Abstract
The Notch signaling pathway is a key regulator of the intestinal epithelium, playing a fundamental role in stem cell maintenance, progenitor cell proliferation, and cell fate determination. Notch controls iterative functions in the developing intestine and in adult tissue homeostasis, regulating the balance of proliferation vs. differentiation. This chapter first summarizes the canonical Notch signaling pathway and its regulation and then reviews the current view of Notch function in the intestine, focusing on the numerous mouse models that have been generated with Notch pathway phenotypes. In addition, there is consideration of intestinal stem cells and other progenitor cell populations involved in the Notch-directed specification of cell fate to the absorptive enterocyte lineage over the secretory cell lineage (goblet, Paneth, enteroendocrine, tuft). Notch pathway involvement in human disease is briefly discussed, highlighting the challenge of therapeutic disruption of the Notch pathway due to severe intestinal toxicity.
Keywords
Notch signaling, Intestinal stem cell, Goblet cell, Paneth cell, Enteroendocrine cell, Tuft cell, Atoh1, Hes1, Adam10
6.1
Notch Pathway Overview
The Notch pathway is an evolutionarily conserved signaling pathway present in all metazoans that influences a wide range of developmental and physiological processes, including the maintenance of self-renewing adult cells and tissues. Since Notch is a critical regulator of proliferation and differentiation in both development and tissue homeostasis, it is not surprising that dysregulation of Notch activity or mutations within the Notch signaling pathway have been linked with inherited human disorders as well as cancer. First named after a Drosophila partial loss-of-function mutation that resulted in “irregular notches” in the wing margin, the Notch pathway has been the focus of numerous studies in worms, flies, and mammals. Canonical Notch signaling mediates direct cell-to-cell communication to establish differential cell processes in neighboring cells ( Fig. 6.1 ). Activation of the Notch pathway involves direct physical contact between cells expressing membrane-bound ligands (signal sending) and cells expressing Notch receptors (signal receiving). Ligand-binding activates sequential proteolytic processing of the Notch receptor to release the Notch receptor intracellular domain (NICD), which subsequently travels to the nucleus to activate the transcription of specific target genes. Thus, Notch signaling induces differential gene expression programs in neighboring cells. Signaling events are normally transient, with rapid degradation of NICD limiting the duration of the response. Responses are determined by the cellular context of the signaling, with NICD-targeting specific effector genes to transduce tissue-specific biological responses.

The Notch pathway therefore represents a unique mechanism for short-range cellular communication between juxtaposed cells. Developmental studies, particularly in invertebrates, have shown that this short-range signaling can function in distinct ways to regulate varied and often-divergent responses through effects on cell specification, proliferation, apoptosis, differentiation, and tissue patterning. For example, Notch signaling is involved in the process of lateral inhibition in which subtle differences in Notch signaling between two equivalent progenitor cells are transcriptionally amplified such that a bias in productive Notch signaling occurs between each cell. This unequal priming of Notch signaling leads to the establishment of each cell as either signal-sending or signal-receiving to pattern the developing tissue. Notch signaling can also occur between two distinct cell populations to establish boundary/inductive cell fate interactions associated with tissue patterning. Moreover, Notch signaling can control binary cell fate decisions between two daughter cells that are dependent on asymmetrical inheritance of Notch regulatory components. Lastly, Notch signaling has been implicated in stem cell maintenance and self-renewal through cellular interactions between stem cells and juxtaposed niche cells. In many cases of complex tissue and organ formation, these different modes of Notch signaling are used iteratively and/or in a combinatorial manner to generate complicated differentiation programs and outcomes. Importantly, Notch signaling does not act alone in these events, and invariably the Notch pathway interacts or cross-talks with other key signaling pathways (Jak/STAT, RTK, TGFβ, Wnt, BMP, Hedgehog) to establish functional and complex signaling networks required for development and tissue homeostasis. Several excellent reviews on canonical Notch signaling have been recently published. Not surprisingly, Notch signaling has been shown to play a critical role in gastrointestinal tissues. This chapter summarizes the current understanding of canonical Notch signaling mechanisms and highlights the important role that Notch plays in the intestinal epithelium to regulate stem cell self-renewal, progenitor cell proliferation, and cell fate determination.
6.2
Regulation of Notch Pathway Activity
In mammals, multiple Notch receptor and ligand family members are widely expressed during development and in adult tissues ( Table 6.1 ). The complexity of Notch receptor and ligand interactions suggests that individual ligand/receptor combinations are likely to be involved in both distinct as well as overlapping functions. Another unique feature of Notch signaling is that ligand-mediated Notch receptor proteolysis generates an active NICD fragment that does not utilize downstream secondary messengers for signal amplification. More importantly, Notch proteolysis and the initiation of Notch signaling is an irreversible process in which each activated Notch receptor can only signal once through the generation of a single NICD fragment. Thus, the availability and presentation of Notch ligands to receptors must be highly regulated, not only to control productive signaling in a spatiotemporal manner but also to prevent inappropriate signaling and the potential for oncogenic transformation. Differential expression and transcriptional regulation of Notch receptors and ligands contribute to this regulation. However, cells that express the same levels of receptors and ligands are capable of committing to different Notch-dependent cell fates, indicating that Notch activity is under additional regulation. Indeed, studies in invertebrates and mammals have highlighted the importance of posttranslational modifications and trafficking of both receptors and ligands to modulate Notch activity. The relevance of structural domains within Notch receptors and ligands in relation to key aspects of proteolysis, glycosylation, intracellular trafficking, and ubiquitin-mediated degradation that regulate Notch activity are described below ( Fig. 6.1 and Table 6.1 ).
Component/Activity | Gene(s) a | Protein Type/Activity | Modulatory Function |
---|---|---|---|
Receptor | Notch1, Notch2, Notch3, and Notch4 | Type 1 transmembrane protein | |
Ligand | Dll1, Jag1, and Jag2 | DSL and DOS domain-containing | |
Dll3 and Dll4 | DSL only | ||
Proteolysis | Furin-like convertase | Proprotein convertase (receptor S1 cleavage) | Heterodimer formation |
Adam10 | Metalloproteinase (receptor S2 cleavage) | Initiates receptor signaling b | |
TspanC8s c | Metalloproteinase trafficking/substrate presentation (receptor S2 cleavage) | Positive and negative regulators | |
Psen(1 or 2), Ncstn, Psenen, Aph1(a, b or c) | γ-Secretase complex: 1:1:1:1 stoichiometry (receptor S3/4 cleavage) | NICD generation | |
Adam9, Adam10, Adam12, Adam17 | Metalloproteinase (ligand) | Ecto domain shedding | |
Glycosyltransferase | Pofut1 | O-Fucosyltransferase (receptor) | Essential for Notch activity |
Fringe Family: Lfng, Mfng, Rfng | β1,3-GlnNAc transferase (receptor) | Optimal Notch activity/ligand specificity | |
Poglut1 | O-Glucosyltransferase (receptor) | Essential for Notch activity Promotes extracellular cleavage | |
Gxylt1, Gxylt12, and Xxylt1 | O-Xylosyltransferase (receptor) | Negative regulator | |
Eogt1 | O-GlcNAc transferase (receptor) | Impaired Notch signaling Reduced DLL1/DLL4 binding | |
Membrane/endosomal trafficking | Mib1 | Ring finger E3 ubiquitin ligase (ligand endocytosis) | Optimal ligand activity |
Itch/Nedd4 family | HECT domain E3 ubiquitin ligase (receptor endocytosis) | Reduces Notch activity; lysosomal trafficking/degradation | |
Deltex1, 2, 3, and 4 | Ring finger E3 ubiquitin ligase (receptor endocytosis) | Optimal receptor activity | |
Numb | Receptor-binding partner (asymmetric partitioning during cell division) | Negative regulator | |
Fbxw7 | F-Box ubiquitin ligase (NICD degradation) | Reduces Notch activity; proteosomal trafficking/degradation | |
Crumbs | Receptor-binding partner (inhibits ligand-independent Notch endocytosis/activation) | Negative regulator | |
Commd99 | Regulator of endosomal trafficking and Notch recycling to the cell surface | Positive regulator | |
Ubiquitin-specific | Usp28 and Usp12 | Counteracts Fbxw7 |
a Mouse gene symbols are listed; see text for gene definitions and protein activity.
b Rate-limiting step for initiation of a Notch signaling event.
c Tspan5, 10 and 14 (positive regulators) and Tspan15 and 33 (negative regulators).
6.2.1
Notch Receptors and Ligands
Canonical Notch signaling involves activation of Notch receptors at the cell surface by ligands of the DSL (Delta/Serrate/LAG-2) family, which includes Delta and Serrate/Jagged in Drosophila and mammals and LAG-2 in Caenorhabditis elegans. In mammals, there are four different Notch receptors (Notch1, 2, 3, 4), three Delta-like proteins (Dl1, Dll3, and Dll4), and two Jagged proteins (Jag1 and Jag2). Both Notch receptors and their ligands are type 1 transmembrane proteins. All Notch receptors consist of a multidomain protein, including an extracellular domain with 29–36 tandem epidermal growth factor (EGF)-like repeats, a heterodimer domain, a negative regulatory region (NRR) composed of three cysteine-rich LNR (Lin12, Notch repeats) repeats, a transmembrane segment, and an intracellular domain that contains the NICD signaling segment. Several of the EGF-like repeats are critical for regulating the affinity of ligand binding and signaling efficiency. For example, EGF-like repeats 11–12 are required for productive ligand signaling between neighboring cells ( trans activation), whereas EGF-like repeats 24–29, which map to the Abruptex region of Drosphila Notch, inhibit functional ligand interactions within the same cell ( cis inhibition). Recent structural analysis suggests that the alignment of Ca 2 + -binding and non-Ca 2 + -binding EGF repeats can create a hinged conformation of the Notch extracellular domain. In addition, X-ray structures of Notch1-Dll4 and Notch1-Jag1 complexes have revealed that the module at the N terminus and DSL domains of Dll4 and Jag1 interact with Notch EGF repeats 11–12 in an antiparallel fashion. It is postulated that variations to the extent of interface interactions between different Notch receptor/ligand complexes as well as ligand-binding interactions with other Notch EGF repeats may contribute to differences in ligand affinity and productive Notch activation. In the absence of ligand, the NRR domain prevents inappropriate receptor activation by masking a critical proteolytic cleavage site that only becomes accessible after ligand-induced conformational changes (see text below). The intracellular segment of the Notch receptor contains a RBP-Jκ association module (RAM) motif, ankyrin repeats, nuclear localization signals, and a proline/glutamic acid/serine/threonine-rich (PEST) motif that regulates NICD protein stability.
Classic Notch ligands (Dll1, Jag1, and Jag2) contain a DSL domain, a Delta, and OSM-11-like (DOS) domain and multiple EGF-like motifs within their extracellular domains. Additionally, both Jag1 and Jag2 contain a cysteine-rich domain located in a juxtamembrane region. Both DSL and DOS domains are involved in productive ligand binding to Notch receptors. However, similar to Notch ligands found in C. elegans , the mammalian ligands (Dll3 and Dll4) lack the DOS motif. In addition, several Notch coligands and other noncanonical Notch ligands that lack both DSL and DOS domains have been identified that act through alternative and poorly defined mechanisms and will not be discussed further.
6.2.2
Proteolysis is Essential for Notch Receptor Maturation and Activation
During maturation in the secretory pathway, the Notch receptor undergoes a critical cleavage by furin-like protein convertases at site 1 (S1) to produce the extracellular N-terminal and intracellular C-terminal fragments (NTF and CTF) that form the heterodimeric receptor molecule, which is held together by noncovalent interactions ( Fig. 6.1 ). Upon ligand engagement at the cell surface, a series of receptor cleavage events in the CTF are initiated. The first proteolytic cleavage event at site 2 (S2) within the extracellular juxtamembrane region of the Notch CTF is performed by a disintegrin and metalloproteinase (Adam), which leads to removal of the Notch ectodomain and the generation of a membrane-anchored Notch CTF, termed Notch extracellular truncation (NEXT). This Adam-mediated cleavage event is the key regulatory step in the initiation of Notch signaling. Numerous studies in mice have demonstrated that Adam10 is the principal sheddase responsible for ligand-dependent Notch S2 cleavage in vivo . In addition, in vitro studies have confirmed that canonical ligand-induced proteolysis of human Notch receptors is strictly dependent upon Adam10.
The TspanC8s are members of an evolutionarily conserved tetraspanin family that interact with and promote Adam10 intracellular trafficking and enzymatic maturation. Consistent with a role of Adam10 in Notch activation, TspanC8s promote Notch activity in flies and worms. However, in mammals, different TspanC8s differentially regulate shedding of distinct Adam10 substrates, including Notch, in a manner that is likely dependent on Adam10 subcellular localization, conformation, and/or direct TspanC8 – substrate interactions.
NEXT is susceptible to subsequent sequential intramembrane proteolytic processing at sites S3 and S4 by the presenilin-dependent γ-secretase complex to release the NICD signaling domain. As part of a negative feedback regulatory mechanism, the interacting ligand (and possibly the NTF of the Notch receptor ectodomain) is trans-endocytosed into the signal-sending cell to reduce ligand availability; in addition, this may initiate other signaling events in the signal-sending cell. The γ-secretase complex is comprised of the membrane proteins presenilin (Psen), nicastrin (Ncstn), presenilin enhancer 2 homolog (Psenen), and anterior pharynx defective 1 homolog (Aph1) in a 1:1:1:1 stoichiometry. At least six possible γ-secretase complexes can be formed in rodents due to two isoforms of presenilin and three isoforms of Aph1. The biological significance of the different complex configurations is not currently known; however, biochemically, it appears that different complexes may exhibit differential processing efficiency for certain substrates and differential susceptibility to pharmacological inhibitors. In the case of ligand-dependent Notch signaling, both presenilin-1 and -2 can participate in Notch intramembrane processing leading to transcriptional activation. However, it will be important to identify the temporal and spatial expression patterns of each of the components of the γ-secretase complex in gastrointestinal tissues to determine, which complexes are formed because complex composition likely affects substrate specificity and biological function in vivo. Additionally, several membrane-anchored Notch ligands are susceptible to sequential processing by Adam and γ-secretases, but the physiological importance of these cleavage events with respect to regulation of Notch signaling remains unsolved. It is conceivable that Adam-mediated ectodomain shedding would deplete cell surface ligand levels and downregulate Notch signaling. Similarly, the production of soluble ligand could modulate and possibly interfere with productive interactions of membrane-bound ligands with cell surface Notch receptors. By contrast, intramembrane processing of Notch ligands by γ-secretases may generate intracellular fragments that have distinct signaling activities independent of Notch receptors. However, the biological significance of this alternative signaling pathway associated with Notch ligands awaits further investigation.
Following NEXT cleavage by the γ-secretase complex, the biologically active NICD is released from the membrane into the cytosol. NICD translocates to the nucleus of the Notch receptor-expressing cell and binds to the DNA-binding protein RBP-Jκ (recombination signal-binding protein for immunoglobulin kappa J region; also known as CSL), the transcriptional coactivator Mastermind (MAML) and other coactivators to initiate the transcription of target genes, including hairy and enhancer of split 1 (Hes1). In the absence of NICD, RBP-Jκ appears to occupy its DNA-binding sites some of the time and participate in a repressor complex.
The Notch signal is short lived due to rapid proteosomal degradation of NICD. The PEST motif in the C-terminus of NICD is rapidly phosphorylated by CDK8 kinase, ubiquitinated by E3 ligases such as F-box and WD-40 domain protein 7 (Fbxw7) and then sent for rapid protein degradation. Thus, study of the Notch signaling pathway is challenging because of the difficulty of detecting nuclear NICD due to its rapid degradation. As an alternative to NICD detection, assessment of a transcriptional target of Notch signaling such as Hes1 is frequently used as an indicator of Notch signaling activity; however, there are caveats to this method of detection as well because Hes1 can be regulated by other pathways, including the fibroblast growth factor pathway, GATA transcription factors, Ids, and Pax transcription factors. More recently, genetic mouse Notch reporter strains have been engineered to mark active Notch signaling at the level of sequential Notch cleavage and NICD production (e.g., NIP1-Cre) or transcriptionally using knockin CreER reporter alleles to Notch target genes, such as Hes1 and Olfm4.
6.2.3
Glycosylation
Notch and DSL ligands are extensively glycosylated, with many potential sites for both N-linked and O-linked glycosylation. Studies in flies and mammals have revealed that O-linked glycosylation modifications of the Notch extracellular domain can regulate Notch activity through a variety of potential mechanisms, including modulation of ligand specificity, increasing levels of functional cell surface Notch receptors through correct folding and trafficking, and by improving ligand-mediated Notch proteolysis. The endoplasmic reticulum (ER)-localized glycosyltransferase protein O-fucosyltransferase1 (Pofut1) is required for addition of O-fucose moieties to specific Ser or Thr residues in distinct EGF-like repeat motifs of the Notch receptor. Many of the O-fucose modified EGF repeats can be further extended by the Fringe family of glycosyltransferases (discussed in more detail below). In flies and mice, analysis of loss-of-function Pofut1 mutants has revealed that this glycosylation pathway regulates Notch signaling activity. Interestingly, earlier studies in flies suggested that Pofut1 had ER chaperone activity distinct from its enzyme activity, which was required for efficient folding and transport of Notch receptors to the cell surface and optimal signaling. Similarly, in mammalian cells, overexpression of a Pofut1 mutant that lacks enzyme activity partially restored Notch signaling, suggesting that O-fucose modifications are not essential for trafficking but are needed for efficient ligand-mediated signaling. Although other unidentified ER glucosidases may contribute to Notch folding and chaperone activity, the O-fucose moieties and their subsequent modifications by Fringe glycosyltransferases are critical for Notch to signal optimally.
In mammals, three Fringe proteins, Lunatic, Manic, and Radical Fringe, are responsible for the addition of N-acetylglucosamines to O-fucosylated EGF repeats of the Notch receptor. Specifically, Fringe-mediated modifications of the highly conserved EGF-like 12 repeat alter Notch responsiveness and the binding specificity to different Notch ligands. Although Delta-like and Jagged ligands have intrinsically different Fringe-independent affinities for Notch receptors, in general, Fringe-dependent modifications enhance responsiveness of Notch receptors to Delta-like ligands over Serrate/Jagged ligands. These differences in ligand-binding affinities associated with Fringe glycosylation have been implicated in regulating the strength and location of Notch signaling involved with tissue patterning and morphogenesis in a context-dependent manner. The importance of glycosylation for the strength of Notch signaling was clearly demonstrated when a Thr-to-Ala mutation of the conserved O-fucose site within the EGF-like 12 repeat of Notch1 was introduced, and the resultant homozygous mouse mutants (Notch 12f/12f ) were viable but had reduced growth rates. Moreover, the complex expression patterns of the three mammalian Fringe proteins combined with variations and often-opposing effects on ligand responsiveness in a cell-dependent manner suggest that these glycosyltransferases may have other undefined regulatory functions.
In Drosophila , another glucosyltransferase, Rumi, has been implicated in regulating Notch signaling through the addition of O-glucose to specific serine residues of the Notch extracellular domain. These O-glycosylation modifications are not required for cell surface expression or ligand binding, but are important for correct folding and ligand (Delta)-mediated conformational changes needed for initiating Notch proteolysis and activation. A mammalian homolog of Rumi, Poglut1 can rescue Notch-phenotypes in Rumi mutant flies. Conversely, Poglut1 deletion in mice produces embryonic lethality with many Notch-like developmental defects. However, other developmental defects distinct from Notch are found in Poglut1-deficient mice suggesting that O-glycosylation is involved in regulating multiple targets critical for mammalian development. Specific xylosyltransferases, Gxylt1, Gxylt2, and Xxylt1 can further elongate O-glucose glycans through addition of xyloses. In Drosophila, xylosyl extension of O-glucose glycans on Notch inhibits Notch activation possibly by inhibiting proper Notch trafficking to the cell surface. Other O-glycosylation modifications, including O-GlcNAc glycosylation, have been detected in mammalian Notch receptors and ligands. Also in mammals, the EGF-specific O-GlcNAc transferase Eogt1 is responsible for O-GlcNAc modifications of EGF repeats. While the Drosophila homolog Eogt has been reported to have genetic interactions with Notch, the recent analysis of Eogt1-deficient mice revealed that Notch receptors lacking only O-GlcNAc glycans have reduced ligand-induced Notch signaling due to decreased affinity for Dll1 and Dll4 but not Jag1. Further studies are clearly needed to better understand which EGF repeats on Notch receptors are modified by glycosylation and how these posttranslational modifications alter Notch signaling in vivo .
6.2.4
Endosomal/Membrane Trafficking
Notch ligands generate productive Notch signaling through binding cell surface Notch receptors on adjacent cells ( Fig. 6.1 ). However, Notch ligand activity is critically dependent on mono-ubiquitination and endocytic trafficking, suggesting that ubiquitinated/endocytosed Notch ligands undergo posttranslational modifications upon trafficking in endocytic vesicles in order to gain biological activity. In Drosophila , two structurally unrelated E3 ubiquitin ligases, Neuralized (Neurl) and Mindbomb (Mib), mediate mono-ubiquitination of Notch ligands, which is necessary for ligand endocytosis. In mammals, there are two different Mindbomb paralogs (Mib1 and Mib2) and two Neuralized paralogs (Neurl1 and Neurl2). Analysis of germline and conditional-knockout mice of each Mib and Neurl gene has revealed that only deletion of Mib1 produces widespread Notch loss-of-function phenotypes. In contrast, loss of Mib2 or the two Neurl paralogs in mice is well tolerated and do not produce such phenotypes, indicating that Mib1 has an essential, nonredundant role in Notch ligand ubiquitination and trafficking in mammals. Similarly, in humans, single-allele familial mutations of Mib1 are correlated with left ventricular noncompaction cardiomyopathy (LVNC) and aberrant Notch signaling. However, it should be noted that Notch is not the only target of Mib1 and it is likely that Mib1 can interact with additional protein substrates. Together, these results suggest that ubiquitination and/or endocytosis is required for ligand competency. Additionally, ligand endocytosis after receptor binding has been implicated in generating the molecular force needed to confer conformational changes within the Notch receptor ectodomain that enables Adam-mediated cleavage. However, it is currently unclear how these disparate activities associated with Notch ligand endocytosis and trafficking enhance Notch signaling activity.
Productive Notch signaling is also dependent on Notch receptor endocytosis, which controls the rate of Notch degradation and, therefore, regulates the levels of functional Notch receptors at the cell surface. Several E3 ligases, including the Itchy/Nedd4 (neural precursor expressed, developmentally downregulated 4) family, act as negative regulators of Notch signaling that target nonactivated Notch for lysosomal trafficking and degradation. In many instances, loss of these ubiquitin ligases results in Notch overactivation, presumably due to the accumulation of Notch receptor within endosomal compartments where it becomes susceptible to ligand-independent proteolytic activation and NICD release. By contrast, a different E3 ligase, Deltex, promotes Notch signaling by antagonizing the negative effects of the Itchy/Nedd4 family and enhancing Notch accumulation in an endosomal compartment that promotes receptor recycling and ligand-independent activation. Unlike the essential role of Adam10 in ligand-dependent Notch S2 cleavage, Adam17 and possibly other proteases are responsible for ligand-independent Notch S2 cleavage in these specific endosomal compartments. Intriguingly, both murine Notch1 and Notch2, and human Notch1, require Adam17 for ligand-independent activation, whereas human Notch2 is resistant to such processing. While Notch1 NRR activating mutations that result in ligand-independent proteolysis are found frequently in human leukemias, highlighting the importance of tight control of metalloprotease access to the S2 site, it is currently unclear whether such ligand-independent Notch activation plays a role in mammalian development and normal tissue homeostasis. Recently, several Notch interacting proteins have been identified such as Drosophila Crumbs and mammalian Commd9 that regulate Notch localization and endosomal trafficking that impact ligand-independent activation. Together, these studies suggest that the balance of effects generated by different E3 ligases and other binding partners impacts the fate, sorting and compartment-specific degradation of Notch that ultimately control the cell surface half-life of Notch, its accessibility to functional ligand interactions as well as its ability to be processed by γ-secretases.
Numb is another well-characterized Notch antagonist in which asymmetric partitioning and loss of Notch activity occur in one daughter cell during mitosis. The mechanism by which Numb inhibits Notch activity is less well understood. Interestingly, studies in mammals have demonstrated that a Golgi protein (ACBD3) associates with Numb upon Golgi fragmentation during cell division, providing a mechanism for coupling asymmetric Notch signaling to mitosis. A recent study reported that the tumor suppressor miR-34a and Numb synergize to regulate asymmetric division and suppress plasticity in colon cancer stem cells. Similar processes of asymmetric segregation of Notch activity have been associated with Notch ligands and the asymmetric segregation of Neuralized, which promotes ligand endocytosis and activity.
Other E3 ubiquitin ligases, including Fbxw7, can act as tumor suppressors and have been implicated in the degradation of several proto-oncogenes, including NICD. CDK8 kinase-dependent phosphorylation of the NICD PEST domain makes it available for Fbxw7 ubiquitination and subsequent proteosomal degradation. Intestine-specific deletion of Fbxw7 resulted in impaired goblet cell differentiation and accumulation of proliferating progenitor cells and this was associated with increased Notch activity and c-Jun expression. Loss of Fbxw7 also accelerated Apc-dependent tumorigenesis confirming its role as a tumor suppressor in the intestine. Recently, Behrens and colleagues have proposed that Fbxw7 not only reduces the stability of NICD but also that the Fbxw7 gene is also transcriptionally downregulated by the Notch effector Hes5, creating a potential intracellular positive feedback loop for Notch signaling.
Lastly, more recent studies have shown that deubiquitination of Notch by ubiquitin-specific proteases Usp28 and Usp12 regulate Notch trafficking and activity. In colorectal cancer, Usp28 has been shown to reduce FBW7-dependent ubiquitin-mediated proteasomal degradation of NICD, thereby increasing Notch activity. Conversely, in vitro studies have demonstrated that upon Itch-dependent polyubiquitination of inactive uncleaved Notch, Usp12 deubiquitinates Notch and promotes its lysosomal degradation. Whether additional ubiquitin ligases and ubiquitin-specific proteases can regulate other core components of Notch signaling remain to be determined.
6.2.5
Summary of Notch Signaling
Notch signaling is an evolutionarily conserved mechanism for establishing physiological differences in neighboring cells ( Fig. 6.1 ). As described above, the signal is short lived due to the proteolytic cleavage of the receptor upon ligand activation and the subsequent rapid destruction of the released NICD signaling fragment. After ligand-receptor interaction, the key rate-limiting step for the initiation of a Notch signaling event is Adam10-mediated S2 cleavage; however, as outlined above, other types of regulation can occur at many steps of the pathway that modulate the activity of both ligands and receptors. These regulatory mechanisms may affect either the amplitude or duration of a signaling event. Evidence suggests that pathway activity is regulated by restriction of ligand or receptor availability (both spatially and temporally), modulation of ligand and receptor trafficking, and posttranslational modification of receptor and ligand. In addition to protein regulation, microRNAs are also involved in controlling pathway component availability. For example, in intestinal stem cells (ISCs) of the Drosophila gut, miR-305 regulates Notch and insulin pathways required for adaptive homeostasis associated with nutritional status. Another example is the tumor suppressor miR34a that binds to the 3′ UTR mRNA sequences of Notch receptors, leading to reduced Notch protein levels and decreased downstream Notch signaling. In colon cancer stem cells, miR-34a controls a bimodal Notch signal that specifies the choice between self-renewal and differentiation. More recent work demonstrated that miR-34a acts together with Numb and Notch to form a feed forward loop that curbs excessive proliferation by enforcing a binary cell fate choice and suppressing cancer stem cell plasticity. This field of research is still relatively nascent, and it is likely that mammalian Notch activity is differentially regulated based on temporal, tissue-specific, and cell-specific contexts.
6.3
Overview of Intestinal Structure and Development
The intestine is composed of cells originating from each of the three germ layers: the epithelium from endoderm, the mesenchyme (muscle, myofibroblasts, etc.) from mesoderm, and the enteric nervous system from ectoderm. The homeobox transcription factor caudal type homeobox 2 (Cdx2) appears to be a master regulator of intestinal identity, and its expression is essential for specification of the intestinal epithelium from the primordial gut endoderm, and for establishing normal epithelial-mesenchymal interactions. After morphogenesis of the intestine, stem and progenitor cells continuously divide and differentiate to maintain the epithelium throughout the lifespan of the organism. At least six distinct epithelial cell types are formed. This includes absorptive enterocytes and three secretory (granulocytic) cell types: mucus-producing goblet cells, antimicrobial peptide-producing Paneth cells, and hormone-releasing endocrine cells. Less frequent intestinal cell types include tuft cells, also called brush cells, which are chemosensory cells that orchestrate intestinal responses to parasite infection (also see Chapter 32 ), and microfold, or M, cells, which transport luminal antigens across the epithelium to mucosa-associated immune cells. The general structures of developing and adult intestine, including epithelial and mesenchymal components, are shown in Fig. 6.2 .

A complex network of signaling pathways and transcription factors work in concert to maintain homeostasis by regulating proliferation and cellular differentiation. Many studies have demonstrated the central importance of Notch signaling for homeostatic control of the intestinal epithelium, regulating both progenitor cell proliferation and cell fate determination. In addition, other fundamental signaling pathways are critical for intestinal development and homeostasis, including Wnt, Hedgehog, and bone morphogenetic protein (BMP). The specific roles of each of these pathways have been established primarily from analysis of genetically engineered mouse models. Before detailing the important functions of Notch signaling in the intestine, these other fundamental signaling pathways will be briefly summarized because they are somewhat interdependent and regulate overlapping functions.
6.3.1
Developmental Signaling Pathways
6.3.1.1
Hedgehog Signaling Restricts the Proliferative Zone
In the intestine, Hedgehog signaling is exclusively paracrine. The pathway ligands Sonic Hedgehog (Shh) and Indian Hedgehog (Ihh) are secreted from epithelial cells and activate downstream signaling through their receptors Patched 1 and 2 and effectors Gli1, 2, and 3 in the mesenchyme. Gli2 appears to be the main effector for Hedgehog signaling in the developing intestine. At embryonic day 8.5 of mouse development (E8.5), Ihh and Shh are expressed in the gut endoderm in overlapping patterns, and by late fetal development, this expression pattern is restricted toward the proliferating epithelium of the intervillus zone. Mesenchymal cell clusters that form just beneath the epithelium at these zones are Hedgehog ligand responsive and drive villus formation starting at E14.5. Mice deficient in Shh or Ihh die perinatally and exhibit many gastrointestinal defects, including changes in enteric nervous system development, loss of smooth muscle, and altered epithelial proliferation. Similar phenotypes were observed in other studies that examined the consequences of blocking all Hedgehog signaling in the intestine by treating neonatal mice with a Hedgehog neutralizing antibody or a pharmacological inhibitor, or by using a genetic mouse model that expressed a secreted form of the pan-Hedgehog inhibitor Hhip from the intestinal epithelium via the villin promoter (Villin-Hhip mice). Phenotypes include increased epithelial proliferation and formation of ectopic crypt-like structures, impaired cluster formation and villus emergence, and reduced smooth muscle. Hedgehog signaling is also important for maintaining intestinal homeostasis in the adult. Mice with Cre-activated Hhip expression (VFHhip mice), or a conditional deletion of Ihh (Cyp1a1-CreIhh fl/fl ) in the intestinal epithelium showed expansion of the proliferative zone and progressive loss of smooth muscle.
In addition to its role in restricting proliferation and maintaining stromal differentiation, Hedgehog signaling has also been implicated in the inhibition of ISC self-renewal and differentiation, likely through suppressing Wnt signaling. Thus, the combined effect of Hedgehog signaling is important for villus formation, smooth muscle proliferation and differentiation, and restriction of the epithelial proliferative compartment in perinatal and adult intestine. Furthermore, since Hedgehog signaling mutants displayed epithelial phenotypes, a feedback mechanism that can signal from the mesenchyme back to the epithelium in response to Hedgehog signaling must exist.
6.3.1.2
BMP Signaling Restricts Crypt Number
Active BMP signaling is transduced through the cytoplasm to the nucleus by the SMAD transcription factors following BMP ligand-mediated receptor activation. BMP signaling in the intestine is bidirectional with multiple BMP ligands, receptors, SMAD transcription factors, and BMP inhibitors being expressed in both the epithelium and mesenchyme. Mesenchymal cells at intervillus and intercryptal regions have been shown to secrete BMP2 and BMP4 ligands. In the epithelium, it appears that the most active BMP signaling, as determined by the presence of nuclear phospho-SMAD1, 5, and 8 transcription factors, occurs in the villus rather than the crypts. This is likely due to inhibition of BMP signaling in the crypt region resulting from enriched expression of BMP inhibitors, including Noggin and Gremlin 1, in subcryptal mesenchymal cells. The pattern of BMP ligand and inhibitor expression leads to the formation of an increasing BMP activity gradient along the crypt-villus axis.
Phenotypic differences observed from modulating BMP signaling exclusively in the epithelium or mesenchyme suggests the pathway may play different roles in these two cellular compartments. A transgenic mouse in which the villin promoter was used to drive expression of Xenopus noggin in the intestinal epithelium (X-noggin), presented with ectopic crypt structures in 3-month-old mice and adenomatous foci development in older mice, suggesting that BMP signaling normally functions to limit crypt number. These types of epithelial changes are reminiscent of a rare, autosomal-dominant gastrointestinal syndrome called familial juvenile polyposis (FJP), which is characterized by development of hamartomatous polyps throughout the gut. Accordingly, mutations in SMAD4 and Bmpr1a have been identified in FJP patients, consistent with BMP signaling alterations in the pathogenesis of this syndrome. Indeed, a second mouse model in which the Bmpr1a receptor was conditionally deleted using the interferon-inducible Mx1-Cre also exhibited polyp formation. In contrast, loss of Bmpr1a in the epithelium only showed increased proliferation and a defect in secretory cell differentiation, but not formation of ectopic crypts or polyps, suggesting that epithelial BMP signaling is not sufficient for driving FJP. In agreement, depleting BMP signaling exclusively in pericryptal fibroblasts resulted in increased mesenchymal proliferation, development of a reactive stroma, and increased polyposis formation at 1 year. These reports suggest that it is mesenchymal BMP signaling that is primarily responsible for suppressing epithelial hyperproliferation.
It is thought that epithelial BMP signaling limits epithelial stem cell proliferation via restriction of ISC self-renewal. There is some evidence that BMP represses ISC self-renewal via Wnt pathway suppression ; however, this is uncertain as some studies showed no change in Wnt signaling after repression of epithelial BMP signaling, or observed that BMP regulation was Wnt-independent.
It is likely that BMP signaling is a key factor mediating Hedgehog effects on epithelial proliferation and villus morphogenesis. BMP4 and BMP7 are positively regulated by Hedgehog signaling, and the ectopic crypt phenotypes in the Hedgehog loss-of-function Villin-Hhip mouse and the BMP loss-of-function X-noggin mouse are similar. During development, Hedgehog-responsive mesenchymal clusters express BMP ligands and signal modifiers. Manipulating BMP signaling via BMP antagonist or exogenous BMP ligand administration, and conditional Bmpr1a deletion in Hedgehog-responsive mesenchyme, resulted in altered cluster formation, and diminished villus size and emergence. Together these studies demonstrate an important role for BMP in regulating intestinal development and ISC homeostasis.
6.3.1.3
Wnt Signaling Promotes Proliferation
In opposition to the antiproliferative effects of the Hedgehog and BMP signaling pathways, canonical Wnt signaling is a key pathway promoting proliferation in the intestinal crypts. Overactive Wnt signaling, such as that seen in the Apc min mouse model, leads to enlarged crypts with enhanced proliferation and progression to adenocarcinoma. Conversely, blocking Wnt signaling by forced expression of the secreted Wnt inhibitor Dickopff (Dkk1), or inactivation of the critical Wnt pathway components T-cell-specific transcription factor 4 (Tcf4), the signaling effector β-catenin, the Tcf4 target gene Myc , or deletion of R-spondin/Lgr complex components, leads to decreased proliferation and crypt loss. There is also evidence that noncanonical Wnt signaling through Wnt5a is important for aspects of gastrointestinal development, regeneration, and inflammation ; however, canonical Wnt signaling through β-catenin and Tcf4 appears to be most important for maintaining survival and promoting proliferation of ISCs. Accordingly, downstream targets of Tcf4-mediated Wnt signaling include proproliferation genes such as c-Myc and Cyclin D1.
Numerous Wnt ligands, receptors, and coreceptors are expressed in the intestine, with ligands produced in different epithelial cell populations as well as in pericryptal mesenchymal cells. The key cellular source and identification of specific Wnts functioning as ISC niche factors have been an area of active investigation. Global ablation of Wnt ligand secretion by pharmacologic inhibition of Porcupine (Porcn) led to reduced ISC numbers and blocked proliferation and regeneration. Surprisingly, ablation of Wnt ligand secretion in the epithelium via genetic deletion of Porcn did not disrupt intestinal homeostasis and regeneration, demonstrating that epithelial cells such as Paneth cells are not the key niche cell source of Wnts. Moreover, Porcn deletion in Myh11-positive subepithelial myofibroblasts did not affect intestinal homeostasis and regeneration. However, pericryptal Myh11-negative Foxl1-positive stromal cells have been shown to express Wnt family genes and are suggested to be a critical source of Wnt signaling by their secretion of Wnt activity potentiators, including Wnt 2b, Wnt5a, R-spondin 3, and the BMP inhibitors gremlin 1 and 2. Importantly, unlike Paneth cells, these mesenchymal cells were shown to be essential to support ISC proliferation. In addition to regulation of stem/progenitor cell proliferation, Wnt plays a role in cell fate determination in the intestine, with Wnt target genes Sox9 and EphB2/EphB3 mediating the differentiation of Paneth cells as well as their retention in the crypt base. From these studies, a simplified model of epithelial cell maintenance in the intestine includes stimulation of stem/progenitor cell proliferation by high Wnt in the crypt base, with cell cycle exit and terminal differentiation as cells move out of the crypt and the Wnt signaling zone.
6.4
Notch Signaling in Intestine
All of the Notch ligands (Dll1, Dll3, Dll4, Jag1, and Jag2) and receptors (Notch1, 2, 3, 4) are expressed in the mouse gut during early development (E13.5) through adulthood with the exception of Dll3, which recedes after early development. Based on mRNA expression patterns, the Notch ligands Dll1, Dll4, and/or Jag1 and receptors Notch1 and Notch2 were thought to be the most likely mediators of epithelial Notch signaling in the adult intestine. It has not been fully determined which intestinal cell populations express specific ligands and receptors. It is likely that different cellular targets are involved with distinct aspects of Notch regulation, including stem cell maintenance, progenitor cell proliferation, cell fate specification, and possibly cell maturation. Identification of signal-receiving cells by immunostaining for NICD or Hes1 shows positive labeling in several epithelial cells in the crypts, suggesting that Notch signaling is primarily active in stem and progenitor cells. However, other than crypt base columnar (CBC) stem cells, the cellular identity of NICD- and Hes1-expressing cells have not yet been definitively established. Although Hes1 mRNA appears to be predominantly localized to the crypts, two reports have also shown nuclear Hes1 protein expression in villus enterocytes of the developing intestine. Finally, NICD protein was also observed in scattered goblet cells in one report. Thus, Notch signaling may also be active in some mature cells in the villus. Although the focus of this chapter is on epithelial Notch signaling, it is worthwhile to note that Notch signaling components are also expressed in the intestinal mesenchyme, where they are important for the development of the enteric nervous, vasculature, and lymphatic systems, and likely play a role in inflammatory cell function in the gut.
6.4.1
Intestinal Phenotypes of Notch Mutants
Experimental disruption or activation of Notch signaling has a profound effect on the intestinal epithelium, resulting in changes in proliferation, cellular differentiation, and cell fate specification ( Table 6.2 ). Collectively, these studies have demonstrated that Notch signaling is essential for intestinal epithelial cell proliferation, with a pronounced reduction in dividing cells observed upon Notch inhibition. Conversely, experimental models with constitutive activation of Notch signaling exhibit increased proliferation, although, compared to Notch inhibition, fewer studies have explored the effects of Notch activation. In addition to its important role in maintaining progenitor cell proliferation, Notch appears to be the key pathway regulating a binary cell fate decision directing epithelial cell differentiation, with Notch signaling inhibiting secretory cell fate through regulation of the critical transcriptional factors Hes1 (and perhaps other Hes family members) and Atoh1 (discussed below) to allow absorptive enterocyte differentiation. In most instances, complete disruption of Notch signaling in the intestine results in morbidity due to the extreme cellular remodeling that affects intestinal function, including barrier function. Thus, many of the mutant mice with Notch pathway alterations have been studied at perinatal stages. Analysis in adults is limited to short time periods due to the rapidity of cellular remodeling in the intestine, with significant morbidity, including death, commonly observed with Notch pathway inhibition. In some instances, adult viability can be maintained through the use of mosaic Cre recombinase drivers to effect genetic changes in only a fraction of the crypts.
Component | Gene/Complex | Age a | Animal Model b | Intestinal Phenotype | Ref. | ||
---|---|---|---|---|---|---|---|
Proliferation | Apoptosis | Cell Specification | |||||
Receptor | Notch1 | 4 wks | LOF; Vil-CreERT2 x floxed Notch1; 12 days after Cre induction | Normal | Normal | ||
Notch1 | 2–4 mo | LOF; Vil-CreERT2 x floxed Notch1; 6–60 days after Cre induction | Normal | Moderate yet transient secretory cell hyperplasia; stem cell loss | |||
Notch1 | 8 wks | LOF; Vil-Cre x floxed Notch1 | Moderate goblet cell hyperplasia; increased number and clustering of M cells | ||||
Notch1 | 12 wks | LOF; Notch1 inhibiting antibody; 12 days after first injection | Moderate goblet cell hyperplasia | ||||
Notch2 | 4 wks | LOF; Vil-CreERT2 x floxed Notch2; 6 or 12 days after Cre induction | Normal | Normal | |||
Notch2 | 12 wks | LOF; Notch2 inhibiting antibody; 12 days after first injection | Normal | Normal | |||
Notch1 and Notch2 | 4-8 wks | LOF; Vil-CreERT2 x floxed Notch1/2; 6 or 12 days after Cre induction | Decreased | Secretory cell hyperplasia; stem cell loss | |||
Notch1 and Notch2 | 12 wks | LOF; Inhibiting antibodies specific for Notch1 and Notch2; 12 days after first injection | Decreased | ↓ Hes1, ↑ Atoh1; secretory cell hyperplasia; stem cell loss | |||
Ligand | Dll1 | 4 wks | LOF; Vil-CreERT2 x floxed Dll1; 14 days after Cre induction | Normal | Moderate goblet cell hyperplasia | ||
Dll1 | Adult | LOF; Ah-Cre x floxed Dll1; 5–28 days after induction | ↑ Atoh1, moderate secretory cell hyperplasia | ||||
Dll4 | 4 wks | LOF; Vil-CreERT2 x floxed Dll4; 14 days after Cre induction | Normal | Normal | |||
Jag1 | 4 wks | LOF; Vil-CreERT2 x floxed Jag1; 14 days after Cre induction | Normal | Normal | |||
Dll1 and Dll4 | 4 wks | LOF; Vil-CreERT2 x floxed Dll1/Dll4; 3–5 days after Cre induction | Decreased | ↓ Hes1; goblet cell hyperplasia; stem cell loss | |||
Nuclear effector | RBP-Jκ | 36 wks | LOF; P450-Cre x floxed RBP-J; 4–5 days after Cre induction | Decreased | Normal | ↓ Hes1, ↑ Atoh1; goblet cell hyperplasia but no change in other secretory cell types | |
RBP-Jκ | 4-5 wks | LOF; Vil-CreERT2 x floxed RBP-J; 6 and 12 days after Cre induction | Decreased | ↓ Hes1, ↑ Atoh1; secretory cell hyperplasia; stem cell loss | |||
MAML | P0 | LOF; 9kbVil-Cre x floxed “STOP” dnMAML | Decreased | Secretory cell hyperplasia | |||
NICD | P0 | GOF; 9kbVil-Cre x Rosa-floxed “STOP” NICD | Increased | Increased | ↑ Hes1, ↓ Atoh1; decreased goblet and endocrine cells | ||
NICD | Adult | GOF; Fabpl-Cre x Rosa-floxed “STOP” NICD x Z/AP reporter mouse | Increased | Normal | Decreased goblet and endocrine cells | ||
Receptor proteolysis | Adam10 | P0, Adult | LOF; 9kbVil-Cre (or Vil-CreERT2) x floxed ADAM10 | Decreased | ↑ Atoh1; secretory cell hyperplasia; stem cell loss | ||
Adam17 | P0, Adult | LOF; 9kbVil-Cre x floxed ADAM17 | Normal | Normal | |||
Adam17 | Adult | LOF; hypomorphic allele | Normal when unchallenged | Normal when unchallenged | |||
γ-Secretase | 7–8 wks | LOF; pharmacological inhibitors (DBZ, BZ); 1–5 day treatment in rats | ↓ Hes1, ↑ Atoh1; increased goblet and endocrine cells | ||||
γ-Secretase | 6 wks | LOF; pharmacological inhibitor (LY-411,575); 5 or 15 day treatment in TgCRND8 mice | Goblet cell hyperplasia | ||||
γ-Secretase | Adult | LOF; pharmacological inhibitor (DBZ); 5 day treatment in mice | Decreased | Stem cell apoptosis | ↑ Atoh1; secretory cell hyperplasia; stem cell loss | ||
Glycosyltransferase modifier | Pofut1 | 4 wks, 36 wks | LOF; 12.4 kbVil-Cre x floxed Pofut-1 | Decreased with displacement towards top of crypt | ↓ Hes1 and Hes5, ↑ Atoh1; secretory cell hyperplasia (restricted to crypts) | ||
Membrane and endosomal trafficking modifier | Mib1 | 2–4 wks | LOF; 12.4kbVil-Cre x floxed Mib1 | Decreased | Increased secretory cells, mislocated Paneth cells on villi, only 20% of mutants are viable past 4 weeks of age | ||
Fbxw7 | Adult | GOF; 9kbVil-Cre x floxed Fbxw7 | Increased | Increased | ↑ NICD1, ↑ Hes1, ↑ Hes5, ↓ Atoh1; decreased secretory cells | ||
Canonical bHLH effector | Hes1 | E14-E19 | LOF; Hes1 knockout | Normal | Increased in intervillus zone | ↑ Hes5, ↑ Atoh1, increased goblet and endocrine cells | |
Hes1 | P0 | LOF; Hes1 knockout | Altered distribution along the crypt-villus axis | Precocious differentiation of Paneth cells | |||
Hes1 | P2.5 | LOF; Vil-Cre x floxed Hes1 | Normal | Increased | Moderate goblet and endocrine cell hyperplasia; | ||
Hes1 | Adult | LOF; Vil-Cre x floxed Hes1 | Normal | Normal | Normal | ||
Hes1, Hes3, Hes5 | P2.5, 2 mo, 1 yr | LOF; Vil-Cre x Hes1 f/- , Hes3 −/− and Hes5 −/− | Decreased | Increased | Secretory cell hyperplasia; Paneth cells mislocalized; expanded colonic crypts | ||
Hes5 | Adult | LOF; Hes5 knockout | ↑ Dll1, ↑ Fbxw7, moderate goblet cell hyperplasia |
6.4.1.1
Mutations in Essential Notch Signaling Components
Complete disruption of Notch signaling in the mouse intestinal epithelium by conditional knockout of the essential DNA-binding protein RBP-Jκ resulted in loss of proliferating cells and increased goblet cell number. Increased goblet cell number was also observed with intestine-specific activation of a dominant-negative form of the Notch transcriptional coactivator MAML. Conversely, constitutive activation of the Notch pathway using NICD transgenic mice resulted in expansion of the proliferative zone and loss of secretory cells. Together, these mouse models demonstrated that Notch signaling normally acts to promote proliferation and inhibit secretory cell differentiation in the intestinal epithelium ( Table 6.2 ). Moreover, Notch signaling has been determined to be essential for maintenance of active stem cells, with rapid stem cell loss observed after global Notch inhibition with γ-secretase inhibitor treatment.
The Notch receptors Notch1 and Notch2 are both expressed in the crypt epithelium and were confirmed to be the key signaling receptors using mice genetically engineered with dual receptor deletion in the intestinal epithelium. Deletion of both receptors induced a phenotype similar to the intestine-specific RBP-Jκ-knockout mouse, suggesting that these receptors function redundantly, and together likely transmit all of the epithelial Notch signals. Similarly, a study that used neutralizing antibodies specific for Notch1 or Notch2 receptors showed that simultaneous treatment with both receptor antibodies induced intestinal epithelial cell phenotypes similar to the double receptor-deficient genetic model and to global Notch inhibition with γ-secretase inhibitor. Individual Notch1 receptor deletion or blockade showed moderate goblet cell hyperplasia, but normal goblet cell numbers with Notch2 loss or blockade, suggesting that Notch1 is predominant for cell fate determination. Lineage tracing studies confirmed active Notch1 receptor signaling in CBC stem cells, and Notch1 receptor deletion resulted in reduced stem cell number and decreased expression of the stem cell marker Olfm4. Thus, Notch1 appears to be the primary receptor regulating ISC function, and Notch1 and Notch2 receptors together regulate epithelial cell proliferation, cell fate determination, and postinjury regeneration.
In situ hybridization studies demonstrated that the Notch ligands Dll1, Dll4, and Jag1 are expressed in the crypt epithelium of the mouse intestine. In vivo function was assessed in mice with inducible, intestinal epithelial-specific deletion of Dll1, Dll4, and/or Jag1. Deletion of Jag1 or Dll4 had no apparent effect, while deletion of Dll1 resulted in a moderate increase in goblet cells without affecting progenitor cell proliferation. Combined deletion of Dll1 and Dll4 showed marked goblet cell hyperplasia with reduced cell proliferation, and loss of stem cells, a phenotype consistent with complete Notch loss-of-function. These data demonstrate that Dll1 and Dll4 are the key ligands regulating intestinal epithelial cell homeostasis. Interestingly, gene expression profiling studies showed that Paneth cells contain Dll1 and Dll4 transcripts, suggesting a key signaling relationship between Paneth cells and stem cells, with Paneth cells presenting Notch ligands Dll1 and Dll4 to adjacent stem cells expressing Notch1 receptor. However, analysis of genetic mouse models with Paneth cell ablation showed that these cells are not required for stem cell maintenance, suggesting alternate or additional cellular sources of Notch ligand are possible. Indeed, Dll1 is also expressed in secretory progenitor cells, which could be positioned next to stem cells when Paneth cells are lost.
The Adam proteases perform the first essential cleavage of the Notch receptor to activate signaling ( Fig. 6.1 ). Both Adam10 and Adam17 have been shown to cleave Notch receptors in vitro ; however, studies with genetic mouse models have determined that Adam10 is the key protease performing this function in vivo. Mice with deletion of Adam10 in the intestinal epithelium exhibited a Notch inhibition phenotype, with loss of proliferation and increased secretory cell differentiation. In contrast, mice with intestine-specific deletion of Adam17 had apparently normal intestines. Another mouse model with hypomorphic Adam17 expression also appeared normal when unchallenged; however, these mice exhibited increased sensitivity to inflammation upon treatment with dextran sulfate sodium. The authors attributed this phenotype to reduced shedding of EGF family ligands, many of which are Adam17 substrates, and the subsequent reduction in downstream signaling pathways important for intestinal repair.
The γ-secretase complex has the ability to cleave more than 60 types of transmembrane proteins, including the Notch receptor, Notch ligands Dll1 and Jag2, ErbB4, CD44, and E-cadherin. There does not appear to be a specific consensus sequence to determine whether a protein is cleaved by γ-secretase; instead, the main prerequisite for a potential substrate appears to be prior removal of the ectodomain by sheddases, such as removal of the Notch receptor ectodomain by Adam10. Rodents treated with the γ-secretase inhibitor benzodiazepine, dibenzazepine, or LY-411575 exhibited intestinal goblet cell hyperplasia and epithelial degeneration reminiscent of the phenotype observed in Notch disruption models. The similarity of the phenotypes induced by γ-secretase treatment and by complete Notch disruption through genetic models demonstrates that Notch is the dominant γ-secretase substrate in the intestine.
The Hes genes are classic transcriptional targets of canonical Notch signaling. The Hes proteins are basic helix-loop-helix (bHLH) transcription factors that primarily function as transcriptional repressors. Multiple Hes genes are expressed in the intestine, including Hes1, Hes3, Hes5, Hes6, and Hes7. Hes1-deficient embryos exhibited intestinal phenotypes similar to complete Notch disruption models; however, the phenotype of Hes1-deficient mice was not as severe as complete Notch loss-of-function mutants, and no effect on proliferation was observed. A more complete loss-of-function phenotype was observed after combined inactivation of Hes1, Hes3, and Hes5, with reduced cell proliferation and increased secretory cell formation, suggesting that these three Notch effectors cooperatively regulate intestinal homeostasis. A separate study of Hes1-deficient embryos reported precocious differentiation of Paneth cells and increased expression of Paneth cell genes. Therefore, Notch signaling through Hes1 may also play an important regulatory role in cellular maturation as well as differentiation.
6.4.1.2
Mutations in Notch Signaling Modifiers
Posttranslational regulation of receptors and ligands is a key mechanism used for titrating the level of Notch signaling ( Fig. 6.1 and Table 6.1 ). Mutations in these modifying proteins can result in phenotypes similar, although usually milder, to those found in core Notch pathway mutant models ( Table 6.2 ). For example, a mouse mutant with deficiency in the O-fucosyltransferase Pofut1 exhibited increased secretory cell number and decreased proliferation consistent with disrupted Notch activity, although the phenotype was less severe than total Notch loss-of-function mutants, and mice survived through adulthood albeit with smaller size.
Cell trafficking and ubiquitination are also important for regulating the levels of Notch receptors and ligands available at the cell surface. The E3 ubiquitin ligases Mib1 and Neurl have been shown to be important modifiers of Notch signaling ( Fig. 6.1 and Table 6.1 ), but so far only Mib1 has been reported to affect Notch signaling in the mouse intestine. Since Mib1 normally functions to promote efficient Notch signaling, loss-of-function mutation in both zebrafish and mouse intestine resulted in increased intestinal secretory cell number, consistent with reduced Notch function. As seen in the Pofut1 mouse mutants, the secretory cell transformation in Mib1 mutant mice was milder than complete Notch loss-of-function models, suggesting that some Notch signaling still occurs in the absence of this activity in mice. Interestingly, in the Mib1-deficient mice, Paneth cells were occasionally mislocated to the villus, a phenotype reminiscent of mice with deficiencies in ephrin signaling.
To limit the duration of Notch signaling, the NICD signaling molecule is transient with rapid ubiquitination and proteosomal degradation. An intestinal phenotype has been reported in mice deficient for the ubiquitin ligase Fbxw7 (also commonly called Fbw7), which has been implicated in the ubiquitination and subsequent degradation of NICD in addition to other proto-oncogenes (e.g., c-Jun, c-Myc). Fbxw7 messenger RNA is enriched in the intestinal crypts, suggesting that it may predominantly function in progenitor cells. Intestine-specific deletion of the gene for this ubiquitin ligase in the mouse using Villin-Cre resulted in Notch gain-of-function activity, including increased numbers of proliferating cells and alterations in secretory cell differentiation. Increased Notch signaling was observed together with decreased goblet and Paneth cell numbers. One study observed a paradoxical increase in the number of Neurogenin 3 (Neurog3)-expressing cells and in the expression of early endocrine transcription factors; however, the number of mature chromogranin-expressing cells was unchanged, suggesting a block in terminal differentiation of this lineage. However, another study showed reduced levels of Neurog3 in mice with intestinal epithelial deletion of Fbxw7, which would be consistent with a Notch gain-of-function phenotype. The basis for the discrepancy between these two studies is unclear. Mice deficient for both Fbxw7 and c-Jun showed that deletion of c-Jun restored proliferation to normal levels, but did not affect the alteration in secretory cell differentiation. These findings suggest that Fbxw7-mediated regulation of Notch signaling is more important in a later progenitor cell population than in a multipotential progenitor or stem cell. Further studies suggested that the Notch effector Hes5 directly represses Fbxw7 transcription, which may play a role in lateral inhibition mechanisms directing cell fate specification by prolonging NICD half-life in Notch signaling cells.
6.4.2
The Role of Atoh1 in Intestinal Cell Differentiation and Adaptive Response
6.4.2.1
Atoh1 Promotes Secretory Cell Differentiation
It is thought that Notch signaling regulates epithelial cell differentiation in the intestine by affecting the expression of the transcriptional effectors Hes1 (and perhaps other Hes family members) and Atoh1 ( Fig. 6.3 ). Notch signaling is known to repress Atoh1 transcription via Hes1. Accordingly, models of Notch disruption generally exhibit increased Atoh1 expression ( Table 6.2 ).

Atoh1 is a bHLH transcription factor involved in cell fate determination of intestinal secretory cells, as well as several neuronal cell types important for the proprioceptive system, including spinal cord interneurons, inner ear hair cells, and subsets of cerebellar and brainstem neurons. Currently, it is not well understood how Atoh1 transcription is activated in the intestinal epithelium, but once activated, it binds to its own 3′ enhancer to perpetuate its expression. One study reported that Cdx2 is a direct transcriptional activator of Atoh1. Studies in the neuronal system have indicated that Wnt signaling may be important for increasing Atoh1 expression. Overexpression of β-catenin in neural progenitor cells increased the activity of a GFP reporter under the control of an Atoh1 enhancer, and hyperactive Wnt signaling in lung progenitors activated Atoh1 and the development of intestinal secretory cell marker expression. Furthermore, increased Atoh1 expression after Notch inhibition was shown to depend on the formation of a DNA-binding complex that contains the Wnt pathway coactivators β-catenin and Tcf/Lef.
Atoh1-expressing cells are found in the epithelium of the progenitor cell zones and on the villi of both embryonic and adult intestine ( Fig. 6.3 ). Use of an Atoh1-lacZ reporter mouse demonstrated that intestinal expression was first observed at E15.5, near the time of initial epithelial cytodifferentiation. Some Atoh1-expressing cells in the crypt coexpressed the proliferation marker Ki67, suggesting that secretory cell fate specification occurs early in the differentiation process, prior to cells becoming postmitotic. Atoh1 also continues to be expressed in all mature secretory cells ( Fig. 6.3 ). Analysis of Dll1 expression in the intestine by in situ hybridization or with a Dll1-lacZ or a Dll1-GFP reporter mouse showed that Dll1 is expressed in a very similar pattern to Atoh1, suggesting that mature secretory cells and progenitor cells specifying to the secretory lineage express Notch ligand. Notch ligand expression in secretory progenitors may be important for the cellular patterning observed on the villus, perhaps involving lateral inhibition to control the pattern of secretory cell types surrounded by absorptive enterocytes.
In accordance with a possible role in the activation of Atoh1 expression, Wnt signaling can affect secretory cell differentiation and may be involved in making intestinal progenitor cells competent for secretory cell fate. Tcf4-null mice and transgenic mice expressing the Wnt inhibitor Dickkopf in the intestinal epithelium (Villin-Dkk1), both of which exhibit loss of Wnt signaling, showed reduced numbers of goblet and endocrine secretory cell types. Furthermore, Atoh1 expression was lost in the Villin-Dkk1 mice, and the Notch ligands Dll1 and Jag1 have been reported to be downstream targets of Wnt signaling. Thus, Wnt signaling appears to have a strong interaction with the Notch signaling pathway and could potentially influence secretory cell differentiation through Notch-dependent mechanisms. Interestingly, Notch blockade via administration of inhibitory antibodies to Notch1 and Notch2 receptors led to an upregulation of Wnt signaling, and a secretory cell hyperplasia that could be rescued by treatment with Wnt blocking antibodies. Further studies are needed to more completely understand how Wnt and Notch signaling interact in the intestine.
Many studies point toward Notch pathway regulation of Atoh1 as the key mechanism by which Notch signaling affects cell fate determination. Atoh1 is required for intestinal secretory cells in developing and adult intestine. Atoh1-null mice lacked all intestinal secretory cell types, including endocrine, goblet, and Paneth cells, and died at birth, presumably from a respiratory defect resulting from loss of certain neuronal populations. A second study that utilized a Cre-lox approach to specifically ablate Atoh1 expression in the adult intestinal epithelium (Atoh1 Δintestine ) using a mosaic Cre transgenic was observed to generate mice that lived to adulthood. Loss of secretory cells was observed specifically in the recombined Atoh1 Δintestine crypts, whereas nonrecombined crypts retained a normal secretory cell number, demonstrating that Atoh1 has a similar role in both developing and adult intestine, with expression of this transcription factor essential for differentiation of all secretory cell types.
Because Atoh1 is expressed in mature secretory cells and in cells of the progenitor zone, Atoh1 could be required for secretory cell differentiation, cell maintenance, or both. Many studies indicate that Atoh1 actively promotes adoption of a secretory cell fate. Indeed, embryonic transgenic founder mice with forced Atoh1 expression in the intestinal epithelium (Vil-Math1 mice) had increased numbers of all secretory cell types (goblet, endocrine, and Paneth) and loss of absorptive cells, demonstrating that Atoh1 is able to actively induce secretory cell types at the expense of absorptive enterocytes. Furthermore, epithelial proliferation was decreased in the Vil-Math1 transgenic mice, which implies that Atoh1 may also mediate Notch regulation of proliferation.
Atoh1 appears to be the key Notch effector in the intestinal epithelium. The Shivdasani laboratory combined inhibition of Notch signaling by RBP-Jκ deletion with Atoh1 deletion to demonstrate that Notch regulation of cellular proliferation, as well as secretory/absorptive cell fate determination, is Atoh1 dependent, suggesting that Notch functions solely through inhibition of Atoh1 expression for these functions. However, Atoh1 is not expressed in stem cells; thus, Notch regulation of stem cell function is Atoh1 independent. The Shroyer laboratory has used genome-wide approaches (ChIPseq and RNAseq) to identify direct targets of Atoh1, demonstrating an important role for the downstream transcription factor Spdef in regulating Atoh1 transcription, as well as hundreds of genes that are Atoh1 transcriptional targets in the small intestine and colon.
6.4.2.2
Atoh1 and the Adaptive Response
Small bowel resection in mice causes an adaptive response, with increased epithelial proliferation and sustained increases in secretory cell number and mucosal surface area, including increased crypt depth and villus height. Atoh1 Δintestine mice had a blunted response to resection with smaller increases in crypt depth. Increased secretory cell number was also observed in mice infected with the parasitic nematode Trichinella spiralis. The secretory cell changes suggest that this worm-induced perturbations in Notch signaling. Accordingly, it was recently described that Atoh1 transcript levels are more than twofold higher in T. spiralis infection, suggesting that the increase in secretory cells may be due to induction and differentiation of an Atoh1-expressing progenitor cell. Thus, regulation of Atoh1 expression, and possibly Notch signaling, may be important for the ability of the intestine to respond to environmental stimuli. In addition, it has been demonstrated that intestinal regeneration following γ-irradiation doses shown to ablate ISCs is dependent on Notch, with roles for Dll1 and Dll4 signaling through Notch1 and Notch2.
6.5
Notch Signaling and Intestinal Stem and Progenitor Cells
Notch signaling regulates several distinct processes in the intestine, including stem cell maintenance, progenitor cell proliferation, cell fate specification, and possibly cell maturation. Because mutations affecting Notch signaling result in altered proliferation, it is reasonable to hypothesize that stem or progenitor cells directly respond to Notch signals. Recent advances in stem cell research have brought renewed vigor into identification and characterization of ISC populations. Many new ISC markers have been identified, and methods for in vitro culture of ISCs have been developed, allowing for more detailed and mechanistic studies of these cells. Currently, there is continued debate about the identity of ISCs with evidence supporting the presence of both facultative and active stem cell populations ( Fig. 6.4 ). Upon injury or loss of active stem cells, quiescent or facultative stem cells can be mobilized to replenish the active stem cell pool. This cellular plasticity includes committed transit-amplifying (TA) progenitors that can revert to stemness upon injury/loss of active stem cells, including progenitors committed to secretory or absorptive fate. Notch signaling in active ISCs was first demonstrated by a lineage tracing experiment that marked a long-lived stem cell capable of undergoing sequential Notch processing and NICD production. Since then, numerous Notch gain-of-function and loss-of-function approaches have been used to not only demonstrate an essential role of Notch signaling in maintaining the ISC pool but also to show that Notch acts iteratively within TA cells to regulate cell fate specification. Thus, a critical balance of Notch activity is required to maintain stem cells and to ensure that correct production of enterocyte and secretory progenitors occurs for crypt homeostasis and intestinal function.

6.5.1
The Active Crypt Base Columnar Stem Cell
Based on the critical role of Wnt signaling for intestinal proliferation, it was hypothesized that Wnt-regulated genes would be expressed in stem and/or progenitor cell populations. Screening of colorectal cancer cells that had been transfected with a dominant-negative Tcf4 construct to block Wnt signaling identified the Wnt-regulated gene Lgr5, which was subsequently found to be expressed in the base of the crypts where the CBC potential stem cells had been described by Cheng and LeBlond. Using an Lgr5-lacZ knockin transgenic mouse and BrdU-labeling studies, it was determined that Lgr5-cells are actively cycling with an average of one round of cell division per day. Lineage tracing for the progeny of Lgr5-cells labeled all epithelial cell types, with a time period of ~ 5 days for a migrating “ribbon” to reach the villus tip, a timing consistent with the known rate of epithelial cell differentiation and migration. Importantly, the labeling was shown to be long lived, with labeled ribbons maintained 14 months after induction. Lgr5 homologues (Lgr4, 5 and 6) encode G-protein coupled transmembrane proteins that act as receptors for R-Spondins and potentiate Wnt signaling. Deficiency of the Lgr5 gene results in neonatal lethality, presumably due to fusion of the tongue to the floor of the mouth, which prevented normal feeding after birth. Lgr5 is expressed in the proliferative intervillus zone of the intestine at this developmental stage, but no effect on proliferation or migration of epithelial cells was observed in Lgr5-deficient embryos. This lack of intestinal phenotype is due to redundant Lgr4 expression. Mice with genetic deletion of both Lgr4 and Lgr5 exhibit rapid demise of intestinal crypts, a phenotype consistent with Wnt pathway inhibition.
Analysis of genes enriched in Lgr5-positive cells identified additional markers of the CBC stem cell: achaete scute-like 2 (Ascl2; also called Mash2), olfactomedin4 (Olfm4), Sox9, and others. Importantly, Olfm4 is a Notch target gene, confirming that the CBC stem cell is a direct cellular target of Notch signaling. Ascl2, a Wnt target gene encoding a transcription factor, was shown to direct the expression of a number of other Wnt target genes, including Lgr5. Transgenic expression of Ascl2 in the intestinal epithelium resulted in crypt hyperplasia and disorganized and branched villi with ectopic proliferative crypts at 2 weeks of age. In contrast, conditional deletion of Ascl2 in the intestine led to disappearance of CBC stem cells within 5 days as shown by loss of Olfm4 expression (although proliferation was not dramatically different compared to controls). Mechanistically, Schuijers et al. recently showed Ascl2 forms a bimodal switch that interprets Wnt levels and cooperates transcriptionally with β-catenin/Tcf4 to stabilize the stem cell identity of Lgr5 CBCs.
6.5.2
Notch Regulation of ISCs
Within the ISC niche, there is significant crosstalk between Wnt and Notch signaling in maintaining the stem cell pool and for stem cell/progenitor cell proliferation. Recent functional studies have demonstrated active Notch1 and Notch2 signaling in CBCs. In addition, Notch target genes Hes1 and Olfm4 are localized to CBCs and both Hes1- and Olfm4-CreER reporter mice produce long-lived lineage tracing events after tamoxifen activation. As described above, many studies have demonstrated that Notch signaling is crucial for the survival and maintenance of the CBC stem cell pool. Within the small ISC niche, CBCs likely receive Notch signaling input from Paneth cells that express Dll1 and Dll4 ; however, some studies have shown that Paneth cells are not required for ISC homeostasis, thus suggesting Notch ligands are expressed in alternate cells. High Notch activity in CBCs, TA progenitors, and enterocyte progenitors promotes cell proliferation through upregulation of Hes transcription factors and subsequent derepression of CDK inhibitors, p21Kip1, and p57Kip2. Conversely, inhibition of Notch signaling using γ-secretase inhibitors or specific inhibition of both Notch1 and Notch2 receptor signaling via treatment with neutralizing antibodies or by genetic deletion reduced progenitor cell proliferation and reduced Lgr5 CBC numbers. While Notch1 and Notch2 have been suggested to have overlapping functions in ISCs, more recent studies indicate that Notch1 is the dominant Notch receptor regulating CBCs. Moreover, inhibiting other Notch signaling components by genetic deletion of the Notch S2 protease Adam10, Dll1 and Dll4 ligands or the NICD transcriptional-binding partner RBP-Jκ results in loss of stem cell markers Olfm4 and Lgr5, reduced progenitor cell proliferation and loss of CBCs. Overall, inhibition of Notch signaling leads to a rapid and dramatic loss of the stem cell pool and crypt collapse resulting in intestinal failure and rapid demise of the animals.
While Notch signaling is required for the maintenance and survival of CBCs, the exact mechanism(s) of how Notch and Wnt signaling regulate the balance between maintaining stem cell activity and differentiation are still unresolved. Interestingly, current findings suggest that Lgr5 CBC cell divisions occur symmetrically and adopt stochastically stem cell or TA fates through neutral competition, whereas Lgr5 CBCs located in the upper part of the niche at the border of the Paneth cell field are more likely to be displaced into the TA cell domain. Recent single-cell transcriptomic studies have identified two Lgr5-expressing cell populations in which one population arises from the other and has characteristics of bipotential progenitors that express both enterocyte and secretory progenitor markers. Because these bipotential progenitors express detectable but low levels of Atoh1 and Notch ligands Dll1 and Dll4, it is conceivable that these Lgr5 cells are poised but as yet not committed by Notch-mediated partitioning into defined enterocyte (Notch Hi , Atoh1 Low ) and secretory (Notch Low , Atoh1 Hi ) lineages (see text in Cell Differentiation section). The application of such single-cell resolution techniques will be an important approach to further investigate the role of Notch signaling within these heterogeneous progenitor cell populations.
6.5.3
Facultative Stem Cell Populations
Lgr5 CBCs are responsible for day-to-day intestinal homeostasis, while other facultative, reserve stem cell populations can be activated during repair or regeneration and are capable of repopulating the stem cell niche and restoring the Lgr5 + stem cell pool. Directly above the CBC/Paneth cell zone, at the + 4 position from the crypt base, is a population of slowly-cycling, “quiescent” stem cells. Markers for + 4 facultative stem cells include Bmi1, mTert, and Hopx. Bmi1 was the first gene reported to mark stem cells at the “+ 4 position,” a location previously noted to contain long-lived label-retaining cells (LRCs) presumed to be stem cells. Lineage tracing with Bmi1-CreER showed infrequent labeling of all mature epithelial cell types, with ribbons of labeled cells persisting for up to 12 months, demonstrating that Bmi1 marks a stem cell. Importantly, when CBC stem cells are depleted in the Lgr5 DTR model of intestinal injury, where treatment with diphtheria toxin specifically ablates Lgr5 CBCs, Bmi1 cells are rapidly mobilized to enter the cell cycle and repopulate the Lgr5 CBC population in the crypt, as demonstrated by a robust increase in lineage tracing. Furthermore, both genetic loss of Bmi1 and ablation of Bmi1 cells achieved by activating diphtheria toxin using a Bmi1-CreER transgenic model, suggest that Bmi1-expressing cells are essential for stem cell function and epithelial cell maintenance.
Many of the + 4 facultative stem cell markers are also expressed in Lgr5 CBCs, further highlighting the concept of plasticity within the stem cell zone but also raising the question as to whether these + 4 stem cells are truly distinct from the Lgr5 stem cell population. Several different secretory and enterocyte progenitors have been shown to act as facultative stem cell populations, including progenitor cells expressing Paneth and/or enteroendocrine cell markers, Dll1-expressing secretory progenitors, Sox9-expressing cells, intestinal alkaline phosphatase-expressing enterocyte progenitors, keratin 19-expressing cells, doublecortin-like kinase 1(DCLK1)-expressing cells, and Neurog3- and Nkx2.2-expressing enteroendocrine cells. The emerging concept is that numerous TA progenitor cells can occupy open CBC stem cell niche positions. The diverse nature of these facultative stem cell populations that can each be activated when CBC stem cells are lost, demonstrates the extensive cellular plasticity within the crypt functioning to maintain intestinal homeostasis during repair or regeneration. Interestingly, recent functional and transcriptomic analysis has demonstrated that Hopx-expressing cells are functionally and molecularly distinct from secretory progenitors, consistent with the idea that multiple progenitor cell populations can serve as facultative stem cells. Similar approaches will be needed to provide a more definitive description of how each population contributes to repopulating the stem cell compartment upon different modes of intestinal injuries or stress.
6.5.4
Intestinal Stem Cell Plasticity and Notch Signaling
Understanding the cellular plasticity between different facultative stem cell populations and Lgr5 CBC cells is a dynamic area of research. Recent work by Shivdasani and colleagues demonstrated that secretory and absorptive progenitors have comparable levels of H3K4me2 and H3K27ac histone marks and DNase I hypersensitivity, indicating a broadly open chromatin structure at most of the same cis-elements in both progenitor cell populations. Because secretory and enterocyte progenitors can function as facultative stem cells, the chromatin dynamics found in these cells may make them susceptible to stem cell reversion upon exposure to appropriate signals. Whether Notch is important for maintaining and regulating the ability of different facultative stem cell populations to repopulate the Lgr5 stem cell compartment and reestablish intestinal homeostasis is currently being investigated. Notch disruption via Adam10 deletion within Lgr5 CBCs leads to stem cell loss with “escaper,” Notch competent, stem cells replenishing the ISC compartment. More recently, it was shown that loss of Bmi1-CreER lineage tracing upon Adam10 deletion can be rescued by activated Notch, suggesting that Adam10-mediated Notch signaling is also required for the maintenance and survival of Bmi1-marked cells.
Some studies have investigated the role of Notch signaling in models of intestinal injury and regeneration. In response to whole-body radiation (> 10 Gy), impaired crypt regeneration was observed after γ-secretase inhibitor treatment and in both Notch1- and Notch2-deleted intestine, suggesting that Notch is required for postinjury regeneration. Musashi-1 (Msi1) is an RNA-binding protein that is thought to function in asymmetric cell division by binding to and repressing Numb, an inhibitor of Notch signaling ( Table 6.1 ). In addition, Msi1 has been shown to upregulate Hes1 expression in neural stem cells, which is essential for neural stem cell self-renewal. In the intestine, Msi1 is expressed in numerous intestinal progenitor cell populations, including stem cells and more short-lived progenitors, suggesting a potential role in the regulation of Notch signaling. However, a recent study demonstrated that Msi proteins are dispensable for normal homeostasis and self-renewal of active ISCs. Intriguingly, though, it was demonstrated that after radiation-induced injury, Msi proteins are both necessary and sufficient to drive exit of facultative stem cells from quiescence to reenter the cell cycle. Whether Msi1 modulates Notch signaling in this regenerative response warrants further investigation.
6.5.5
Other Progenitor Cell Markers
Prominin1 (Prom1; also called CD133) has been used as a surface marker for isolation of stem cells in many tissues. Knockin transgenic mouse models were studied to determine Prom1 expression patterns in the intestine, with expression detected in both Lgr5 CBC stem cells and TA cells. Lineage tracing of the progeny from Prom1-expressing cells showed that cells from all epithelial lineages were labeled and that labeling persisted for at least 60–75 days. Interestingly, the frequency of tracing events observed 7 days after induction of labeling was only 10% of that observed 1 day after induction, suggesting that the majority of Prom1-positive cells are short-lived progenitor cells and not long-lived stem cells.
6.6
Cell Fate Specification
Differentiation of intestinal progenitors to mature secretory cell types involves a cell becoming competent for the secretory cell fate, specification to a particular secretory lineage and, finally, terminal differentiation ( Fig. 6.5 ). As discussed above, many reports show that Atoh1 is the critical factor for specification to the secretory lineage, although it is still poorly understood how progenitor cells become competent or how they are further specified into different secretory cell types. Data from Atoh1 loss-of-function mouse models were originally interpreted to demonstrate the existence of an Atoh1-expressing common secretory progenitor cell that was capable of differentiating into all of the mature secretory cell types (i.e., goblet, Paneth, enteroendocrine, and tuft cells). Alternatively, cell fate mapping studies suggested a different mechanism in which secretory cell types may each have distinct progenitors. These studies, which followed the progeny of single, randomly labeled clones in the intestine, identified short-lived progenitors that formed enterocytes together with a single type of secretory cell (goblet or endocrine), suggesting that selection of a particular secretory cell type likely occurs prior to the Notch-regulated choice between absorptive and secretory cell fate. Marked clones containing both secretory cell types and no enterocytes were never observed, suggesting that a common secretory progenitor does not exist or is extremely short lived. Due to their slow turnover rate, Paneth cell-containing clones were not detected in these cell fate mapping studies. Thus, this analysis did not address the possible existence of a shared goblet/Paneth cell progenitor (discussed below).

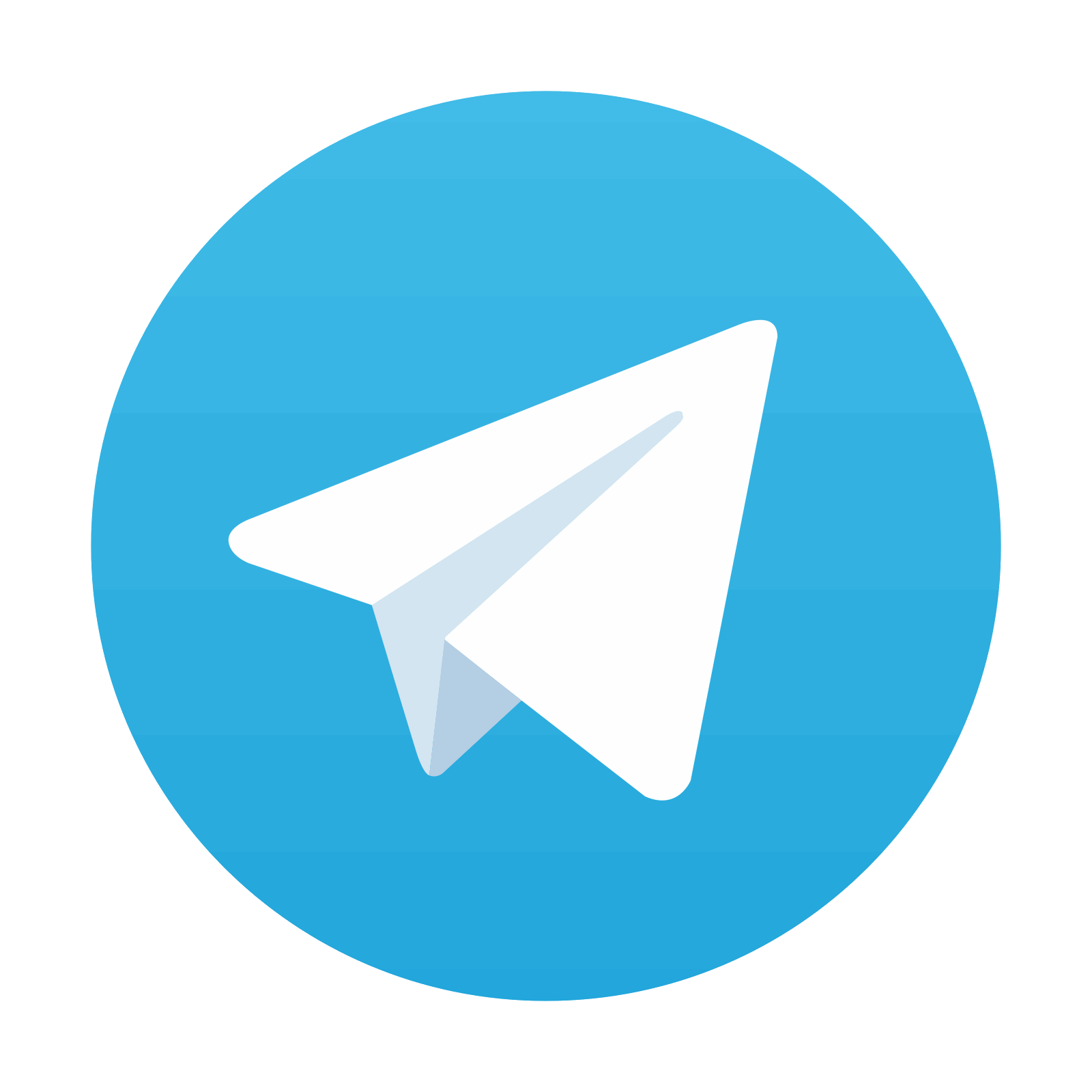
Stay updated, free articles. Join our Telegram channel

Full access? Get Clinical Tree
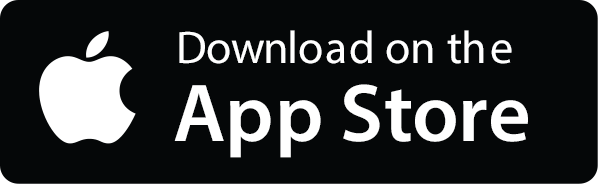
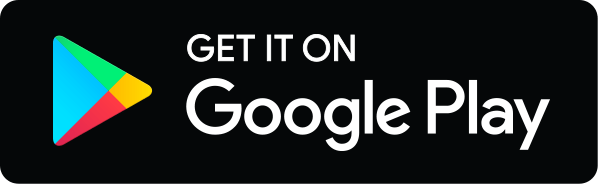
