas. Cartilage hair hypoplasia which presents with diarrhea (but not with pancreatic insufficiency), cytopenia, and metaphyseal chondrodysplasia is more common in certain populations such as the Amish.
Table 39.3
FDA-approved pancreatic enzyme products
Dosages available (lipase/protease/amylase units) | Bead/microsphere diameter (mm) | Notes | |
---|---|---|---|
Creon® | 3000/9500/15,000 | 0.7–1.6 | Oral, delayed-release capsules |
6000/19,000/30,000 | |||
12,000/38,000/60,000 | |||
24,000/76,000/120,000 | |||
Pancreaze® | 4200/10,000/17,500 | 2 | Oral, delayed-release capsules |
10,500/25,000/43,750 | |||
16,800/40,000/70,000 | |||
21,000/37,000/61,000 | |||
Pertyze® | 8000/28,750/30,250 | 0.8–2.2 | Oral, delayed-release capsules with bicarbonate-buffered enteric-coated microspheres |
16,000/57,500/60,500 | |||
Ultreza™ | 13,800/27,600/27,600 | 2.0–2.4 | Oral, delayed-release capsules |
20,700/41,400/41,400 | |||
23,000/46,000/46,000 | |||
Viokace™ | 10,440/39,150/39,150 | N/A | Non-enteric-coated tablets |
20,880/78,300/78,300 | Approved only for use in adults | ||
Must be given with a proton pump inhibitor | |||
Zenpep® | 3000/10,000/16,000 | 1.8–1.9 for 3000 and 5000 | Oral, delayed release capsules |
5000/17,000/27,000 | |||
10,000/34,000/55,000 | |||
15,000/51,000/82,000 | |||
20,000/68,000/109,000 | |||
25,000/85,000/136,000 | |||
2.2–2.5 for others | A generic product is available at the 5000 lipase unit dose |
Pearson Marrow–Pancreas Syndrome
Pearson described a syndrome of refractory, transfusion-dependent sideroblastic anemia with vacuolization of the bone marrow and exocrine pancreatic insufficiency [4] . Other variable features may include hepatic failure, proximal renal tubulopathy, watery diarrhea, patchy erythematous skin lesions, neutropenia, and thrombocytopenia and high serum lactate/pyruvate ratios [50]. This condition should be considered in the differential diagnosis of Shwachman syndrome. In this syndrome, vacuolization of the marrow is seen, while in Shwachman syndrome the bone marrow is dysplastic; the pancreas is fibrotic in Pearson syndrome, while it is fatty in Shwachman syndrome [51]. Pearson syndrome is caused by mitochondrial DNA alterations which are more abundant in the blood than in other tissues [50]. Diagnosis is suspected based on clinical findings and can be confirmed by Southern blot analysis which detects rearrangements of mitochondrial DNA [52]. This syndrome is usually fatal in infancy, but some children who survive past infancy develop severe neurological symptoms suggestive of another mitochondrial DNA disorder, Kearns–Sayre syndrome [53]. In children without multisystem involvement, bone marrow transplantation or unrelated cord blood cell transplantation has been suggested as a mechanism to manage the severe hematological manifestations of this syndrome [52] .
< div class='tao-gold-member'>
Only gold members can continue reading. Log In or Register a > to continue
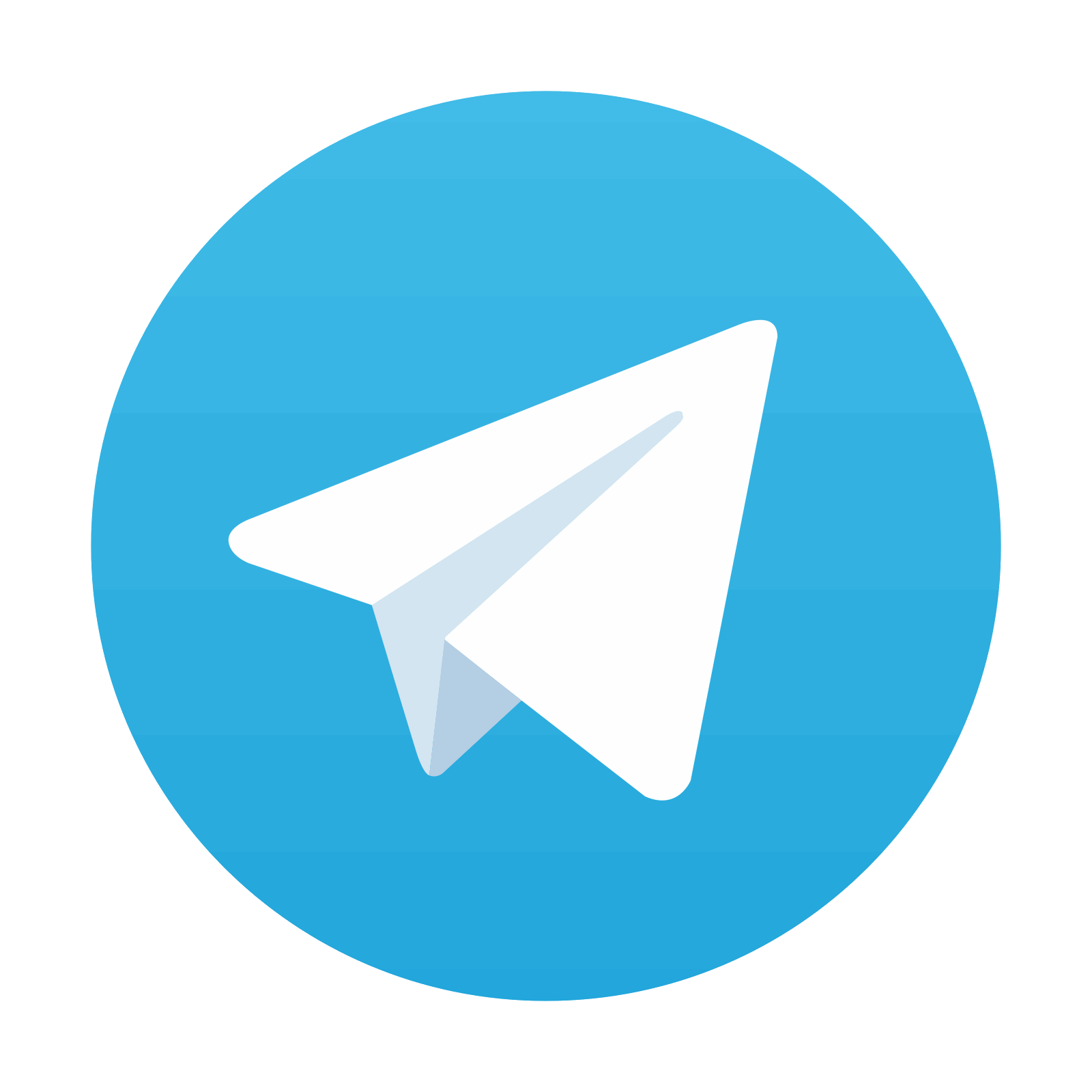
Stay updated, free articles. Join our Telegram channel

Full access? Get Clinical Tree
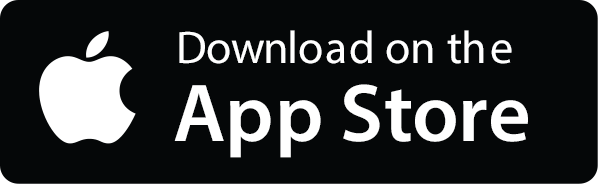
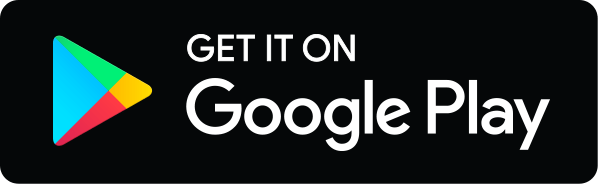