Introduction
The bladder, in concert with the urethra and the pelvic floor, is responsible for storage and periodic expulsion of urine. The integrated function of these components of the lower urinary tract (LUT) is dependent on a complex control system in the brain, spinal cord and peripheral ganglia, and on local regulatory factors (de Groat, 2006). Dysfunction of the central nervous control systems or of the components of the LUT can produce insufficient voiding and retention of urine, or different types of urinary incontinence (mainly urgency and stress incontinence), or the symptom complex of the “overactive bladder” (OAB), characterized by urgency, frequency with or without urgency incontinence, often with nocturia (Abrams et al, 2002).
Pharmacologic treatment of urinary incontinence and LUT symptoms (LUTSs) including OAB is the main option, and several drugs with different modes and sites of action have been tried (Andersson, 2007; Andersson and Wein, 2004; Andersson et al, 2009a, 2009b; Ouslander, 2004; Zinner et al, 2004). However, to be able to optimize treatment, knowledge about the mechanisms of micturition and of the targets for treatment is necessary. Theoretically, failure to store urine can be improved by agents that decrease detrusor activity and increase bladder capacity, and/or increase outlet resistance.
Neural Circuits Controlling Storage and Expulsion of Urine
Normal micturition occurs in response to afferent signals from the LUT (Birder et al, 2010; de Groat, 2006; Fowler et al, 2008). Both bladder filling and voiding are controlled by neural circuits in the brain, spinal cord, and peripheral ganglia. These circuits coordinate the activity of the smooth muscle in the detrusor and urethra with that of the striated muscles in the urethral sphincter and pelvic floor. Suprapontine influences are believed to act as on–off switches to shift the LUT between the two modes of operation: storage and elimination. In adults, urine storage and voiding are under voluntary control and depend upon learned behavior. In infants, however, these switching mechanisms function in a reflex manner to produce involuntary voiding. In adults, injuries or diseases of the central nervous system (CNS) can disrupt the voluntary control of micturition and cause the reemergence of reflex micturition, resulting in OAB and detrusor overactivity (DO). Because of the complexity of the CNS control of the LUT, OAB and DO can occur as a result of a variety of neurological disorders as well as changes in the peripheral innervation and smooth and skeletal muscle components (Andersson and Arner 2004; Andersson and Wein, 2004).
Filling of the bladder and voiding involve a complex pattern of afferent and efferent signaling in parasympathetic (pelvic nerves), sympathetic (hypogastric nerves), and somatic (pudendal nerves) pathways. These pathways constitute reflexes, which either keep the bladder in a relaxed state, enabling urine storage at low intravesical pressure, or which initiate bladder emptying by relaxing the outflow region and contracting detrusor. Integration of the autonomic and somatic efferents result in that contraction of the detrusor muscle is preceded by a relaxation of the outlet region, thereby facilitating bladder emptying. On the contrary, during the storage phase, the detrusor muscle is relaxed and the outlet region is contracted to maintain continence.
Parasympathetic Pathways
The sacral parasympathetic pathways mediate contraction of the detrusor smooth muscle and relaxation of the outflow region. The preganglionic parasympathetic neurons are located to the sacral parasympathetic nucleus (SPN) in the spinal cord at the level of S2–S4. The axons pass through the pelvic nerves and synapse with the postganglionic nerves either in the pelvic plexus, in ganglia on the surface of the bladder (vesical ganglia), or within the walls of the bladder and urethra (intramural ganglia). The ganglionic neurotransmission is predominantly mediated by acetylcholine acting on nicotinic receptors, although the transmission can be modulated by adrenergic, muscarinic, purinergic, and peptidergic presynaptic receptors. The postganglionic neurons in the pelvic nerve mediate the excitatory input to the normal human detrusor smooth muscle by releasing acetylcholine acting on muscarinic receptors (see later). However, an atropine-resistant (nonadrenergic, noncholinergic [NANC]) contractile component is regularly found in the bladders of most animal species. Such a component can also be demonstrated in functionally and morphologically altered human bladder tissue (O’Reilly et al, 2002), but contributes only to a few percent to normal detrusor contraction (Andersson and Wein, 2004). Adenosine triphosphate (ATP) is the most important mediator of the NANC contraction, although the involvement of other transmitters cannot be ruled out (Andersson and Wein, 2004). The pelvic nerve also conveys parasympathetic nerves to the outflow region and the urethra. These nerves exert an inhibitory effect on the smooth muscle, by releasing nitric oxide and other transmitters (Andersson and Wein, 2004).
Sympathetic Pathways
The sympathetic innervation of the bladder and urethra originates from the intermediolateral nuclei in the thoracolumbar region (T10–L2) of the spinal cord. The axons leave the spinal cord via the splanchnic nerves and travel either through the inferior mesenteric ganglia (IMF) and the hypogastric nerve or pass through the paravertebral chain to the lumbosacral sympathetic chain ganglia and enter the pelvic nerve. Thus, sympathetic signals are conveyed in both the hypogastric nerve and the pelvic nerve. The ganglionic sympathetic transmission is, like the parasympathetic preganglionic transmission, predominantly mediated by acetylcholine acting on nicotinic receptors. Some preganglionic terminals synapse with the postganglionic cells in the paravertebral ganglia or in the IMF, while other synapse closer to the pelvic organs and short postganglionic neurons innervate the target organs. Thus, the hypogastric and pelvic nerves contain both pre- and postganglionic fiber. The predominant effect of the sympathetic innervation is to contract the bladder base and the urethra. In addition, the sympathetic innervation inhibits the parasympathetic pathways at spinal and ganglionic levels. In the human bladder, electrical field stimulation in vitro causes nerve release of noradrenaline, which in the normal detrusor causes relaxation. However, the importance of the sympathetic innervation for relaxation of the human detrusor has never been established. In contrast, in several animal species, the adrenergic innervation has been demonstrated to mediate relaxation of the detrusor during filling (Andersson and Arner, 2004).
Somatic Pathways
The somatic innervation of the urethral rhabdosphincter and of some perineal muscles (eg, compressor urethrae and urethrovaginal sphincter) is provided by the pudendal nerve. These fibers originate from sphincter motor neurons located in the ventral horn of the sacral spinal cord (levels S2–S4) in a region called Onuf’s (Onufrowicz’s) nucleus (Thor and de Groat, 2010; Thor and Donatucci, 2004).
Afferent Pathways
The afferent nerves to the bladder and urethra originate in the dorsal root ganglia at the lumbosacral level of the spinal cord and travel via the pelvic nerve to the periphery (de Groat and Yoshimura, 2010; Kanai and Andersson, 2010). Some afferents originate in dorsal root ganglia at the thoracolumbar level and travel peripherally in the hypogastric nerve. The afferent nerves to the striated muscle of the external urethral sphincter travel in the pudendal nerve to the sacral region of the spinal cord. The most important afferents for the micturition process are myelinated Aδ-fibers and unmyelinated C-fibers traveling in the pelvic nerve to the sacral spinal cord, conveying information from receptors in the bladder wall. The Aδ-fibers respond to passive distension and active contraction, thus conveying information about bladder filling. The activation threshold for Aδ-fibers is 5–15 mm H2O. This is the intravesical pressure at which humans report the first sensation of bladder filling. C-fibers have a high mechanical threshold and respond primarily to chemical irritation of the bladder urothelium/suburothelium or to cold. Following chemical irritation, the C-fiber afferents exhibit spontaneous firing when the bladder is empty and increased firing during bladder distension. These fibers are normally inactive and are therefore termed “silent fibers.” Afferent information about the amount of urine in the bladder is continuously conveyed to the mesencephalic periaqueductal gray (PAG), and from there to the pontine micturition center (PMC), also called Barrington’s nucleus (Holstege, 2005; Kuipers et al, 2006).
Afferent Signaling from the Urothelium/Suburothelium
Recent evidence suggests that the urothelium/suburothelium may serve not only as a passive barrier but also as a specialized sensory and signaling unit, which, by producing nitric oxide, ATP, and other mediators, can control the activity in afferent nerves, and thereby the initiation of the micturition reflex (Andersson, 2002; Birder, 2010; Birder and de Groat, 2007; de Groat, 2004). The urothelium has been shown to express, for example, nicotinic, muscarinic, tachykinin, adrenergic, bradykinin, and transient receptor potential (TRP) receptors (Birder, 2010; Birder and de Groat, 2007). Low pH, high K+, increased osmolality, and low temperatures can all influence afferent nerves, possibly via effects on the vanilloid receptor (capsaicin- [CAP] gated ion channel, TRPV1), which is expressed both in afferent nerve terminals and in the urothelial cells (Birder et al, 2001; Birder et al, 2002). A network of interstitial cells, extensively linked by Cx43-containing gap junctions, was found to be located beneath the urothelium in the human bladder (Brading and McCloskey, 2005; McCloskey, 2010; Sui et al, 2002; Sui et al, 2004). This interstitial cellular network was suggested to operate as a functional syncytium, integrating signals and responses in the bladder wall. The firing of suburothelial afferent nerves, conveying sensations and regulating the threshold for bladder activation, may be modified by both inhibitory (eg, nitric oxide) and stimulatory (eg, ATP, tachykinins, prostanoids) mediators. ATP, generated by the urothelium, has been suggested as an important mediator of urothelial signaling (Andersson, 2002). Supporting such a view, intravesical ATP induces DO in conscious rats (Pandita and Andersson, 2002). Furthermore, mice lacking the P2X3 receptor were shown to have hypoactive bladders (Cockayne et al, 2000; Vlaskovska et al, 2001). Interstitial cells can also be demonstrated within the detrusor muscle (Brading and McCloskey, 2005; McCloskey, 2010). They may be involved in impulse transmission, but their role has not been clarified.
There seem to be other, thus far unidentified, factors in the urothelium that could influence bladder function (Andersson and Wein, 2004). Even if these mechanisms can be involved in, for example, the pathophysiology of OAB, their functional importance remains to be established.
Neural Control of Bladder Filling
During the storage phase, the bladder has to relax in order to maintain a low intravesical pressure. Urine storage is regulated by two separate storage reflexes, of which one is sympathetic (autonomic) and the other is somatic (Thor and Donatucci, 2004). The sympathetic storage reflex (pelvic-to-hypogastric reflex) is initiated as the bladder distends (myelinated Aδ-fibers) and the generated afferent activity travels in the pelvic nerves to the spinal cord. Within the spinal cord, sympathetic firing from the lumbar region (L1–L3) is initiated, which, by effects at the ganglionic level, decreases excitatory parasympathetic inputs to the bladder. Postganglionic neurons release noradrenaline, which facilitates urine storage by stimulating β3-adrenoceptors (ARs) in the detrusor smooth muscle (see later). As mentioned previously, there is little evidence for a functionally important sympathetic innervation of the human detrusor, which is in contrast to what has been found in several animal species. The sympathetic innervation of the human bladder is found mainly in the outlet region, where it mediates contraction. During micturition, this sympathetic reflex pathway is markedly inhibited via supraspinal mechanisms to allow the bladder to contract and the urethra to relax. Thus, the Aδ afferents and the sympathetic efferent fibers constitute a vesico-spinal-vesical storage reflex, which maintains the bladder in a relaxed mode while the proximal urethra and bladder neck are contracted.
In response to a sudden increase in intra-abdominal pressure, such as during a cough, laugh, or sneeze, a more rapid somatic storage reflex (pelvic-to-pudendal reflex), also called the guarding or continence reflex, is initiated. The evoked afferent activity travels along myelinated Aδ afferent nerve fibers in the pelvic nerve to the sacral spinal cord, where efferent somatic urethral motor neurons, located in the nucleus of Onuf, are activated. Afferent information is also conveyed to the PAG and from there to the PMC (the L region). From this center, impulses are conveyed to the motor neurons in the nucleus of Onuf. Axons from these neurons travel in the pudendal nerve and release acetylcholine, which activates nicotinic cholinergic receptors on the rhabdosphincter, which contracts. This pathway is tonically active during urine storage. During sudden abdominal pressure increases, however, it becomes dynamically active to contract the rhabdosphincter. During micturition, this reflex is strongly inhibited via spinal and supraspinal mechanisms to allow the rhabdosphincter to relax and permit urine passage through the urethra. In addition to this spinal somatic storage reflex, there is also supraspinal input from the pons, which projects directly to the nucleus of Onuf and is of importance for voluntary control of the rhabdosphincter (Blok et al, 1997; Holstege, 2005; Sugaya et al, 2005).
Neural Control of Bladder Emptying
Electrophysiological experiments in cats and rats provide evidence for a voiding reflex mediated through a vesico-bulbo-vesical pathway involving neural circuits in the pons, which constitute the PMC. Other regions in the brain, important for micturition, include the hypothalamus and cerebral cortex (Fowler et al, 2008; Griffiths, 2004; Griffiths et al, 2005; Holstege, 2005). Bladder filling leads to increased activation of tension receptors within the bladder wall and thus to increased afferent activity in Aδ-fibers. These fibers project on spinal tract neurons mediating increased sympathetic firing to maintain continence as discussed earlier (storage reflex). In addition, the spinal tract neurons convey the afferent activity to more rostral areas of the spinal cord and the brain. As mentioned previously, one important receiver of the afferent information from the bladder is the PAG in the rostral brain stem (Fowler et al, 2008; Holstege, 2005; Kuipers et al, 2006). The PAG receives information both from afferent neurons in the bladder and from more rostral areas in the brain, that is, cerebral cortex and hypothalamus. This information is integrated in the PAG and the medial part of the PMC (the M region), which also control the descending pathways in the micturition reflex. Thus, PMC can be seen as a switch in the micturition reflex, inhibiting parasympathetic activity in the descending pathways when there is low activity in the afferent fibers and activating the parasympathetic pathways when the afferent activity reaches a certain threshold. The threshold is believed to be set by the inputs from more rostral regions in the brain. In cats, lesioning of regions above the inferior colliculus usually facilitates micturition by elimination of inhibitory inputs from more rostral areas of the brain. On the other hand, transections at a lower level inhibit micturition. Thus, the PMC seems to be under a tonic inhibitory control. A variation of the inhibitory input to PMC results in a variation of bladder capacity. Experiments on rats have shown that the micturition threshold is regulated by, for example, gamma-aminobutyric acid (GABA)-ergic inhibitory mechanisms in the PMC neurons.
Spinal lesions rostral to the lumbosacral level interrupt the vesico-bulbo-vesical pathway and abolish the supraspinal and voluntary control of micturition (Anderson and Wein, 2004). This results initially in an areflexic bladder accompanied by urinary retention. An automatic vesico-spinal-vesical micturition reflex develops slowly, although voiding is generally insufficient due to bladder-sphincter dyssynergia, that is, simultaneous contraction of bladder and urethra. It has been demonstrated in chronic spinal cats that the afferent limb of this reflex is conveyed through unmyelinated C-fibers, which usually do not respond to bladder distension, suggesting changed properties of the afferent receptors in the bladder. Accordingly, the micturition reflex in chronic spinal cats is blocked by CAP, which blocks C-fiber–mediated neurotransmission.
Targets for Pharmacologic Intervention
Anatomically, several CNS regions may be involved in micturition control: supraspinal structures, such as the cortex and diencephalon, midbrain, and medulla, and also spinal structures (Fowler et al, 2008; Fowler and Griffiths, 2010; Griffiths, 2004; Griffiths et al, 2005; Holstege, 2005; Sugaya et al, 2005). Several transmitters are involved in the micturition reflex pathways described earlier and may be targets for drugs aimed for control of micturition (de Groat and Yoshimura, 2001). However, few drugs with a CNS site of action have been developed (Andersson and Pehrson, 2003).
Endogenous opioid peptides and corresponding receptors are widely distributed in many regions in the CNS of importance for micturition control (de Groat and Yoshimura, 2001). It has been well established that morphine, given by various routes of administration to animals and humans, can increase bladder capacity or block bladder contractions. Furthermore, given intrathecally to anesthetized rats and intravenously to humans, the mu-opioid receptor antagonist, naloxone, has been shown to stimulate micturition, suggesting that a tonic activation of mu-opioid receptors has a depressant effect on the micturition reflex. However, intrathecal naloxone was not effective in stimulating micturition in conscious rats at doses blocking the effects of intrathecal morphine (Andersson and Wein, 2004).
Morphine given intrathecally was effective in patients with DO due to spinal cord lesions, but it was associated with side effects, such as nausea and pruritus. Further side effects of opioid receptor agonists comprise respiratory depression, constipation, and abuse (Andersson and Wein, 2004). Attempts have been made to reduce these side effects by increasing selectivity toward one of the different opioid receptor types. At least three different opioid receptors—μ, δ, and κ—bind stereospecifically with morphine and have been shown to interfere with voiding mechanisms. Theoretically, selective receptor actions, or modifications of effects mediated by specific opioid receptors, may have useful therapeutic effects for micturition control.
Tramadol is a well-known analgesic drug. By itself, it is a weak mu-receptor agonist, but it is metabolized to several different compounds, some of them almost as effective as morphine at the mu-receptor. However, the drug also inhibits serotonin (5-HT) and noradrenaline reuptake (Raffa and Friderichs, 1996). This profile is of particular interest, since both mu-receptor agonism and amine reuptake inhibition may be useful principles for treatment of DO/OAB.
When tramadol is given to a normal, awake rat, the most conspicuous changes in the cystometrogram are increases in threshold pressure and bladder capacity. Naloxone can more or less completely inhibit these effects (Pandita et al, 2003). However, there are differences between the effects of tramadol and morphine. Morphine has a very narrow range between the doses causing inhibition of micturition and those increasing bladder capacity and evoking urinary retention. Tramadol has effects over a much wider range of doses, which means that it could be therapeutically more useful for micturition control. It may be speculated that the difference is dependent on the simultaneous influence of the 5-HT and noradrenaline uptake inhibition (Pandita et al, 2003).
In rats, tramadol abolished experimentally induced DO caused by cerebral infarction (Pehrson et al, 2003). Tramadol also inhibited DO induced by apomorphine in rats (Pehrson and Andersson, 2003)—a model of bladder dysfunction in Parkinson’s disease. Whether or not tramadol may have a clinically useful effect on DO/OAB remains to be studied in randomized controlled clinical trials (RCTs).
Safarinejad and Hosseini (2006) evaluated in a double-blind, placebo-controlled, randomized study the efficacy and safety of tramadol in patients with idiopathic DO. A total of 76 patients 18 years or older were given 100 mg tramadol sustained release every 12 hours for 12 weeks. Clinical evaluation was performed at baseline and every 2 weeks during treatment. Tramadol significantly reduced the number of incontinence periods and induced significant improvements in urodynamic parameters. The main adverse event was nausea. It was concluded that in patients with nonneurogenic DO, tramadol provided beneficial clinical and urodynamic effects. Even if tramadol may not be the best suitable drug for treatment of LUTS/OAB, the study proofs the principle of modulating micturition via the mu-receptor.
Lumbosacral autonomic, as well as somatic, motor nuclei (Onuf’s nuclei) receive a dense serotonergic input from the raphe nuclei, and multiple 5-HT receptors have been found at sites where afferent and efferent impulses from and to the LUT are processed (Ramage, 2006). The main receptors shown to be implicated in the control of micturition are the 5-HT1A, 5-HT2, and 5-HT7 receptors (Ramage, 2006). There is some evidence in the rats for serotonergic facilitation of voiding; however, the descending pathway is essentially an inhibitory circuit, with 5-HT as a key neurotransmitter.
It has been speculated that selective serotonin reuptake inhibitors (SSRIs) may be useful for treatment of DO/OAB. On the other hand, there are reports suggesting that the SSRIs in patients without incontinence actually can cause incontinence, particularly in the elderly, and one of the drugs (sertraline) seemed to be more prone to produce urinary incontinence than the others (Movig et al, 2002). Patients exposed to serotonin uptake inhibitors had an increased risk (15 out of 1000 patients) for developing urinary incontinence. So far, there are no RCTs demonstrating the value of SSRIs in the treatment of DO/OAB.
Duloxetine is a combined noradrenaline and serotonin reuptake inhibitor, which has been shown to significantly increase sphincteric muscle activity during the filling/storage phase of micturition in the cat acetic acid model of irritated bladder function (Katofiasc et al, 2002; Thor et al, 1995). Bladder capacity was also increased in this model, both effects mediated centrally through both motor efferent and sensory afferent modulation. The effects of duloxetine was studied in a placebo-controlled study comprising women with OAB (Steers et al, 2007) and was, compared with placebo, shown to cause significant improvements or decreases in voiding and incontinence episodes, for increases in the daytime voiding intervals, and for improvements in quality-of-life (I-QoL) scores. Urodynamic studies showed no significant increases in maximum cystometric capacity or in the volume threshold for DO.
Both in the brain and the spinal cord, GABA has been identified as a main inhibitory transmitter (de Groat and Yoshimura, 2001). GABA functions appear to be triggered by binding of GABA to its inotropic receptors, GABAA and GABAC, which are ligand-gated chloride channels, and its metabotropic receptor, GABAB (Chebib and Johnston, 1999). Since blockade of GABAA and GABAB receptors in the spinal cord and brain (Pehrson and Andersson, 2002) stimulated rat micturition, an endogenous activation of GABAA+B receptors may be responsible for continuous inhibition of the micturition reflex within the CNS. In the spinal cord, GABAA receptors are more numerous than GABAB receptors, except for the dorsal horn where GABAB receptors predominate.
Experiments using conscious and anesthetized rats demonstrated that exogenous GABA, muscimol (GABAA receptor agonist), and baclofen (GABAB receptor agonist) given intravenously, intrathecally, or intracerebroventricularly inhibit micturition (Pehrson et al, 2002). Baclofen given intrathecally attenuated oxyhemoglobin-induced DO, suggesting that the inhibitory actions of GABAB receptor agonists in the spinal cord may be useful for controlling micturition disorders caused by C-fiber activation in the urothelium and/or suburothelium (Pehrson et al, 2002).
Stimulation of the PMC results in an immediate relaxation of the external striated sphincter and a contraction of the detrusor muscle of the bladder demonstrated in cats a direct pathway from the PMC to the dorsal gray commissure of the sacral cord (Blok et al, 1997). It was suggested that the pathway produced relaxation of the external striated sphincter during micturition via inhibitory modulation by GABA neurons of the motoneurons in the sphincter of Onuf (Blok et al, 1997). In rats, intrathecal baclofen and muscimol ultimately produced dribbling urinary incontinence (Pehrson et al, 2002).
Thus, normal relaxation of the striated urethral sphincter is probably mediated via GABAA receptors (Pehrson et al, 2002; Pehrson and Andersson, 2002), GABAB receptors having a minor influence on motoneuron excitability (Rekling et al, 2000).
Gabapentin was originally designed as an anticonvulsant GABA mimetic capable of crossing the blood–brain barrier (Maneuf et al, 2003). The effects of gabapentin, however, do not appear to be mediated through interaction with GABA receptors, and its mechanism of action remains controversial (Maneuf et al, 2003), even if it has been suggested that it acts by binding to a subunit of the α2δ unit of voltage-dependent calcium channels. Gabapentin is also widely used not only for seizures and neuropathic pain but also for many other indications, such as anxiety and sleep disorders, because of its apparent lack of toxicity.
In a pilot study, Carbone et al (2003) reported on the effect of gabapentin on neurogenic DO. These investigators found a positive effect on symptoms and significant improvement in urodynamic parameters after treatment with gabapentin, and suggested that the effects of the drug should be explored in further controlled studies in both neurogenic and nonneurogenic DO. Kim et al (2004) studied the effects of gabapentin in patients with OAB and nocturia not responding to antimuscarinics. They found that 14 out of 31 patients improved with oral gabapentin. The drug was generally well tolerated, and the authors suggested that it can be considered in selective patients when conventional modalities have failed. It is possible that gabapentin and other α2δ ligands (eg, pregabalin and analogs) will offer new therapeutic alternatives.
Noradrenergic neurons in the brainstem project to the sympathetic, parasympathetic, and somatic nuclei in the lumbosacral spinal. Bladder activation through these bulbospinal noradrenergic pathways may involve excitatory α1-ARs, which can be blocked by α1-AR antagonists (Yoshiyama et al, 2000). In rats undergoing continuous cystometry, doxazosin, given intrathecally, decreased micturition pressure, both in normal rats and in animals with postobstruction bladder hypertrophy. The effect was much more pronounced in the animals with hypertrophied OABs. Doxazosin given intrathecally, but not intra-arterially, to spontaneously hypertensive rats exhibiting bladder overactivity, normalized bladder activity (Persson et al, 1998). It was suggested that doxazosin has a site of action at the level of the spinal cord and ganglia.
A central site of action for α1-AR antagonists has been discussed as an explanation for the beneficial effects of these drugs in LUTS (especially storage symptoms) associated with benign prostatic hyperplasia (BPH) (Andersson and Gratzke, 2007; Andersson and Wein, 2004).
Patients with Parkinson’s disease may have neurogenic DO, possibly as a consequence of nigrostriatal dopamine depletion and failure to activate inhibitory D1 receptors (Andersson, 2004). However, other dopaminergic systems may activate D2 receptors, facilitating the micturition reflex. Apomorphine, which activates both D1 and D2 receptors, induced bladder overactivity in anesthetized rats via stimulation of central dopaminergic receptors. The effects were abolished by infracollicular transection of the brain and by prior intraperitoneal administration of the centrally acting dopamine receptor blocker, spiroperidol. It has been shown that the DO induced by apomorphine in anesthetized rats resulted from synchronous stimulation of the micturition centers in the brainstem and spinal cord, and that the response was elicited by stimulation of both dopamine D1 and D2 receptors. Blockade of central dopamine receptors may be expected to influence voiding; however, the therapeutic potential of drugs having this action has not been established (Andersson and Wein, 2004).
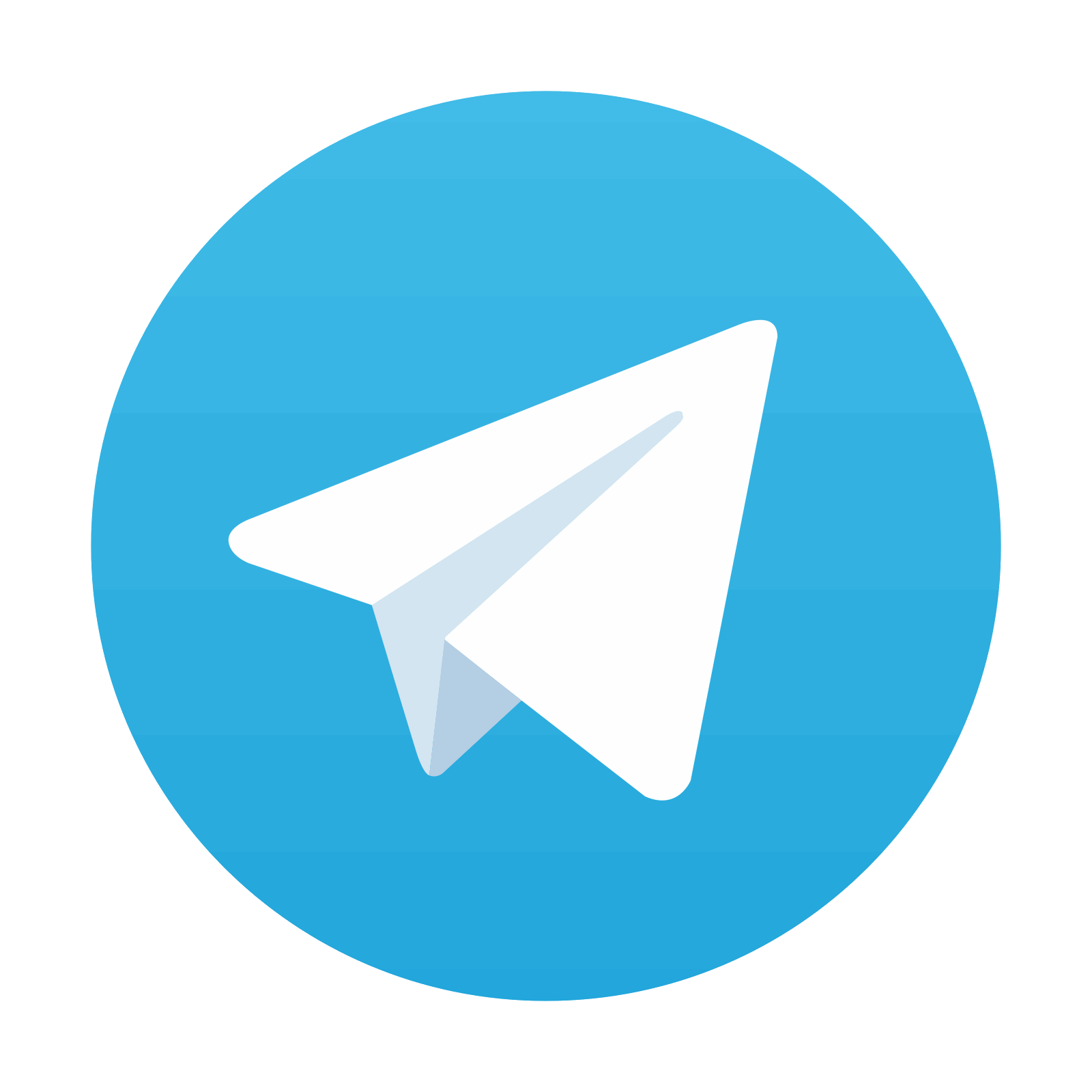
Stay updated, free articles. Join our Telegram channel

Full access? Get Clinical Tree
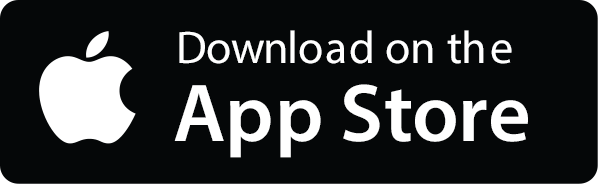
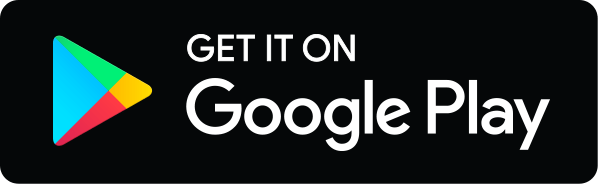