Abstract
The human colon absorbs water and electrolytes stores feces until elimination is socially convenient, and salvages nutrients by bacterial metabolism of nutrients that were not absorbed in the small intestine. Colonic motility is characterized by patterned contractions of longitudinal and circular muscle layers, which result from interactions between several types of cells in the gut wall (neurons, glia, interstitial cells of Cajal, smooth muscle cells, enteroendocrine cells, resident white blood cells, and fibroblast-like cells) and which are regulated by the extrinsic nervous system. Motility is closely integrated with colonic secretion and absorption. This chapter reviews colonic motility and where possible, relates anatomy, coordinated motor patterns, and propulsion of material to their underlying cellular and molecular mechanisms. Since there are considerable interspecies differences in colonic anatomy and function, the emphasis is on colonic sensorimotor functions in health and disease in humans, supplemented by data from other species, where necessary.
Keywords
Colon, Physiology, Constipation, Manometry, Transit, Innervation, Interstitial cells of Cajal, Neurons
Acknowledgment
This study was supported in part by USPHS NIH Grants RO1 DK68055 (AEB) from the National Institutes of Health.
23.1
Introduction
The human colon absorbs water and electrolytes and stores contents until elimination is socially convenient. It also scavenges nutrients produced by bacterial metabolism of undigested carbohydrates. The colon works slowly, taking an average of 65 h to transfer contents from the cecum to the rectum. However, transit can be much slower, without causing any symptoms. Disturbances of colonic motility and sensation, which primarily manifest as constipation, diarrhea, and abdominal discomfort in patients with disorders of function [e.g., irritable bowel syndrome (IBS)], are major reasons for physician visits. These common disorders can be debilitating and last for years. In addition, colonic sensorimotor dysfunctions also occur in organic disorders such as inflammatory bowel disease.
This medical need has stimulated considerable research into normal and disordered colonic functions. Despite this, our understanding of many conditions remains rudimentary. Treatment is often suboptimal because of the complexities. Colonic motility is characterized by patterned contractions of longitudinal and circular muscle layers at each independent point along the organ. Moreover, contractions can influence pressure and flow at some distance. The machinery that drives motility is distributed along the length of the tubular organ. In addition to multiple enteric cell types [e.g., neurons, glia, interstitial cells of Cajal (ICC), smooth muscle cells, enteroendocrine cells, resident white blood cells, and fibroblast-like cells], the extrinsic nervous system significantly affects colonic motility. Muscle contractions act on a non-Newtonian material that changes its fluid content and viscosity as it is propelled along the bowel. Motility is also closely integrated with colonic secretion and absorption. Motility also varies considerably between different parts of the large bowel and there are significant interspecies differences. Finally, the techniques used to measure colonic motility can affect motility themselves and only detect some of the components of colonic motility. These factors confer formidable mechanical complexity to colonic activity; the cardiovascular system, with its four-chambered remote pump and modestly contractile conduits seems relatively simpler by comparison.
Over recent decades, excellent techniques have been developed to analyze receptor function, ion channels, and second messenger pathways in enteric cells. However, it has proved difficult to understand how these components are integrated to generate motor patterns. While we understand many mechanisms of smooth muscle contraction, their interactions with mechanically activated enteric neuronal circuits are still largely a mystery. Smooth muscle action potentials are readily recorded, but their relationship to the mechanics of mixing and propulsion are still obscure. Relating patterns of contraction to their fluid-mechanical consequences is often a matter of speculation or guesswork.
Here, we review our current understanding of colonic motility and, where possible, relate the gross anatomy, coordinated motor patterns, and propulsion of material to their underlying cellular and molecular mechanisms. We will speculate on how gaps between different approaches may be bridged. Because the colon varies so much in its structure between different species and because there are species differences in the effects of mediators, this chapter will focus, where possible, on colonic function in humans. For example, 5-HT 3 receptor antagonists modulate peristaltic reflex activity in in response to mucosal stroking in guinea-pig intestinal preparations, but not in the human gut. Enteric neuronal recordings reveal distinctive features of human enteric neurons, such as the potent postsynaptic excitatory action of histamine through H 3 receptors or the prominent role of protease-activated receptor 1. However, studies in dogs and other large animals have provided valuable data that have not yet been replicated in humans. Furthermore, studies on small laboratory animals, particularly at the cellular and molecular level, have characterized fundamental mechanisms that may also be very germane to human motility.
23.1.1
How Motility and Sensation Are Measured
Patterns of smooth muscle contraction and relaxation facilitate the storage, mixing, and propulsion of content. The muscle cells are the final common effectors in this system. Motility can be monitored either by direct measurement of muscle activity or by indirect measurements (e.g., intraluminal pressure or propulsion). Direct measurements include electrical recordings of membrane potential (e.g., intracellular or whole-cell clamp recording) or extracellular recordings of membrane-associated currents (e.g., action potentials). Intracellular calcium or membrane potential can be measured in vitro using calcium- or voltage-sensitive dyes. In addition, force can be measured with isometric transducers and shortening can be recorded with isotonic transducers or with computerized spatiotemporal mapping. These measures may be supplemented by recordings of intraluminal impedance, which reflect the distribution of content. Finally, a major limitation to understanding visceral afferent processing was overcome by studies demonstrating direct electrophysiological recordings from human visceral afferents ex vivo.
In humans, intraluminal pressure changes can be recorded at multiple sites using perfused tube manometry, solid-state pressure transducers or, more recently, with fiber-Bragg grating based, high-resolution fiber optic catheters. A barostat can measure tone, mechanical properties (i.e., pressure/volume relationships) at a single point and correlate these with perceptions (e.g., of distention). Propulsion or transit of intraluminal content can be measured either ex vivo or in vivo (i.e., with dye-marked content, radioopaque beads, or scintigraphy).
These techniques all have their strengths and limitations. Some must be used in isolated preparations while others are usable both in vitro and in vivo. Spatial and temporal resolution are variable. Some are invasive and modify normal physiology. The great challenge for students of colonic function is to integrate observations from these different techniques into a single conceptual whole. This attempt toward that ambitious goal starts with a brief summary of the anatomy of the colon and then describes the effector cells (i.e., smooth muscle), and intrinsic and extrinsic neural circuitry. Thereafter, we characterize major motor patterns, concentrating on human colonic motor patterns, adding material from studies in animals where necessary. Finally, the endogenous and exogenous factors that modulate motor patterns in normal physiology and major colonic sensorimotor disorders will be outlined.
23.2
Anatomy and Basic Control Mechanisms
23.2.1
Macroscopic Structure of the Colon
In adult cadavers, the colon is approximately 1.5 m long. Anatomically, different regions can be distinguished; the cecum; ascending, transverse, descending, and sigmoid colons; and the rectum, which lies between the rectosigmoid junction and the anal canal. There are interregional differences between the right and left colon, which are derived from the embryological mid- and hindguts, respectively. Their junction is between the right two-thirds and left third of the colon. The right colon is supplied by the vagus nerve, superior mesenteric vessels, and superior mesenteric ganglion. In contrast, the left colon is largely supplied by pelvic nerves from sacral S 2-4 segments, inferior mesenteric vessels, and from the inferior mesenteric ganglion (IMG). The ascending and transverse colons are relatively compliant and well suited to act as a reservoir. In contrast, the descending and sigmoid colons are less compliant and largely function as a conduit, although sometime material may remain in these distal regions for extended periods.
23.2.2
Structure and Activity of Colonic Smooth Muscle
The external smooth muscle of the colon consists of an outer longitudinal and inner circular layer. The circular layer comprises thick bundles of cells, separated by connective tissue septa. In most mammals, the longitudinal muscle layer forms a thin uniform layer. In humans, other primates, horses, guinea pigs, and rabbits the longitudinal muscle in some colonic regions is gathered into thicker taeniae, separated by thinner intertaenial muscle. In the human descending colon, the taeniae thicken and in the sigmoid colon, the longitudinal fibers become more scattered. At the rectum, bundles of longitudinal muscle spread out to encircle the gut, but with thicker anterior and posterior bands. In the anal canal, the longitudinal muscle layer merges with the striated muscle of the external anal sphincter while the circular muscle layer extends into the smooth muscle of the internal anal sphincter.
In much of the human colon, the circular smooth musculature forms arches spanning the taeniae, rather than circular rings. Thus, the colon has a triangular cross section with taeniae at the corners ( Fig. 23.1 ). The taenia coli can be considered to function as cables upon, which arcs of circular muscle are suspended, facilitating efficient contraction of the circular muscle. Thus, a 17% contraction of circular muscle reduces luminal diameter of the colon by 59%. If the longitudinal muscles were of uniform thickness, a 17% contraction of circular muscle would only reduce luminal diameter by 31%. Because the longitudinal smooth muscle is much thinner between taeniae, the intertaenial colonic wall is puckered and thrown into sacculations called haustrations. The haustral septae are not fixed structures; they are sustained contractions of circular muscle, which move slowly. Myogenic activity is not sufficient to explain haustra; neural input contributes too. Haustra move, disappear, and reform during mass propulsion of colonic contents. It has been suggested that haustral out-pouchings may have slower flow than the central lumen and may facilitate colonic fermentation and absorption of water and electrolytes. Changes in the septal orifices may regulate flow between segments. Relaxation of longitudinal muscle and circular muscle layers allows the colon to accommodate content, facilitating storage. It has been suggested that the longitudinal and circular muscle layers are reciprocally innervated, so that contraction of the circular muscle layer is always associated with inhibition of the longitudinal muscle layer. However, such associations may be better explained by mechanical interactions between the two layers of muscle. When the circular muscle shortens, elongation of the segment of intestine must occur to preserve the volume of the tissue making up the colonic wall. It has also been shown that longitudinal and circular muscles can contract synchronously during peristaltic activity; however, the extent to which they contract synchronously or out of phase during other motor patterns is less certain.

23.3
Colonic Electrophysiology
23.3.1
Smooth Muscle
Neurotransmission from active enteric excitatory and inhibitory motor neurons sums with myogenic mechanisms to regulate the excitability and contractility of smooth muscle cells, as in most regions of gut. Resting membrane potential which averages approximately − 50 mV in the colon is not uniform across the thickness of the circular muscle layer, but rather is characterized by a gradient, being up to 36 mV more hyperpolarized in the inner circular smooth muscle region than at the myenteric border. This gradient, which is generated by carbon monoxide, causes a graded contractile response to excitatory inputs, so that weak excitation recruits only the more depolarized smooth muscle and stronger input recruits more hyperpolarized smooth muscle. Studies in heme oxygenase-2 knockout (HO-2-KO) mice, which lack the constitutive form of heme oxygenase, suggest that in the colon the gradient is maintained by carbon monoxide derived from submucosal neurons.
Resting membrane potential is determined by multiple types of K + channels, nonselective cation conductances, possibly mediated via TRP channels, an electrogenic sodium pump, and sodium channels, which all shift resting membrane potential away from the equilibrium potential of K + ions. When muscle is hyperpolarized by neurotransmitters, slow waves reach lower levels of depolarization, evoke fewer Ca 2 + action potentials, less Ca 2 + entry and less forceful contractions. In contrast, excitatory transmitters activate inward currents, depolarize membrane potential, and increase peak depolarization of slow waves. At very depolarized potentials, continuous, low probability opening of Ca 2 + channels (window currents) can occur, producing continuous modest influx of Ca 2 + . Calcium influx via voltage-dependent calcium channels contributes to excitation-contraction coupling and possibly other Ca 2 + -sensitive cell signaling pathways. Both L- and T-type calcium channels are involved. [While CaV1.2 channels are important in excitation–contraction coupling, gastrointestinal (GI) smooth muscle cells express additional types of voltage-dependent calcium channels, as detailed elsewhere in this volume.]
Three major types of myogenic smooth muscle electrical activity primarily contribute to the patterning of motility in the human colon: (i) slow-wave activity with a mean frequency of 2–4 contractions/min, but with intermittent longer duration myogenic potentials; (ii) prepotential oscillations similar to membrane potential oscillations (MPO) in canine colonic muscles; and (iii) action potentials superimposed upon slow waves.
In the human colon, electrical slow waves, which average 12 mV in amplitude, cause Ca 2 + entry by activating voltage-dependent dihydropyridine-sensitive (L-type) Ca 2 + channels; Ca 2 + entry induces electromechanical coupling and segmental contractions. When action potentials are superimposed upon slow waves, Ca 2 + entry is greatly augmented. Between slow waves, the open probability for Ca 2 + channels is low, so action potentials and powerful muscle contractions do not occur. In older studies, it was suggested that contractions were never evoked by slow waves alone; action potentials were always required. However, it is now clear that slow waves in the colon may trigger sufficient Ca 2 + influx to activate the contractile apparatus causing small contractile events that may not have been detected by the strain gauge transducers used in older studies.
Calcium entering smooth muscle cells via voltage-dependent calcium channels leads to phosphorylation of the myosin light chains in the contractile apparatus, which triggers cross bridge cycling and contraction. Phosphorylation is activated when intracellular [Ca 2 + ] rises, primarily via calcium/calmodulin activation of myosin light chain kinase and/or inhibition of myosin light chain phosphatase. However, other kinases, including Rho kinase, can also phosphorylate myosin light chains in a calcium-independent manner and may regulate the calcium sensitivity of the contractile apparatus. Intracellular calcium levels are primarily determined by opening of voltage-dependent calcium channels, especially CaV1.2, other channels may also contribute, including stretch-sensitive nonselective cation channels and channels coupled to G-protein coupled receptors. Influx of Ca 2 + from extracellular fluid may be supplemented by the release of calcium from inositol trisphosphate receptor-coupled intracellular stores, via G q/11 . This pathways is activated via excitatory neurotansmitters from enteric motor neurons, including acetylcholine acting via muscarinic receptors and tachykinins acting on neurokinin receptors.
23.3.2
Introduction to Interstitial Cells of Cajal
In 1911, Ramon y Cajal originally described the cells that now bear his name and speculated that they might modify smooth muscle contraction. However, the contribution of ICC to normal GI motility was uncovered much later by two seminal findings. First, neonatal BALB/c mice injected peritoneally with a monoclonal antibody ACK2 lost ICC and developed lethal intestinal dilatation and hypomotility. ACK2 is a neutralizing antibody to Kit, a type 3 receptor tyrosine kinase which is expressed on ICC. Second, mice with spontaneous mutations in the Kit gene resulting in the loss of function in one allele and decreased function in the other (W/W v mice) lacked intestinal electrical slow waves and myenteric small intestinal ICC, supporting the concept that ICCs were responsible for generating slow waves.
By contrast to smooth muscle, ICC have multiple processes and typically few thick filaments. They regulate gut motility via several mechanisms. They generate electrical slow waves that then propagate through smooth muscle cells via gap junctions (reviewed in Ref. ); they influence the smooth muscle membrane potential and membrane potential gradient ; and they mediate some of the mechanosensitivity of smooth muscle. It has also been suggested that they play an important role in mediating neurotransmission from axons of enteric motor neurons to the smooth muscle although this has recently been questioned.
In addition to ICC, other classes of interstitial cells include ICC-like (ICC-LI) cells, fibroblast-like platelet-derived growth factor receptor-α (PDGFRα +) cells, fibroblast-like cells, and teleocytes. In several species, including primates, ICC, and PDGFRα + form gap junctions with smooth muscle cells and function as a multicellular syncytium referred to as the SIP syncytium. Indeed, nerve varicosities form more junctional connections with ICC than with smooth muscle. Electrical coupling ICC and PDGFRα + also express a variety of receptors for neurotransmitters, hormones, paracrine substances, and inflammatory mediators.
23.3.3
Structure and Organization of ICC
In large animals, several types of ICC in the colon are located between the layers of longitudinal and circular muscle layers, in the plane of the myenteric plexus (MP) (ICC MY , also referred to as ICC AP ) and in the submucosa adjacent to the circular muscle (submuscular ICC, ICC SM , which may also be referred to as submucosal ICC). ICC are also found within the muscle layers (ICC IM ) and a few can be found along the serosa (serosal ICC). In large animals, such as human and dog, a subclass of ICC IM is also found in the septa (septal ICC) that separate bundles of circular muscle cells. ICC MY and ICC SM form extensive networks along the colon and are electrically coupled to one another and to the smooth muscle cells by gap junctions. They also make close appositions and synapse-like contacts with varicose axons of enteric motor neurons ( Fig. 23.2 ). A recent study has suggested the existence of another class of ICC distinguished by close associations with microvessels in several layers of the human colon.

23.3.4
Pacemaker Function
Enteric motor neurons innervate ICC, can influence slow-wave frequency, and influence ICC excitability. In the canine and feline colon, the major slow-wave activity and predominant contractile rhythm (2–4/min) recorded in vitro are associated with the intact submucosal border and hence ICC SM . Similarly, slow-wave pacemaker activity in human colon originates at the submucosal border. At the myenteric border, associated with ICC MY are the pacemakers for the small, rapid (12–20/min) oscillations in membrane potential (MPOs) of longitudinal and circular smooth muscle layers in dog and human. Slow waves and MPOs summate in the central region of circular muscle producing a complex pattern of activity that regulates contractile amplitude and frequency. These cyclic depolarizations then produce action potentials in smooth muscle cells especially when excitatory motor neurons are active ( Fig. 23.3 ).

The rat colon also has several pacemakers albeit organized differently compared to associated humans and larger mammals, such as dogs. The submucosal border is associated with slow-wave activity occurring at around 14/min. The myenteric border is associated with very slow complexes, occurring at a mean frequency below 1/min. These slow depolarizations evoke bursts of smooth muscle action potentials at high frequency and corresponding, large-amplitude prolonged contractions. They are not blocked by tetrodotoxin (which blocks neuronal activity) but are blocked by nifedipine, consistent with their action potentials playing a key role in excitation-contraction coupling. These slow complexes may be related to colonic migrating motor complexes (CMMCs) of rodent colon, although the latter require intact neuronal activity. Alternatively, they may be the rodent equivalent of the long-duration myogenic contractions reported in the canine colon.
ICC-generated slow waves are triggered by pacemaker currents, which evolve into a rapid upstroke when they exceed a depolarization threshold. This rapid upstroke is insensitive to dihydropyridines, which block L-type calcium channels, thereby distinguishing them from smooth muscle action potentials, which are predominantly mediated by L-type calcium channels. The upstroke potential is followed by a plateau potential that is also generated by ICC. While smooth muscle cells can modify the shape of slow waves propagated from ICCs, they do not generate de novo slow waves because they do not have a pacemaker channel. Slow waves from ICCs spread into smooth muscle via gap junctions and activate a variety of smooth muscle ion channels, including L-type calcium channels, contributing powerfully to the contractile response. A Ca 2 + -activated chloride conductance, attributable to the ANO1 channel, plays a key role as a pacemaker current in ICCs. Genetic deactivation of ANO1 abolished slow-wave activity.
23.3.5
Neurotransmission
Structural and functional evidence suggests that cholinergic and nitrergic neurotransmission involves ICC IM in the stomach and ICC DMP in the small intestine. In the colon, close appositions between motor neuron axons and interstitial cells have been reported, suggesting a similar functional arrangement. However, recent analysis has raised doubts about the essential role of ICCs in neurotransmission ; this matter is yet to be settled. It appears that some of the tonic effects of nitric oxide may be mediated via pacemaker ICCs or intramuscular ICCs, although nitric oxide acutely released by enteric motor neuron terminals may not be so dependent on ICCs.
23.3.6
Mechanotransduction
Distension of the colon activates inhibitory enteric reflexes, which cause accommodation of the colonic wall. With further distension, excitatory enteric reflexes are activated, leading to peristalsis. However, when all neural responses are blocked, gut smooth muscle still responds to stretch with depolarization and action potentials. Three mechanisms have been proposed that involve ICC. One is the physical transfer of mechanical information at specialized junctions known as “peg and socket junctions” in which the peg forms a narrow projection of part of the cytoplasm of ICC into smooth muscle cells (the socket). The second is the regulation of the slow wave by mechanical force through the production of prostaglandins, and the third is mechanosensitivity through shear-sensitive ion channels. Sodium channels, which have an established role in cardiac pacemaking, have also been discovered in ICC. A sodium current is expressed in human and dog intestinal circular smooth muscle and ICC but not in smaller animals. This current is mechanosensitive and is activated by membrane shear stress. It is carried by the channel Nav1.5, encoded by SCN5A. The window current of Nav1.5 overlaps with ICC membrane potentials observed during slow waves, suggesting a baseline flow of sodium through Nav1.5. Pharmacologic blockade of the sodium current decreases slow-wave frequency and hyperpolarizes smooth muscle. Stretch of intestinal muscle strips in neuronal blockers and nifedipine (to block L-type Ca 2 + channels) increases the slow-wave frequency. Mutations in SCN5A are associated with GI symptoms in patients with IBS.
23.3.7
ICC Injury, Death, and Recovery
In health, ICC in the human colon and other regions of the GI tract undergo complete turnover every few months. Hence, functional ICC networks are maintained by tightly regulated loss and replacement of ICC. Apoptosis and trans/dedifferentiation, contribute to ICC loss while repair from injury, and differentiation from ICC stem cell precursors replenish ICC. Adult ICC also divide. Factors that affect ICC survival in vitro and in vivo in other tissues include Kit-ligand (stem cell factor or steel factor), nitric oxide (mouse stomach), serotonin via the 5-hydroxytryptamine (5-HT)2B receptor (mouse jejunum), interleukin-9 (cultures), insulin and insulin-like growth factor (IGF)-1 signaling through stem cell factor (mouse stomach), and oxidative stress (mouse stomach).
ICC are reduced in several motility disorders, including slow transit constipation and chronic megacolon. However, the contribution of ICC loss to colonic sensorimotor dysfunctions is unclear since enteric neurons are also depleted in these conditions. It is not known how much damage ICC networks can withstand before functional abnormalities develop. The published literature is conflicting, perhaps because ICC cannot be reliably visualized in formalin-fixed, paraffin-embedded tissue. Human gut tissue requires careful handling because ICC are particularly susceptible to ischemia. In addition, there are limited quantitative data on ICC populations in normal human colon. Antibodies to Ano-1 may be particularly useful in future studies to identify ICC abnormalities since unlike Kit, Ano-1 is not expressed on mast cells.
23.3.8
Fibroblast-Like Platelet-Derived Growth Factor Receptor-α (PDGFR-α) Expressing Cells
Fibroblast-like cells were originally distinguished by features shared with true fibroblasts (e.g., abundant rough endoplasmic reticulum). Now, they can be precisely defined by specific labeling with antibodies for PDGFRα. These cells are intertwined with ICC and axons of excitatory and inhibitory neurons. These cells appear to mediate electrical components of purinergic inhibitory neurotransmission to GI smooth muscle from enteric inhibitory motor neurons. Activation of purinergic P2Y1 receptors on PDGFRα cells leads to opening of small conductance Ca 2 + -activated K + (SK3) channels, also expressed on the same cells. Electrical coupling via gap junctions then causes hyperpolarization and inhibition of smooth muscle cells. The role of PDGFRα cells in colonic motility is currently unknown. Due to the close coupling of smooth muscle cells, interstitial cells, and PDGFRα cells function together as the “SIP” syncytium (after the initial letter of the three cell types ). Recent data suggest interactions between these cell types since nitric oxide causes prejunctional inhibition of purinergic neurotransmitter release in the mouse and human colon
23.4
Innervation of the Colon
The enteric nervous system (ENS) contains sensory neurons, interneurons, and the motor neurons that provide input into the smooth muscle apparatus of the colon. This motor input interacts with myogenic mechanisms to create regional patterns of contraction and relaxation which mix and propel content. Sympathetic and parasympathetic neural pathways, which are modulated by inputs from the central nervous system, modulate colonic motility according to the broader needs of the organism. Extrinsic sensory pathways provide a continuous stream of information to the central nervous system about the mechanical and chemical state of the gut wall and luminal contents. 1
23.4.1
Introduction to the Enteric Nervous System
The ENS is the largest single division of the autonomic nervous system. The cranial and sacral parasympathetic innervation of human gut is mediated by a few tens of thousands of neurons; the sympathetic innervation of the gut amounts to tens of thousands to one hundred thousand neurons. It has been estimated that there may be between 200 and 600 million enteric neurons in the whole GI tract. The ENS directly controls important gut functions by innervating effector tissues, including the smooth muscle layers, epithelial cells and, to a lesser extent, intramural blood vessels, enteroendocrine cells, and lymphoid tissue. Sympathetic and parasympathetic neurons modulate gut functions largely via their inputs onto enteric neurons; though in some cases, they directly innervate effector tissues (see below).
23.4.2
Structure of the Enteric Nervous System
The cell bodies of most enteric neurons are localized in two extensive networks: the MP (sandwiched between the circular and longitudinal muscle layers of the muscularis externa) and the submucous plexus which lies within the submucosal connective tissue. In the human colon, the MP consists of irregularly spaced stellate ganglia joined by thick interganglionic connectives. Each ganglion contains, on average, 70–80 nerve cell bodies and glial cells. In both myenteric and submucous plexuses, human ganglia contained more glia per neuron than in the intensively studied laboratory animal, the guinea pig. Together, the myenteric ganglia and connectives comprise the primary plexus ( Fig. 23.4 ). A secondary plexus (nonganglionated) consists of nerve trunks aligned with circular muscle bundles that innervate the muscle layer and penetrate through it, en route to the submucosa. A tertiary plexus is associated with the longitudinal muscle layer and contains axons of motor neurons that innervate it.

The submucous plexus in human colon actually consists of several distinct, though interconnected layers. These are referred to as Meissner’s plexus (referring to the innermost layer, closest to the mucosa) and Henle’s plexus or Schabadasch’s plexus (referring to the outermost layer nearest the circular muscle). Some authors have distinguished an intermediate plexus. These plexuses contain different populations of nerve cells that can be distinguished on the basis of their size, immunohistochemical coding, and projections. In humans and larger animals, the outer submucous plexus contains motor neurons that project to the external muscle layers and presumably control motility. In contrast, the inner submucosal plexus contains more neurons that project to the mucosa and likely regulates secretomotor and vasomotor activity. In most individuals, there are also a small population of “ectopic” nerve cell bodies in the mucosal plexus that lie between the muscularis mucosa and the glandular tissue; these have coding similar to neurons in the inner submucosal plexus.
Ideally, to understand how the human ENS controls colonic motility, we would have a comprehensive account of all classes of enteric neurons, their properties, connections and their activity during different behaviors. No such account currently exists. For the rather patchy and inconsistent data that are available, the reader is referred to extensive reviews and the present volume. Much of our current understanding of the innervation of the gut comes from the upper gut of laboratory animals; our understanding of colonic innervation in humans is limited. In addition to species differences, techniques vary between laboratories. Our ability to relate events at the cellular level to the real-time behavior of the whole organ is in its infancy although computer modeling can be valuable.
Enteric neurons belong to 15–20 different functional classes. The traditional division of nerve cells as sensory neurons, interneurons, or motor neurons may not be useful for the ENS. For example, neurons characterized by the presence of several long axons arising from the cell body are called the Dogiel-type II neuron. These cells are mechanosensitive and chemosensitive and have therefore been classified as “intrinsic primary afferent neurons” (IPANs). However, these neurons also function as interneurons, since they are potently excited by slow synaptic inputs, which can initiate long trains of action potentials. Moreover, the same cells partly mediate cholinergic secretomotor input to the mucosal epithelium. These cells are sensory, interneurons, and secretomotor neurons. Indeed, many enteric neurons interneurons and motor neurons have direct mechanosensitivity and this may be a widespread characteristic of enteric neurons.
Different classes of enteric neurons can be distinguished by combinations of morphology, histochemical, or immunohistochemical coding and electrophysiological characteristic. The first comprehensive classifications of all functional classes of enteric neurons were in the guinea pig small intestine. This was largely based on combinatorial immunohistochemistry, but has since been supplemented with information from electrophysiological recordings, intracellular dye fills and retrograde tracing studies. The classification scheme has proved very robust and still provides a benchmark for understanding the ENS. Many other studies have compared combinations of markers in enteric neurons without attempting to distinguish all functional classes. This can provide interesting insights. For example, cholinergic and nitrergic neurons account for nearly all myenteric neurons but the proportion of nitrergic neurons (as a percentage of all myenteric neurons is higher in the human colon than the human small intestine. The functional significance of such findings is yet to be established.
23.4.3
Motor Neurons
Final common effector neurons in the ENS innervate smooth muscle, blood vessels (vasomotor neurons), and epithelia (secretomotor neurons). Most relevant to the control of colonic motility are the excitatory and inhibitory motor neurons to the muscularis externa. The longitudinal and circular muscle layers are known to be innervated by separate populations of motor neurons in lab animals and this is also true of the human colon. Excitatory motor neurons in human colon are cholinergic (i.e., contain choline acetyltransferase immunoreactivity), and usually, but not always, contain tachykinins. The axons of circular muscle excitatory motor neurons typically project into the muscle layers close to their cell bodies or up to 11 mm oral to their cell bodies. Once in the muscle they then branch extensively over many millimeters around the circumference, with numerous varicose swelling that are sites of transmitter release. It appears that the majority of close appositions are with ICCs and PGDFRα cells, rather than with smooth muscle directly, at least in some parts of the GI tract. Many excitatory motor neurons also contain a variety of neuromodulators and transmitters including enkephalins and dynorphins. Inhibitory motor neurons to the circular muscle, on the other hand, generally project aborally, from 1 to 19 mm before their axons enter the muscle layers. They account for just under half the motor neurons. They branch extensively in the circular muscle and release a variety of neurochemicals that inhibit smooth muscle contractility. These include nitric oxide (or a related compound), one or more peptides including vasoactive intestinal polypeptide (VIP), pituitary adenylate-cyclase activating peptide (PACAP) and a purine that may be adenosine triphosphate (ATP) or nicotinamide adenine dinucleotide. Polarized projections of inhibitory and excitatory motor neurons to the circular muscle appear to be a common feature in the innervation of gut of many regions including the human colon.
The organization of longitudinal muscle is slightly different. In the guinea pig small intestine and colon, cholinergic excitatory motor neurons to longitudinal muscle generally have short local projections and do not show a preferential polarity, whereas inhibitory motor neurons to the longitudinal muscle of the colon preferentially project aborally before branching.
23.4.4
Interneurons
The polarized projections of excitatory and inhibitory motor neurons extend only over a distance of a few millimeters to a few centimeters, yet polarized reflex pathways in the intestine extend over much greater distances. This is probably because of the contribution of interneurons in enteric neural circuits. Ascending interneurons and descending interneuronal pathways have been identified, but there do not appear to be specialized circumferentially projecting interneurons. In the guinea pig small intestine, the single class of ascending interneuron has projections up to about 15 mm in length and contain immunohistochemical markers, which suggest that acetylcholine and tachykinins are their major transmitters. They make functional chains running up the gut by synapsing onto other interneurons of the same class. In this way, ascending reflexes activated by local stimuli spread far up the intestine beyond the projections of individual motor neurons or interneurons. In the guinea pig colon, chemical coding studies suggest that there may be several classes of ascending interneuron.
There are several different functional classes of descending interneurons. Descending interneurons often form long functional chains running down the gut, synapsing onto other neurons of the same class but contacting other enteric neurons too. In this way, reflexes may extend up and down the colon for distances greater than the individual neuronal components. To speculate, these interneuronal chains may also contribute to switching between patterns of motility, since activation of a single class of interneurons could then modify the activity of circuits over a considerable length of the bowel. Most, descending interneurons are likely to be cholinergic, but they can be divided into different classes on the basis of cotransmitters and modulators that they contain. In the guinea pig small intestine, at least four classes of descending interneuron have been distinguished by immunoreactivity for 5-hydroxytryptamine, somatostatin, and vasoactive intestinal polypeptide, with or with nitric oxide synthase and choline acetyltransferase. Similarly, in the human colon, descending interneurons are more numerous and have longer projections than ascending interneurons and there are at least three classes of interneurons characterized by choline acetyltransferase, nitric oxide synthase, and 5-HT. Compatible with these principles, a model has been proposed that rhythmic motor patterns in the colon may be substantially coordinated by activity in 5-HT-containing descending interneuronal pathways.
23.4.5
Intrinsic Primary Afferent Neurons
Since the earliest studies of reflexes in isolated preparations ex vivo, it was deduced there must be sensory nerves that can be activated by distension, stroking, or chemicals stimuli. Studies on isolated preparations (e.g., Refs. ) confirmed the existence of intrinsic sensory neurons. An important insights came with the finding that Dogiel type II neurons in the guinea pig small intestine (neurons distinguished by having multiple long processes arising from their cell bodies) are mechanosensitive and chemosensitive. Since then, the ion channels and conductances that are responsible for the distinctive long-after hyperpolarizations that follow action potentials in these cells have been characterized. Consistent with this, these neurons are also activated by mediators (including 5-hydroxytryptamine and adenosine triphosphate) released by mucosal enteroendocrine or enterochromaffin cells.
Dogiel type II enteric sensory neurons branch extensively in local enteric ganglia and make slow excitatory synaptic contact with most other classes of enteric neurons, including other Dogiel type II neurons and make fast synaptic contacts with other enteric neurons. Neurons with similar morphological and electrophysiological properties are also located in submucosal ganglia, although this population seems preferentially sensitive to mucosal mechanical stimulation. Dogiel type II neurons constitute about 30% of all myenteric neurons in the small intestine and a smaller proportion in the distal colon. The extensive excitatory interconnections between Dogiel type II neurons suggest that populations of these neurons may be activated together and that this could contribute to setting the threshold for activation of specific motor patterns. Direct evidence for such a role for Dogiel type II neurons has been provided by recording calcium transients in myenteric nerve cell bodies in murine colon during colonic migrating myoelectric complexes. In human small intestine, it was estimated that Dogiel type II neurons comprised about 10% of myenteric neurons, but comparable numbers for the human large intestine are not available.
Other classes of enteric neurons also appear to act as primary afferent neurons, capable of transducing mechanical stimuli. The circular and longitudinal muscle layers are both activated via neural pathways when the guinea pig colon is stretched circumferentially. However, during such stimuli Dogiel type II neurons are not significantly excited and therefore cannot provide the synaptic drive for the motor pattern. Instead, mechanosensory activity was detected in one or more classes of interneurons, which drive circuits via fast, rather than slow excitatory synaptic potentials. The possibility that uni-axonal neurons might be directly mechanosensitive has been directly confirmed. Enteric “viscerofugal” neurons have single axons, which innervate sympathetic neurons in prevertebral ganglia. These neurons show mechanosensitivity in the presence of low [Ca 2 + ], which blocks transmitter release, suggesting that they are not activated by other neurons. Direct activation has been demonstrated with application of mechanical stimuli to their cell bodies, which evokes bursts of action potentials. This idea has been extended by studies using potential-sensitive dyes to monitor electrical activity in enteric neurons during mechanical stimulation. Such studies have shown that a significant proportion of uniaxonal myenteric neurons, including probable interneurons and motor neurons, have direct, rapidly adapting responses to mechanical stimuli that persist when synaptic transmission is blocked. These neurons are found in both guinea pig and mouse colon, alongside slowly adapting mechanoreceptors that may correspond to Dogiel type II neurons.
23.4.6
Other Classes of Enteric Neurons
Other classes of enteric neurons include secretomotor neurons and vasomotor (vasodilator) neurons that control epithelial secretion and blood vessel diameter. These types of neurons may supply collaterals that contact other enteric nerve cell bodies and potentially could interact with motility-generating circuits. Another class, the viscerofugal neurons play an important role in coordinating motility reflexes. These neurons project out of the gut and make synaptic contact onto sympathetic neurons in prevertebral ganglia. Here they provide cholinergic synaptic input to postganglionic sympathetic neurons involved in secretomotor and motility pathways, bypassing the central nervous system. These neurons function as interneurons as they receive prominent fast excitatory synaptic inputs and are directly mechanosensitive, primarily to local strain at their cell bodies. These neurons appear to be synaptically activated in parallel with motor circuits as they often fire bursts of action potentials before contractions. Viscerofugal neurons activate extrinsic reflexes that coordinate activity between different regions of gut such as the rectocolonic inhibitory reflex, which utilizes adrenergic sympathetic pathways.
23.4.7
Sympathetic Innervation
The sympathetic nervous system provides input to all parts of the gut, including the colon. Sympathetic preganglionic neurons have cell bodies in the intermediolateral cell column and project via thoracolumbar ventral roots to sympathetic ganglia, providing powerful cholinergic excitatory input to sympathetic postganglionic neurons located in both paravertebral (sympathetic chain) and prevertebral ganglia. In the colon, the majority of sympathetic neurons from paravertebral ganglia are vasoconstrictor neurons and are not directly involved in regulating motility. Colonic motility is modulated by sympathetic neurons in prevertebral ganglia. Preganglionic fibers from T 12 to L 4 levels of the spinal cord, project in via the thoracic splanchnic nerves, then join the third lumbar splanchnic nerve and branches from the aortic plexus to form the superior hypogastric plexus, located close to the aortic bifurcation at the L4-S1 level. The alternative term for this plexus; “the presacral nerve” is misleading because it is seldom condensed, and it does not resemble a single nerve. Many preganglionic fibers innervate sympathetic (postganglionic) neurons in the superior hypogastric plexus, which project to the colon via the lumbar colonic nerves.
The superior hypogastric plexus also gives rise to right and left hypogastric nerves that descend, uniting with pelvic splanchnic nerves to form the corresponding inferior hypogastric plexuses. Branches from each hypogastric nerve supply the testicular or ovarian plexuses, ureteric plexus, and the plexus on the internal iliac artery. Each hypogastric nerve also joins with the lowest lumbar splanchnic nerve. The inferior hypogastric plexus (corresponding to the “pelvic plexus” in animals) lies posterior to the urinary bladder and beside the rectum and prostate in men. In women, this plexus is also adjacent to the uterus. The inferior hypogastric plexuses in humans include numerous small ganglia containing nerve cell bodies of parasympathetic neurons to the rectum and anal canal, mixed with sympathetic fibers arriving via the hypogastric nerves. Studies in rats indicate that pelvic plexus ganglia also contains the nerve cell bodies of some sympathetic neurons too, which can be identified by the thoracolumbar origin of the preganglionic fibers that innervate them.
Sympathetic neurons receive synaptic drive from preganglionic spinal neurons, collaterals of spinal afferent neurons and from enteric viscerofugal neurons. The postganglionic sympathetic neurons are noradrenergic and contain the marker enyzme tyrosine hydroxylase (TH), but many of them also contain neuropeptides, neuropeptide Y, galanin, and/or somatostatin. They supply intrinsic and extrinsic blood vessels, myenteric, and submucosal enteric ganglia and provide a sparse innervation to the mucosa and to the muscularis externa. At the ileocaecal junction and internal anal sphincter the direct sympathetic innervation of circular smooth muscle is considerably denser. Studies in guinea pigs and pigs have shown distinct classes of sympathetic neurons play different roles: sympathetic neurons to blood vessels contain NPY with TH; those projecting to submucosal ganglia (presumably involved in secretomotor control) contain somatostatin with TH, whereas those that project to the myenteric ganglia (involved with control of motility) contain TH without either peptide. There is also a sympathetic innervation of lymphoid accumulations in the colonic wall where sympathetic neurons appear to be involved in distance transmission to immune cells modulating release of pro and antiinflammatory mediators.
Sympathetic input has potent effects on colonic function. There is evidence for ongoing tonic inhibition of colonic secretion, since disrupting the pathway causes a substantial increase in secretion. This is largely mediated by a strong inhibitory drive to secretomotor neurons in submucosal ganglia, via alpha 2 adrenoceptors. Sympathetic activation also directly contracts sphincters and via indirect effects (i.e., by reducing acetylcholine release from cholinergic neurons), inhibits activation of enteric neurons. Both actions delay GI and colonic transit. In ex vivo animal studies, sympathetic neurons have more pronounced tonic inhibitory effects on colonic secretion than motility. However, the α 2 -adrenergic antagonist yohimbine increased colonic tone and sensation in humans, demonstrating ongoing sympathetically mediated inhibition of colonic motility.
23.4.8
Parasympathetic Innervation
In several species, including primates, the parasympathetic supply to the right colon is derived from the vagus nerve, whose branches reach the prevertebral ganglia (superior hypogastric plexus) and then travel with sympathetic axons to the ceco-ascending and transverse colon. The parasympathetic input to the distal colon is derived from the sacral (S 2-4 ) segments of the spinal cord via the pelvic plexus. Preganglionic parasympathetic fibers originating from ventral rami of the second, third, and often the fourth sacral nerves travel in the pelvic splanchnic nerves and synapse with neurons in the inferior hypogastric plexus or travel directly into the bowel wall. In addition, ascending fibers from the inferior hypogastric plexus travel via superior hypogastric and aortic plexuses to reach the inferior mesenteric plexus, ultimately innervating the descending and sigmoid colon. After entering the colon, these fibers form the ascending colonic nerves or shunt fascicles, traveling cephalad in the plane of the MP to supply a variable portion of the left colon. Sacral parasympathetic pathways to the colon primarily synapse onto myenteric neurons, via excitatory nicotinic cholinergic synapses, leading to increased activity in enteric pathways—although the net effects of this on smooth muscle may be either excitatory or inhibitory. Excitatory pathways are important for colonic propulsive activity, especially during defecation; damage to these pathways can cause severe constipation.
23.4.9
Extrinsic Afferent Pathways
In humans, balloon distention of the left colon evokes abdominal discomfort in the midline or left iliac fossa. The rectum is more sensitive than the colon and can distinguish between flatus and feces. Rectal distention induces rectal or sacral discomfort, akin to the desire to defecate or urgency. The anal canal is exquisitely sensitive, with sensitivity to touch, pain, and temperature comparable to the dorsum of the hand. Every part of the colorectum (and the rest of the gut) is innervated by nociceptors that are activated by large distensions, strong contractions, ischaemia, and inflammatory damage.
The cell bodies of primary afferent neurons are located in ganglia outside the bowel wall. Vagal afferent neurons, with nerve cell bodies located in the nodose and jugular ganglia, project to the proximal colon and accompany vagal efferent parasympathetic pathways. Currently, their exact role in reflex control and sensation is not clear, but they are unlikely to be involved in transmitting pain sensation from the colon.
Spinal primary afferent neurons with nerve cell bodies in the dorsal root ganglia innervate the entire colon and belong to distinct morphological and physiological types. They have been extensively studied in laboratory animals. Lumbar spinal afferents project along the lumbar splanchnic nerves, through the prevertebral IMG and through the lumbar colonic nerves to the colon, where they terminate in sensory endings in the mesentery, serosa, muscular layers, and mucosa throughout the entire colon and rectum. In addition, a population of pelvic spinal afferents, with cell bodies in the sacral dorsal root ganglia, project along the pelvic nerves to the colon and traverse the pelvic/inferior hypogastric plexus en route. Evidence indicates that some of these sacral spinal afferent neurons form a functionally different population from the lumbar spinal afferents, encoding different types of information, particularly from the rectum. Sacral afferents include many low-threshold, wide-dynamic-range mechanoreceptors, which probably are responsible for graded sensations of rectal filling and for activating defecatory reflexes. By contrast, lumbar spinal afferents and some higher threshold sacral afferents are responsible for generating pain sensations from the entire colon and rectum. They respond to gross distention of the bowel wall, traction on the mesenteric membranes, powerful colonic contractions, or chemical stimulation of the mucosa by bile acids, high osmolarity, and other stimuli. Many of these high threshold mechano-nociceptors have transductive endings on both intramural and extramural blood vessels. The sensitivity of many spinal afferents is increased greatly by inflammation in the gut wall. In addition to their role in sensation, spinal afferents also have axon branches (collaterals) in enteric ganglia, prevertebral sympathetic ganglia and on mucosal blood vessels, where they may play a role in generating peripheral reflex hyperemic responses to noxious stimuli.
An important advance has been made by several groups who have recorded from human colonic afferent pathways in ex vivo preparations, which are directly relevant to human patients. The first studies were in human colon and appendix ; since then recordings have been extended to human rectum. These studies have identified different classes of afferents in human distal bowel, including serosal nociceptors, which resemble those described in animal preparations. This approach is highly significant as it allows agents that are effective in animal preparations to be tested in human tissue. A recent study demonstrated that the sodium channel subunit NaV1.7, which plays a key role in somatic nociception is not involved in mouse or human colonic nociception. Another study showed that excitation of murine lumbar splanchnic nerves to the colon by P2Y receptor agonists was mimicked in afferents innervating the human descending colon.
In summary, afferent and efferent (parasympathetic and sympathetic) pathways run in parallel and connect the distal bowel with the neural circuitry in the thoracolumbar and lumbosacral spinal cord via pelvic, rectal, and colonic nerves. The important role of these pathways in both rectal sensation and in generating the normal motility required for defecation is clearly demonstrated by the effects of nerve lesions at several levels. Thus, severing of peripheral nerves and distal spinal cord injury can lead to both the loss of rectal sensation and severely impaired defecatory ability.
23.5
Measuring Colonic Perception and Motor Patterns
Colonic motor activity varies from quiescence, isolated contractions, bursts of contractions to powerful, high-amplitude propagating contractions. One of the great challenges for students of colonic motility is to understand the relationship between local muscle activation, intraluminal pressure, and movement of content. This understanding is necessary to distinguish functionally important patterns of motility and identify reliable biomarkers for disease states and their underlying mechanisms. Our current understanding of motor patterns and perception is limited for the human large bowel. At least in part, this is due to limitations of the methods available to record human colonic motility, in vitro. For this reason, methods of measurement are briefly discussed below.
23.5.1
Assessment of Colonic Sensation in Humans
In humans, colonic (and rectal) perception is usually assessed by asking subjects to indicate when they perceive a given sensation, that is, first threshold, desire to defecate or pain, during balloon distention. A subset of patients with IBS have reduced sensory thresholds, indicative of visceral hypersensitivity.
The rate and pattern of rectal distention influence both contractile responses and perception in health and in IBS. The contractile response to rectal distention is greater during fast than slow distention, perhaps explaining why rapid rectal distention is more likely than slow distention to be perceived in healthy subjects and to evoke increased rectal sensitivity in IBS. The close temporal association between contractions and sensation suggests that sensation may be mediated not by distention per se but by distention-induced contractions. This is consistent with the observation that firing of mechanoreceptors in the distal bowel of laboratory animals is closely related to contractile activity within the receptive field.
An alternative approach to assessing colonic sensation in humans is to ask patients to rate the intensity of perception during balloon distentions of standardized intensity delivered in random order. Perceptual intensity is recorded on separate visual analog scales for gas, desire to defecate and discomfort. With this technique, subjective perceptual ratings reflect the intensity of the stimulus. This technique is responsive to alterations in visceral perception induced by psychological stress and relaxation, and by drugs including the α 2 -agonist and antagonist clonidine and yohimbine, respectively, and, the cholinesterase inhibitor, neostigmine.
23.5.2
Assessment of Colonic Motor Function in Humans
Basic concepts of normal colonic motor activity have been strongly influenced by fluoroscopic studies conducted in animals and humans almost 100 years ago. Later, manometry and colonic transit measurements avoided radiation exposure in humans. The barostat was initially used to assess tone in the stomach, and subsequently in the colon. Recording myoelectrical activity has been used with serosal, mucosal, or intraluminal electrodes, but is fraught with technical difficulties and has fallen out of favor. Some referral centers offer stationary or ambulatory manometry, while the barostat is primarily a research tool.
23.5.3
Assessment of Colonic Transit in Humans
Colonic transit is routinely used to assess colonic motor functions in clinical practice. While transit can be assessed using a radioopaque marker technique or scintigraphy, wireless pH-pressure capsules are increasingly used. Scintigraphy and radioopaque marker techniques entail similar total body radiation exposure, that is, 0.08 rad for the radioactive capsule and for each abdominal radiograph. Colonic transit measured by these three methods is reasonably comparable. However, transit through the ascending and transverse colon was considerably shorter when measured by the radiomarker technique than scintigraphy, probably because particle size influences regional colonic transit.
23.5.3.1
Radioopaque Markers
There are several techniques to assess colonic transit by radioopaque markers. In the Hinton technique, a capsule containing 24 radioopaque markers is given on day 1 and the remaining markers seen on a plain abdominal X-ray on day 6 are counted: fewer than five markers remaining in the colon is normal, more than five markers indicates slow transit. Alternatively overall and regional colonic transit can be assessed by giving a capsule containing 24 radioopaque markers on days 1, 2, and 3 and counting the remaining markers in the abdomen on a plain abdominal X-ray on days 4 and 7. With this technique, a total of fewer than 68 markers remaining in the colon are considered normal while more than 68 markers indicate slow transit.
23.5.3.2
Scintigraphy
Colonic transit can also be assessed by scintigraphy ( Fig. 23.5 ). To avoid dispersion of the radiolabel during passage through the GI tract, the isotope is delivered into the colon by orocecal intubation or via a delayed-release capsule, which contains activated charcoal or polystrene pellets radiolabeled with 99m Tc or 111 In and covered with a single coating of pH-sensitive methacrylate. The capsule dissolves at a pH between 7.2 and 7.4, generally within the distal ileum, releasing the radioisotope within the ascending colon. The colonic distribution of radioisotope on scans taken 4, 24, and 48 h after administration of the capsule is highly sensitive and specific for rapid or slow colonic transit. The proportion of counts in ascending, transverse, descending and rectosigmoid colon, together with stool, is multiplied by a weighting which ranges from 1 (for the ascending colon) to 5 (for stool), respectively. The sum of these products provides the geometric center of overall colonic transit. A low geometric center implies that most radiolabel is close to the cecum while a high geometric center implies that most radiolabel is close to stool.

23.5.3.3
pH-Pressure Capsule
A capsule that measures pH, pressure, and temperature within the GI tract is ingested. The pH rises abruptly when the capsule exits the stomach and drops rapidly when it crosses the ileocecal junction. In a large study, overall agreement between capsule and radioopaque markers of colonic transit was 87%. While the capsule can also assess gastric emptying and small intestinal transit, its sensitivity for detecting delayed gastric emptying (i.e., gastroparesis), as defined by gastric emptying measured by scintigraphy at 4 h, is limited (i.e., 44%).
23.5.4
Assessment of Colonic Motor Activity by Intraluminal Techniques
23.5.4.1
Barostat or Sinusoidal Oscillation
Smooth muscle activity at one or a few points in the colon can be recorded by a balloon controlled by a barostat. Combined barostat-manometry recordings can also be performed ( Fig. 23.6 ). Barostats use an infinitely compliant polyethylene balloon, continuously opposed to the colonic mucosa and can accurately identify colonic contraction and relaxation in that region. The barostat is a rigid piston within a cylinder that can adjust either the pressure or volume within the bag using a servo-mechanism. When the balloon is inflated to a low constant pressure, contraction is accompanied by expulsion of air from the balloon into the barostat. Conversely, when the colon relaxes, the balloon volume increases to maintain a constant pressure. It has been suggested that a barostat-driven balloon is more sensitive than manometric catheters for recording nonocclusive contractions, particularly when the colonic diameter is more than 5.6 cm. Measurements of colonic tone are less variable than pressure waves recorded by manometry. A barostat can also record colonic relaxation, whereas manometry measures only positive-going pressure waves. Changes in colonic tone and phasic activity do not always coincide. Thus, CCK-8 and the selective serotonin reuptake inhibitor, citalopram, inhibited tone but increased phasic activity in the distal colon. Megacolon may be associated with reduced tonic but preserved phasic responses to a meal. Barostats record baseline volume and phasic fluctuations, colonic relaxation, colonic pressure-volume relationships, and perception of distention at the site of the balloon. Regional and age-related differences in biomechanical properties of the colon can thus be detected. Static biomechanical mechanical properties, reflecting muscular and passive elastic components, can be measured after phasic contractile responses to distention have subsided or been blocked pharmacologically with antimuscarinics. Dynamic properties include the phasic muscular response, which may be exaggerated when the rectum is distended rapidly.

Sinusoidal oscillation provides an alternative approach to evaluate the colonic motor responses. This technique has been extensively used to understand airway function and mechanisms of bronchoconstriction in asthma. A colonic (or rectal) balloon is sinusoidally oscillated with small volumes (e.g., 25 mL) at varying frequencies (e.g., 5, 10, or 20 cpm) and durations (typically 20 min). The associated colonic pressure waveform can be decomposed into components in phase with balloon volume (i.e., elastance) and flow (i.e., resistance). This technique has shown that the colonic contractile response to distention is influenced by the distention rate.
23.5.4.2
Colonic Manometry
Stationary or ambulatory manometry can be performed with water-perfused, solid state, or fiber-optic sensors. Current ambulatory recorders can record pressure activity at 6–8 sites, occasionally 16 sites. Sensors are usually spaced at 7.5–20 cm intervals, allowing simultaneous recordings from much of the colon. Recently, fiber-optic manometric catheters with 72, and potentially up to 144 sensors at more closely spaced (1 cm) intervals, have highlighted patterns of colonic motility, particularly propagating contractions, that were not apparent in lower resolution recording. Recordings with 1 cm spacing were subsampled by dropping out sensors, showing that the number of propagating responses is greatly underestimated when sensor spacing is greater than 2 cm, and the direction of propagation is frequently misinterpreted.
Whereas barostat recordings are ideal for detailed examination of smooth muscle activity at a single site, manometry is the gold standard for identifying coordinated patterns of colonic motility measured at multiple sites simultaneously. However, intraluminal pressure changes have to be interpreted carefully. An increase in pressure does not necessarily reflect a contraction of the colonic smooth muscle at that site. Manometric recording devices require positioning using flexible colonoscopy, or per-oral or per-nasal intubation techniques, which are technically demanding. Furthermore, the rectosigmoid and sometimes the entire colon are cleansed to facilitate placement. Such preparation does not affect the overall frequency of propagating sequences or colonic responses to meals or waking, but high-amplitude propagating sequences are more frequent after bowel cleansing, and the spatiotemporal relationships between consecutive propagating sequences are altered.
It is not widely recognized that, in addition to the force of muscle contraction, intraluminal pressures recorded by manometry sensors are influenced by the amount of colonic filling, viscosity of luminal contents, and rate of contraction. Manometry can record nonoccluding contractions of the rabbit colon that cause intraluminal pressure changes. Contractions of the colon wall do not produce large pressure changes unless they are opposed by either downstream occlusion or by the viscosity of propelled content. Muscle contraction may cause significant constriction (isotonic contraction) but little pressure change if content is readily propelled into a dilated downstream segment, against minimal resistance. In contrast, if the same contraction acts against incompressible content, where propulsion is blocked by a nearby occlusion, it will generate considerable pressure but little constriction (isometric contraction). Thus, a nonpropagating pressure wave may reflect highly localized activation of smooth muscle nearby and/or resistance to propulsion of content. Most contractions of the colonic wall have components of both shortening and force generation (“auxotonic” contractions) depending on the resistance that they work against. However, when contractions completely occlude the lumen, the gut wall itself provides considerable resistance to further shortening. Hence, lumen-occlusive contractions can generate huge forces, recorded as local high pressures. Despite this mechanical complexity, multilumen manometry is still the technique of choice for characterizing human colonic motility patterns. A barostat provides more readily interpreted data about regional contraction at one location but cannot evaluate spatial patterns or propagation. Ideally, manometric studies would be based on 24 h recording periods in which rare events can be detected and should ideally be carried out in the unprepared bowel. In reality, shorter periods of recording, in a prepared bowel, have been very informative, bearing in mind the caveats listed above.
23.6
Colonic Motor Patterns
23.6.1
Regulation of Colonic Filling: The Ileocecal Junction
In humans, the ileocecal junction regulates colonic filling and prevents colo-ileal reflux, thereby avoiding contamination of the small bowel by colonic bacteria. This barrier is maintained by a specialized band of muscle at the ileocecal junction and angulation of the ileum on the cecum; the latter is maintained by external (superior and inferior ileocecal) ligaments. Gross observations of intestinal stomas in the early twentieth century showed that chyme was expelled intermittently and forcefully from the ileum, suggesting that the terminal ileum could develop powerful propulsive forces coordinated with ileocecal motility. The human ileocecal sphincter was first studied quantitatively by Cohen and his colleagues in patients who had their colons defunctionalized surgically to treat portosystemic encephalopathy. By performing “pull-throughs” across the sphincter, they confirmed prior findings in dogs, observing a mean tonic pressure of 20 cm H 2 O recorded over 4 cm. This basal pressure was augmented by cecal distention and reduced by ileal distention. While tonic pressures of 20–30 cm H 2 O have been recorded in the ileocecal sphincter (ICS) in dogs, the human ICS generates predominantly during phasic events ; when phasic waves are absent, resting pressure is less than 20 mmHg. Nonetheless, this low tone is sufficient to prevent colo-ileal reflux when the cecum is quiescent. Generally reflux is relatively infrequent, preceded by ascending colonic motor activity or flow, and is often followed by ileal clearing mechanisms. At least part of the junction’s barrier function is attributable to an augmentation of tone during caecal distension.
Phase III of the interdigestive motor cycle [or migrating myoelectric/motor complex (MMC)], a motor pattern that occurs every 90–120 min in the small intestine during fasting, does not contribute to ileocecal transit, because it rarely reaches the terminal ileum in humans and pigs, unlike dogs and other species. Rather, distal ileal motor activity includes propagated contractions, which are single, prolonged (i.e., longer than ileal slow waves; sometimes exceeding 30 s in duration), propagated, and sometimes high amplitude (above 100 mmHg). “Discrete clustered contractions” are rhythmic (i.e., multiphasic and repetitive) bursts of phasic contractions of shorter duration (less than 3 min) that may propagate. They have been recorded in isolated tubular specimens of human ileum ex vivo. Significantly, more prolonged propagating contractions (46%) than discrete clustered contractions (15%) are associated with cecal propagating sequences. It is unclear if this functional coupling between distal ileum and caecum is due to continuity of neuronal pathways or is due to cecal filling induced by a ileal propulsion of content. Ninety three percent of propagating pressure waves in the caecum were temporally associated with episodes of cecal filling; suggesting that episodic cecal filling is one of the triggers for proximal colonic propagating contractions. The rate of delivery of liquids into the proximal colon can influence colonic transit. Thus, a liquid marker injected directly into the proximal colon is emptied more rapidly than after oral ingestion of the same marker.
In the fasted state, cecal filling is slow and erratic, and ileal chyme is retained in the distal ileum for prolonged periods. Most ileal chyme, driven by ileal propagating contractions in synchrony with inhibition of phasic contractions of the ICJ, enters the cecum in a pulsatile fashion within 90 min of a meal. Within 6 months of a right hemicolectomy, isotope movement from the small to the large bowel normalizes in response to the augmented storage capacity in the residual transverse and descending colon.
23.6.2
The Colon as a Storage Organ
Under basal conditions, the healthy colon receives approximately 1500 mL of chyme over 24 h, absorbing all but 100 mL of fluid and 1 mEq of sodium and chloride, which are lost in the feces. Colonic absorptive capacity can increase to 5–6 L and 800–1000 mEq of sodium and chloride daily when challenged by larger fluid loads entering the cecum, as long as there is a slow infusion rate, that is,. 1–2 mL/min. Since the work of Cannon (1902), the proximal colon has been recognized to be the primary site responsible for storage, mixing, and absorption of water and electrolytes. While the rectosigmoid colon functions primarily as a conduit, it can also participate in this compensatory absorptive response. For 25 years, secretory and absorptive processes were believed to be segregated to crypt and surface epithelial cells, respectively. It is now recognized that absorptive mechanisms are constitutively expressed in crypt epithelial cells; secretion is regulated by one or more neurohumoral agonists released from lamina propria cells, including myofibroblasts. When the colon is perfused with a plasma-like solution, water, sodium, and chloride are absorbed while potassium and bicarbonate are secreted into the lumen. Absorption of sodium and secretion of bicarbonate in the colon are active processes, occurring against an electrochemical gradient. There are several different active (transcellular) processes for absorbing sodium, and these show considerable segmental heterogeneity in the human colon. The regional differentiation of colonic mucosal absorption is also demonstrated by regional effects of glucocorticoids and mineralocorticoids on sodium and water fluxes. For example, in the distal colon, epithelial Na + , K + , ATPase is activated by mineralocorticoids. On the other hand, the Na + /H + exchange is activated in proximal colonic epithelium by the mineralocorticoid, aldosterone. Specific channels are involved in water transport across surfaces and epithelia. These water channels, or aquaporins (AQP), are a diverse family of proteins, of which AQP 8 is expressed preferentially in colonic epithelium and small intestinal villus tip cells.
Potassium is absorbed and secreted by active processes; it is unclear if chloride is absorbed by an active process. In contrast to the small intestine, glucose and amino acids are not absorbed in the colon. Colonic conservation of sodium is vital to fluid and electrolyte balance, particularly during dehydration, when it is enhanced by aldosterone. Patients with ileostomies are susceptible to dehydration, particularly when placed on a low sodium diet or during an intercurrent illness. In addition to glucocorticoids and mineralocorticoids (aldosterone), other factors enhancing active sodium transport include somatostatin, α 2 adrenergic agents, and short-chain fatty acids. Clonidine mimics the effects of adrenergic innervation by stimulating α 2 receptors on colonocytes. In contrast, stimulation of mucosal muscarinic cholinergic receptors inhibits active NaCl absorption and stimulates active chloride secretion.
23.6.3
Colonic Motor Patterns In Vivo
Older studies with nonhigh-resolution catheters in which sensors were separated by 10–15 cm suggested that nonpropagated phasic activity was the predominant motor pattern. More recent high-resolution recordings have called this into question, suggesting that propagating phasic activity constitutes the majority of colonic motor activity in humans. This account is based, to a great extent, on data obtained from high-resolution manometry studies, supplemented by lower resolution studies where appropriate.
23.6.4
High-Amplitude Propagating Contractions
Early manometric recordings, with widely spaced sensors, identified prominent high-amplitude contractions that propagated over many centimetres aborally—these came to be known as high-amplitude propagating contractions or sequences (HAPCs or HAPS). These occur with increased frequency after meals, after wakening, prior to defecation but are decreased at night. The definition of HAPCs varies between laboratories, with cutoffs ranging from 50 to 116 mmHg ( Fig. 23.7 ). HAPCs occur an average of 6 times per day and rarely propagate retrogradely. They have been shown to be the manometric equivalents of the highly propulsive “mass movements” of content described radiographically decades earlier. HAPCs typically start in the proximal colon and propagate distally, but few actually reach the rectum. The natural trigger for HAPC initiation is unclear but may involve distension. HAPCs can also be reliably initiated by luminal application of the stimulant laxative bisacodyl—a mechanism that is blocked by lidocaine and hence is dependent on activation of neural pathways. They can also be triggered by the parenteral administration of cholinesterase inhibitor neostigmine or by intraluminal stimuli, that is, glycerol or and oleic acid. They require the gut wall to be intact and are not mediated by either hormonal or extrinsic neural connections. Indeed, in a dog model, HAPCs occur more frequently after extrinsic denervation. More frequent HAPCs have been implicated to cause diarrhea in humans with idiopathic autonomic neuropathy and diarrhea. Conversely, adult patients with slow transit constipation show reduced frequencies of HAPCs.

Propulsive HAPCs that lead to defecation do not just occur out of the blue. Propagating sequences start in the proximal colon up to an hour before defecation. The great majority of these propagate in an anterograde manner. The few retrograde propagating sequences were almost exclusively confined to the ascending colon, propagated over shorter distances than antegrade propagating sequences. Anterograde HAPCs occur in a distinct, linked order in healthy subjects. Typically, the second HAPC starts more proximally than the first one in a burst. The third HAPC starts even more proximally. This ensures that sequences break off and propel material from the distal end of a column of fecal matter. While most single propagating sequences do not span the length the colon, collectively a series of linked propagating sequences can. The mechanisms underlying regional linkage are yet to be determined. This tight linkage is disrupted in patients with slow transit constipation.
Despite their visual prominence, and the corresponding attention that they have received from researchers, HAPCs only account for a small minority (1.4%) of propagating motor patterns recorded with high-resolution manometry in healthy human subjects. Several other distinctive patterns of colonic motility have been distinguished.
23.6.5
Cyclic Motor Patterns
Cyclic motor patterns are the major form of propagating motor activity in the human colon, accounting for 69% of propagating events. These events occur repetitively at 2–6/min in bursts, and propagate either orally or aborally at similar velocities. They are typically of lower amplitude than HAPCs. The abundance of these events was greatly underestimated in earlier low-resolution manometry studies, probably because their average extent of propagation is quite short (less than 5 cm). They are especially concentrated in the sigmoid colon and often occur in extended bursts, lasting from 3 to 30 min. Similar activity has been noted in a number of previous studies and been called variously “periodic colonic motor activity,” “periodic rectal motor activity” and the “rectal motor complex.” A number of lines of evidence suggest that this activity acts functionally as a “rectosigmoid brake,” restraining the propulsion of content into the rectum to limit challenges to continence. Rectal motor complexes are often associated with motor activity more proximally or by the arrival of stool or gas from the upstream colon. This is compatible with the suggestions that rectal motor complex provide a “braking mechanism” to keep the rectum empty. Consistent with this, rectosigmoid cyclical motor patterns are more abundant at night.
The mechanisms that underlie cyclic motor patterns have not been positively identified; however, it is likely that slow waves contribute to setting their frequency. Slow waves in human colon typically occur at 2–4/min. It is feasible that the upper range of cyclic motor patterns (up to 6 Hz) may reflect interactions between several pacemaker regions. However, cyclical activity in the rectosigmoid is likely to involve a neuronal component too as the activity increases rapidly after a meal; well before content could reach the colon. Thus, retrograde events increased from 5.6 to 34.7/h within minutes of eating, but anterograde cylic events did not change significantly.
23.6.6
Single Motor Patterns
Three other patterns that are probably related to cyclical motor patterns were distinguished by high-resolution manometry in normal adults. These consisted of similar types of contractions (in terms of amplitude and duration) to cyclical motor patterns, but occurred singly (i.e., not in clusters). These events were divided into short single motor patterns (anterograde or retrograde), which accounted for nearly one quarter of propagating motor patterns. Long single motor patterns originated in the proximal colon and only migrated anterogradely. Unbiased discriminant and cluster analyses indicated that all of these three single motor patterns were similar to one another and distinct from HAPCs. The rarest colonic motor patterns were single, slowly propagating retrograde contractions that migrated over 40 cm up the colon after starting in the sigmoid colon. Little is known about their significance.
23.6.7
Pan-Colonic Pressurizations
An additional motor pattern in the colon has been recently described. It occurs nearly simultaneously over considerable lengths of the colon and was first called “pan-colonic pressurizations” ; similar patterns have been recorded by a second group. These events were very frequent, with 17–32 events/h and may have been excluded in earlier recordings as breathing or movement artifacts. They are of relatively low amplitude; from 12 to 15 mmHg and increase in frequency on eating. Convincingly, they are associated with relaxations of the internal anal sphincter and a desire to pass flatus. They resemble esophageal common cavity waves and are not associated with abdominal wall straining. This motor pattern may function to propel gas rapidly along the colon. It is not clear whether these events should be classified as “nonpropagating” or “rapidly propagating” as they occur nearly simultaneously along the considerable lengths (33 cm average) of the colon. How contractions can be so tightly coordinated over considerable lengths of bowel is a matter for speculation. The most likely explanation is that there are bursts of action potentials in chains of interneurons connected by fast synaptic contacts. With a high safety factor, estimates of conduction velocity and synaptic delay suggest that this mechanism could activate motor neurons at many points over a distance of up to 0.5 m with a lag of less than 1 s. Whether such lags occur and can be detected in manometric recordings remains to be seen.
23.6.8
ex vivo Studies of Colonic Motility
While manometric recordings from conscious human subjects are the optimal way to study colonic motor patterns, simpler isolated preparations, studied ex vivo, can be invaluable for analyzing the mechanisms that contribute to them. In isolated strips of human colon, activity at three different frequencies is distinguishable. High-frequency (approximately 15–20 cpm) small-amplitude electrical events are generated near the myenteric border by ICC MY ; they can cause small perturbations of tension in this frequency range. Intermediate-frequency (approximately 2–4 cpm) small amplitude contractions occur at frequencies similar to slow waves generated by ICC SM at the submucous border. Low-frequency events (approximately 0.3–1 cpm) of high amplitude are also seen and may arise near the MP.
ex vivo studies of the isolated whole human colon demonstrate two major types of contractions. In one study, cyclic motor complexes, (CMCs) were recorded that propagated either orally or aborally over distances of 40 or more centimeters. In another, short single motor patterns were recorded, using higher resolution recordings. Both can be initiated by enteric nerve stimuli or luminal distension at any location and propagate over considerable distances in either anterograde and/or retrograde directions. How these relate to the motor patterns recorded in vivo is not currently clear.
Studies of animal tissue, ex vivo, have also revealed many details of how neurogenic and myogenic components interact to give rise to patterned colonic motility. When pellets were inserted into the oral end of an isolated preparation of guinea pig distal colon, they were spontaneously propelled aborally. Comparable localized distension evoked an ascending contractile reflex and descending inhibition, similar to that described by Bayliss and Starling as the “law of the intestine.” Both polarized reflexes required continuity of the MP and were blocked by tetrodotoxin. An additional “occult” reflex has been described in which colonic elongation, comparable to that evoked by a group of pellets, inhibits propulsive activity, particularly aborally. When pellets cluster, the most aboral pellet is typically propelled first, followed, one at a time by the remaining pellets. In addition, the rate of propulsion adapts to the size and shape of the pellet. Thus, peristaltic propulsion of pellets is an intrinsic motor pattern, triggered by content, rather than just a sequential activation of simple polarized reflexes. Another motor pattern seen ex vivo is the “colonic migrating motor complex,” which has been extensively studied in the mouse colon. It involves activation of both excitatory and inhibitory motor pathways to the circular muscle layer from myenteric neurons and shows complex pharmacological modulation. Similar patterns occur in the rabbit colon which also propels pellets. This pattern has some resemblance to the colonic motor complexes (CMC) recorded in the human colon ex vivo. In addition, low-amplitude cyclic contractions that are believed to be driven by slow waves have been recorded in several isolated preparations of animal colon. These are the equivalents of the cyclical motor programs recorded in vivo in healthy human colon.
One technical advance for quantitative analysis of motility patterns has been the development of video-based spatiotemporal mapping, which plots a time series of contractile patterns over seconds to minutes as two-dimensional maps. Contractions and relaxations appear as bands in different colors or shades. At present, these have mostly been used on isolated tubular specimens of tissue ex vivo, but they can be combined with other recording techniques such as manometry. Unsurprisingly, movements of the colonic wall are closely, but not simply related to changes in intraluminal pressure. As expected, “common cavities,” where considerable lengths of colon form a single compartment, were associated with rapid propulsion despite very low-pressure gradients. This explains why high-amplitude propagating contractions are not the only propulsive patterns in the colon. A detailed analysis of rabbit colonic motility using spatiotemporal maps identified four motility patterns, that is, peristaltic CMMCs, lower amplitude contractions propagating orally or anally (“ripples”) that likely to correspond to slow-wave related events, rapid phasic contractions that probably involve longitudinal muscle, and deep haustral contractions that moved slowly under neural control. Comparable patterns were also described in the guinea pig colon in vitro using similar techniques. Identifying the manometric equivalents of each of these events (and any other colonic motor patterns) will be very informative for interpretation of human colonic manometry traces.
23.6.9
Relationships Between Colonic Motor Patterns and Propulsion
“Mass movements,” first detected radiographically, are movement of stool over long distances, sometimes associated with HAPCs. More frequently, movement of colonic content occurs in a stepwise manner over short distances, in both antegrade and retrograde directions, associated with propagating sequences. Early human studies, which evaluated the relationship between colonic motor events and transit with slow scintigraphic acquisition rates (e.g., 1 min frames to 1 min frames every 5 min ) may have missed some movements. More recent studies have utilized faster image acquisition rates (i.e., 1 image every 15 s) with visual detection or quantitative assessment of colonic isotope movement. In humans, 93% of propagated contractions were associated with propulsion of content. Low-amplitude propagating sequences with amplitudes between 2 and 5 mmHg were as likely to be associated with movement of content as higher amplitude propagating sequences. However, of all episodes of propulsion, fewer than half were associated with detectable propagating sequences, suggesting that pressure waves are not essential for propulsion. The strength of this pressure-flow relationship is region-dependent, being stronger in the transverse than in the cecum and ascending colon. In theory, the movement of colonic contents that are not accompanied by propagating colonic contractions may be explained by contractions at points remote from the recording sites, or motor events that do not affect intraluminal pressure significantly, such as longitudinal muscle shortening, nonlumen-occluding circular muscle contractions, or alterations in regional wall tone. Retrograde sequences are generally associated with retrograde movements of colonic content. About half of the retrograde contractions follow immediately after an antegrade movement, indicating frequent “reflux” of content back into the region from which it had just moved. This subtle “to and fro” motion is likely to help maintain maximal absorption, retard colonic transit, and therefore reduce stool frequency.
Since there are no physiological or pharmacological perturbations which selectively modulate colonic tone (i.e., without affecting phasic contractions), the contribution of colonic tone to propulsion is unclear. Emptying of the proximal colon occurs more rapidly when wall tone is increased (e.g., by intraluminal fatty acids) than when the tone is low; the volume and consistency of the contents also affect the rate of emptying.
In summary, outside of the immediate predefecatory phase (see below), propagating sequences in the distal colon are less frequent but propagate for shorter distances, at a faster conduction velocity with a lower likelihood of propelling contents than the proximal colon. Conversely, a larger proportion of nonpropagating (segmenting) pressure waves occur in the distal than proximal colon. Considered together, these features would be expected to result in retardation of flow into the distal sigmoid and rectum, thus minimizing challenges to continence while maximizing the mixing of content more proximally.
23.6.10
Physiologic Modulators of Colonic Motility
23.6.10.1
Diurnal Variation in Colonic Motility
With the exception of rectal motor complexes, colonic myoelectric and phasic pressure activity is profoundly suppressed at night. However, propagating and nonpropagating pressure activity increase promptly during shifts to a lighter level of sleep and upon awakening; which likely explains the desire to defecate.
23.6.10.2
Colonic Contractile Response to a Meal
In contrast to the canine colon, contractile activity in the human colon is not cyclical. Eating is accompanied by increased tone throughout the colon, albeit less pronounced in the proximal than distal colon ( Fig. 23.8 ). Eating also increases colonic phasic pressure activity. Since this response is preserved even after a gastrectomy, the term “colonic motor response to eating” is preferable to “gastrocolonic reflex.” The response may begin within a few seconds after eating and lasts up to 2½ h. A biphasic response with early (first 60 min) and late (120 and 150 min) components have also been described. Meal composition and caloric content are both important; a mixed meal containing more than 500 kcal predictably elicits a response. Gastric distention and chemical stimulation by nutrients elicit comparable responses; lipids are the most potent stimuli, while amino acids may inhibit it. In addition to augmenting tonic and phasic motor activity, including sigmoid colon retrograde cyclical patterns, feeding also increases colonic sensation and affects rectocolonic reflexes. This reflex may contribute to the postprandial urge to defecate and to postprandial abdominal discomfort in IBS.

Neural and hormonal mechanisms have been implicated in this response; the precise mechanisms are uncertain. It is conceivable that different mechanisms regulate the early and late components. The early, particularly the immediate, component is likely to be neurally mediated. Indeed, extrinsic denervation abolished this response in dogs and efferent vagal fibers contribute to this response in primates. The later component temporally coincides with arrival of chyme into the ileum and may be influenced by humoral factors such as peptide YY, neuropeptide Y, and neurotensin released from the ileal mucosa. Although serum levels of gastrin and cholecystokinin rise after a meal, intravenous cholecystokinin reduces colonic tone. Moreover, the CCK-A antagonist loxiglumide blocks the effects of CCK on the colon but does not abolish the colonic response to eating, thus making CCK an unlikely mediator. Atropine, naloxone and the 5-HT 3 antagonist ondansetron inhibit it, indicating that cholinergic, opiate, and serotoninergic 5-HT 3 receptors may be involved.
23.6.10.3
Effects of Stress on Colonic Sensorimotor Functions
Acute stress alters GI sensorimotor functions in healthy people and in IBS. However, stress has different effects on upper and lower GI motility. While stress paradigms (e.g., cold pain, labyrinthine simulation, psychological stress), all inhibit gastric emptying and postprandial upper GI motor activity, acute physical stress (e.g., hand immersion in cold water), and psychological stress (e.g., dichotomous listening and mental arithmetic) stimulate contractile activity and propulsive colonic motor function in healthy people. Moreover, an exaggerated colonic motor response to psychological and physical stressors has been reported in IBS, compared to healthy subjects. In addition, manipulation of attention and changes in arousal level produced by stress, distraction and relaxation also alter visceral perception. For example, acute mental stress increased anxiety and perception of distension of the transverse and sigmoid colon. In contrast, mental relaxation reduced perception during sigmoid colonic distension. The effects of mental stress on sensation vary between studies, likely in part because of differences in stress provoking techniques and the degree of distraction associated with stress. For example, stress reduced rectal sensory thresholds in healthy subjects but not in IBS patients, perhaps because healthy subjects but not IBS patients were distracted by the stressful stimulus. However, these studies all evaluated the effects of acute stress; the effects of chronic stress on colonic sensation are unknown.
In animal studies, the effects of stress on GI motility are substantially mediated in the central nervous system by CRF. These effects are mimicked by centrally applied corticotrophin-releasing factor (CRF), its related peptides, urocortin 1 or agonists at CRF 1 receptors (but not CRF 2 receptors). Central CRF1 receptor antagonists reduce CRF and stress-induced increased colonic motility. While CRF 1 receptors are predominantly expressed in the brain, and CRF 2 receptors are mainly expressed in the periphery, CRF 1 receptors are also located on myenteric neurons. While many effects of stress in the body are mediated by CRF activating the hypothalamic-pituitary-adrenal axis, this may not be the case for changes in distal bowel motility. There is also evidence that central activation of CRF1 receptors leads to activation of parasympathetic cholinergic efferent pathways to the large bowel via both vagal and sacral pathways. It has also become clear that peripheral CRF and its relations may play a role in stress effects on the gut. CRF is expressed by cells in the colon wall, including several types of enteric neurons as well as enterochromaffin cells and some immune cells in the mucosal parenchyma. Urocortin 1 is also located in enteric neurons. Peripherally administered CRF or its agonists mimic stress responses similar to centrally administered CRF via CRF1 receptors.
The mechanisms by which stress modulate colonic sensorimotor functions in humans are unclear. Exogenous CRF exaggerated colonic motility and abdominal pain in IBS patients and two studies have evaluated the effects of CRF antagonists in humans. In the first, a nonselective CRF receptor antagonist (i.e., [alpha]-helical CRH) administered intravenously prevented the increase in sigmoid motility induced by rectal electrical stimulation in IBS but did not influence sensory thresholds during electrical stimulation. Because α-helical CRH is a nonspecific antagonist, albeit with higher affinity for CRF 2 than CRF 1 receptors, it is unclear whether increased sigmoid motility was mediated by CRF 1 or CRF 2 receptors. In the second study, a therapeutic dose of pexacerfont, which is a CRF1 antagonist, did not significantly affect GI or colonic transit or bowel symptoms in diarrhea-predominant IBS. These findings suggest that in contrast to a rat model of stress-stimulated colonic motility, this CRF 1 antagonist does not affect colonic motility, as measured by colonic transit, in patients with IBS. However, it is conceivable that the stress levels in these patients, while representative of IBS patients in general, were not sufficient to activate stress-induced alterations in colonic motility. While pexacerfont crosses the blood brain barrier in animals, it is unclear whether this is the case in humans. Similarly, it is unclear if α-helical CRH crosses the blood brain barrier in humans. Hence, further studies will be necessary to elucidate the mechanisms by which stress affects colonic sensorimotor functions in health and IBS.
23.6.10.4
Effects of Physical Exercise on Colonic Sensorimotor Functions
Total, propagated, and nonpropagated colonic phasic pressure activity decline during physical exercise in healthy people. Conceivably, these changes are mediated by exercise-induced increased sympathetic tone. After exercise, the number and amplitude of propagated waves increased but simultaneous waves and cyclical waves did not. While increased physical activity is associated with less constipation, increased intestinal gas clearance, and reduced bloating, the effects of increased physical activity on bowel habits and colonic transit vary among studies; physical exercise either accelerated or did not affect colonic transit.
23.6.10.5
Colonic Relaxation
Colonic phasic pressure activity and tone decline during sleep, except for cyclical motor patterns in the sigmoid colon and rectum. Motor activity also decreases during balloon distention of the rectum and in response to a number of parenterally administered pharmacological agents. The α 2 adrenergic agonist clonidine, morphine, atropine, the 5-HT 1a agonist, buspirone, and the 5-HT 1D agonist, sumatriptan all reduce colonic tone in humans. This propensity for colonic relaxation, particularly that induced by sympathetic stimulation and opiates, may contribute to the pathophysiology of acute colonic dilatation or pseudo-obstruction.
23.6.11
Colo-Colonic Reflexes
Rectal distention by a balloon to subnoxious levels induces colonic relaxation in humans and dogs. During postprandial but not fasting conditions, colonic relaxation during rectal distention is less pronounced in IBS. Conversely, colonic distention increases rectal tone in health ; this reflex is attenuated in female patients with IBS
Colo-colonic reflexes are likely mediated by nervous pathways through the prevertebral ganglia, which are independent of central nervous system activity and have been well characterized in animal preparations. Pathways probably involve collateral axons of spinal sensory neurons that contact sympathetic neurons in prevertebral ganglia and projections of mechanosensitive enteric viscerofugal neurons that also excite sympathetic neurons projecting to the gut. Colonic relaxation induced by rectal distention may explain left-sided colonic transit delays in patients with obstructed defecation since restoration of normal defecation tends to improve colonic motility. Similar reflexes likely operate between the colon and proximal GI tract and perhaps partly explain GI motor disturbances in slow transit constipation as detailed below.
23.7
In Vitro Studies of Colonic Motility
While the primary aim of this chapter is to summarize current understanding of aspects of human colonic motility, studies in laboratory animals have contributed much to our understanding. As discussed above, investigations of human motility are often limited by the techniques that are available for use in live human subjects. In animal preparations, other powerful methods, under tightly controlled conditions, can be applied, particularly in vitro, providing important insights that may be relevant to understanding human motility.
When pellets are inserted into the oral end of an isolated, in vitro guinea pig distal colon, these pellets are spontaneously propelled aborally. Comparable localized distension of the same preparation leads to an ascending contractile reflex and descending inhibition, similar to that described by Bayliss and Starling as the “law of the intestine.” Both polarized reflexes required continuity of the MP and were blocked by tetrodotoxin. It is intuitive to suggest that the propulsive peristalsis equates to the sequential activation of polarized reflexes by the moving pellet. However, similar aborally directed waves of contraction and relaxation also occurred in the absence of content. Furthermore, with a cluster of pellets, typically the most aboral pellet moves first, followed, one at a time by the remaining pellets. More recently, an additional “occult” reflex has been described wherein colonic elongation, comparable to that evoked by a group of pellets, inhibits propulsive activity, particularly aborally. Thus, it is now clear that propulsion of pellets is mediated by an intrinsic motor pattern, rather than just sequential activation of the reflexes that underlie the “law of the intestine.” This motor pattern may be related to the “colonic migrating motor complex,” which has been extensively studied, especially in the mouse colon. The latter involves activation of both excitatory and inhibitory motor pathways to the circular muscle layer from myenteric neurons and shows complex pharmacological modulation that would be expected for any behavior pattern mediated by interacting neural circuits (e.g., Ref. ). Similar patterns are also seen in the rabbit colon which also propels pellets. Here, it has been shown that peristaltic propulsion involves more than sequential activation of reflexes since velocity of pellet movement is affected by resistance, pellet length, and diameter.
This raises the question whether patterns in isolated preparations in vitro can be used to understand recordings of human colonic motility. One technical advance for quantitative analysis of motility patterns has been the development of spatiotemporal mapping techniques, which plot a time series of contractile patterns over considerable durations as two-dimensional maps. Contractions and relaxations appear as bands in different colors or shades. At present, these are confined to isolated tubular specimens of tissue in vitro, but they can be combined with other recording techniques such as manometry. A recent study has confirmed that many nonpropagating pressure events, recorded manometrically, correspond to localized contractions of the circular muscle. As expected, “common cavities,” where considerable lengths of colon form a single compartment, were associated with rapid propulsion despite very low-pressure gradients. This indicates that high-amplitude propagating contractions are not the only propulsive patterns in the colon. A detailed analysis of rabbit colonic motility using spatiotemporal maps identified four motility patterns, that is, peristaltic CMMCs, lower amplitude contractions propagating orally or anally (“ripples”) that likely to correspond to ` related events, rapid phasic contractions that probably involve longitudinal muscle, and deep haustral contractions that moved slowly under neural control. Comparable patterns were also described in the guinea pig colon in vitro using similar techniques. Identifying the manometric equivalents of each of these events (and any other colonic motor patterns) will be very informative for interpretation of human colonic manometry traces.
23.8
Modulators of Colonic Motility
23.8.1
Pharmacologic Modulators
Table 23.1 summarizes the effects of various neurotransmitters and pharmacological agents, which can modulate colonic sensorimotor functions in humans.
Drug Class | Examples | Rationale or Putative Action | Pharmacodynamic (Intestine or Colon) | Clinical Efficacy: Phase IIB or III Primary Endpoints or FDA Approval Status | Safety Issues/Comments | Ref # |
---|---|---|---|---|---|---|
Muscarinic cholinergic agonist | Bethanechol | Stimulates muscarinic M 2 and M 3 receptors | Increased colonic phasic pressure activity, reduced rectal compliance, and enhanced urgency during rectal distention | |||
Acetylcholinesterase inhibitors | Neostigmine and pyridostigmine | By inhibiting acetylcholinesterase, increases availability of acetylcholine at neurons in myenteric plexus and neuromuscular junction | Similar to bethanechol. Also increases colonic and rectal tone | Neostigmine; IIB; in acute colonic pseudo-obstruction Pyridostigmine; IIB; in diabetes mellitus and constipation | Bradycardia | |
Muscarinic antagonists | Tolterodine, darifenacin, atropine | Muscarinic M3 (darifenacin, solifenacin) or nonspecific antagonist (tolterodine, fesoterodine) | Delayed gastric emptying (fesoterodine), small intestinal transit (darifencain 7.5 mg and 15 mg), and colonic transit (solifenacin) | Urinary hesitancy | ||
Adrenergic agonist | Clonidine | α 2 adrenergic agonist | Reduced colonic tone and phasic pressure activity and colonic perception of distention. Increased colonic compliance | IIB; diarrhea-predominant IBS | Dry mouth, sedation, orthostatic hypotension | |
Yohimbine | α 2 adrenergic antagonist | Increased colonic tone and phasic pressure activity and colonic perception of distention. Reduced colonic compliance | ||||
5-HT 3 – antagonist | Ondansetron | Inhibits gastrocolonic response in health and carcinoid diarrhea | ||||
Alosetron | Slowed colonic transit and increased colonic compliance and the distending volume in IBS | III, reduced abdominal pain in females with nonconstipated IBS and improved stool consistency in males | ||||
Granisetron | Inhibits gastrocolonic response in health. Decreases rectal sensitivity and reduces postprandial rectal motor activity in IBS | . | ||||
5-HT 3 -agonist | DDP-733 | Stimulate intrinsic cholinergic neurons to enhance motility | 4 mg dose delayed fasting migrating motor complexes, accelerated small intestinal transit, and induced softer stools or diarrhea in 15% of subjects | IIB, dose-ranging study in 91 IBS-C patients: 1.4 mg dose associated with significantly greater proportion of responders (subject global assessment of relief) | No known vascular effects | |
5-HT 4 -agonists | Prucalopride | Stimulate intrinsic cholinergic neurons to enhance motility | Increases SB, colon motility and transit in healthy controls and patients with chronic constipation | IIB and III in CC (thousands of pts): BM frequency and satisfaction with bowel function both improved. Approved | Greater selectivity for 5-HT 4 than 5-HT 1B or hERG channel | |
ATI-7505 | Stimulate intrinsic cholinergic neurons to enhance motility | Increases colon transit in healthy controls | None reported | Greater selectivity for 5-HT 4 ; not metabolized by CTP 3A4 | ||
Velusetrag (TD-5108) | Stimulate intrinsic cholinergic neurons to enhance motility | Dose-related increase in SB and colon transit in healthy controls | IIB, dose-ranging study in 401 CC patients increased BM frequency and proportion with adequate relief | Greater selectivity for 5-HT 4 | ||
5-HT 4 -antagonists | SB 207266 (piboserod) | No effect on stomach, small intestinal or colonic transit | ||||
NARI and 5-HT 3 -antagonist | DDP-225 | May increase synaptic levels of norepinephrine to reduce visceral pain; inhibit intrinsic cholinergic neurons | Uninterpretable | IIB, dose-ranging study in 87 IBS patients increased proportion with adequate relief | No constipation reports suggests low expectation for 5-HT 3 antagonist activity | |
Antidepressants | May reduce visceral sensation and relieve depression associated with IBS | SSRIs, fluoxetine and paroxetine, and TCA, amitryptiline, do not reduce visceral sensitivity, in contrast to the SNRI, venlafaxine; SSRI accelerates and TCA slows SB transit | Small studies with SSRI or TCA equivocal; large study had no significant benefit of desipramine over placebo in ITT analysis, but did in per-protocol analysis (completed treatment) | Side effects common with TCA. Post hoc analysis for desipramine showed benefit in those with moderate symptoms, abuse, no depression and IBS-D | ||
NK antagonists | NK 1 antagonist, ezlopitant | NK 1 -receptors’ role in nociception | Reduce the emotional response of IBS patients to rectosigmoid distension | None | ||
NK 2 -antagonist, nepadutant | NK 2 -receptors’ influence on smooth muscle contractility | Inhibited stimulatory effects of neurokinin A on small intestinal motor activity in healthy males | None | |||
NK 3- antagonist, talnetant | NK 3 -receptors’ role in nociception | No effect on rectal compliance, sensory thresholds or intensity ratings in healthy controls | Two IIB trials in 1350 IBS patients: no benefit | |||
Κ-opioid agonist | Asimadoline | κ-Opioid receptors in visceral perception | Reduced fasting colonic tone in healthy subjects and sensation during colon distension in health and IBS | IIB, dose-ranging study, 596 IBS patients: improved pain, urgency, and stool frequency. On-demand dosing not effective in reducing severity of abdominal pain in 100 IBS patients | ||
Peripherally selective μ-opioid receptor antagonist | Methylnaltrexone | Quarternary ammonium compound, antagonizes peripheral but not central effects of opiates | Did not reverse codeine’s effects on small intestinal or colonic transit in opiate-naïve healthy volunteers | Approved for treating opiate-induced constipation in patients with advanced illness receiving palliative care after failing laxative therapy | Gastric or intestinal perforation—seven cases during first 18 months after approval | |
Alvimopan | Peripherally acting μ-opioid receptor antagonist (PAMORA), antagonizes peripheral but not central effects of opiates | Accelerates colonic transit in healthy subjects | Approved for accelerating the time to recovery of gastrointestinal functions in patients undergoing surgical small or large intestinal resection with primary anastomosis but not for opioid-induced bowel dysfunction | Higher incidence of myocardial infarction (1.3% for vs 0% for placebo) and all cardiovascular adverse events (2.6% vs 1.1% for placebo) | ||
Naloxegol | Pegylated derivative of naloxone; does not cross blood-brain barrier. Peripherally acting PAMORA | Antagonizes morphine-induced reduced orocecal transit, with limited effects on miosis or opioid withdrawal symptoms | Approved for treating OIC in adults with chronic noncancer pain | Most common side effects are abdominal pain, diarrhea, nausea, headache, and flatulence. No effects on QT/QT c in clinical trials | ||
2,3-Benzodi-azepine modulator | Dextofisopam | Potential to reduce stimulation-induced colonic motility and visceral sensitivity | None reported | IIB study in 140 IBS patients: increased number of months of adequate overall relief of IBS symptoms; efficacy lower over time | More events of worsening abdominal pain; headaches were more frequent with placebo | |
Nitric oxide antagonist | l -NMMA | Antagonizes inhibitory effects of nitric oxide on colonic motility | A lower dose (3 mg/kg/h) but not a higher dose (6 mg/kg/h) increased propagated and nonpropagated colonic motor activity. In another study, 8 mg/kg/h also increased colonic perception of distention | None | None | |
CCK 1 antagonist | Dexloxiglumide | Competitive antagonist of the CCK 1 -receptor | Slower ascending colon emptying with no significant effect on overall colonic transit | Two initial IIB or III trials: not efficacious in IBS-C; a randomized withdrawal design trial showed longer time to loss of therapeutic response, longer for dexloxiglumide | ||
ClC2 channel activator | Lubiprostone | Increases intestinal water and electrolyte secretion | Accelerates SB and colonic transit in healthy controls | CC and IBS-C | Nausea that is usually mild; diarrhea | |
Guanylate cyclase-C agonist | Linaclotide | Increases intestinal water and electrolyte secretion | Accelerated ascending colonic transit and altered bowel function in women with IBS-C | CC and IBS | Diarrhea | |
Plecanatide | As above | Not evaluated | CC only (not IBS-c) | Diarrhea | ||
Probiotics | Several, e.g., Bifidobacteria , Lactobacillus , Saccharomyces species or combinations | Potential mechanisms: immune, barrier, fermentation | Slow colonic transit in IBS-D | Several IIB studies: efficacy in overall IBS and single symptoms, e.g., flatulence, pain | ||
Antibiotics | Neomycin, metronidazole, rifaximin | Changes in gut microflora may be present in IBS | No consistent reduction in breath hydrogen excretion after lactulose load in those with symptom relief | Rifaximin approved for IBS-D in men and women | Rifaximin—headache |
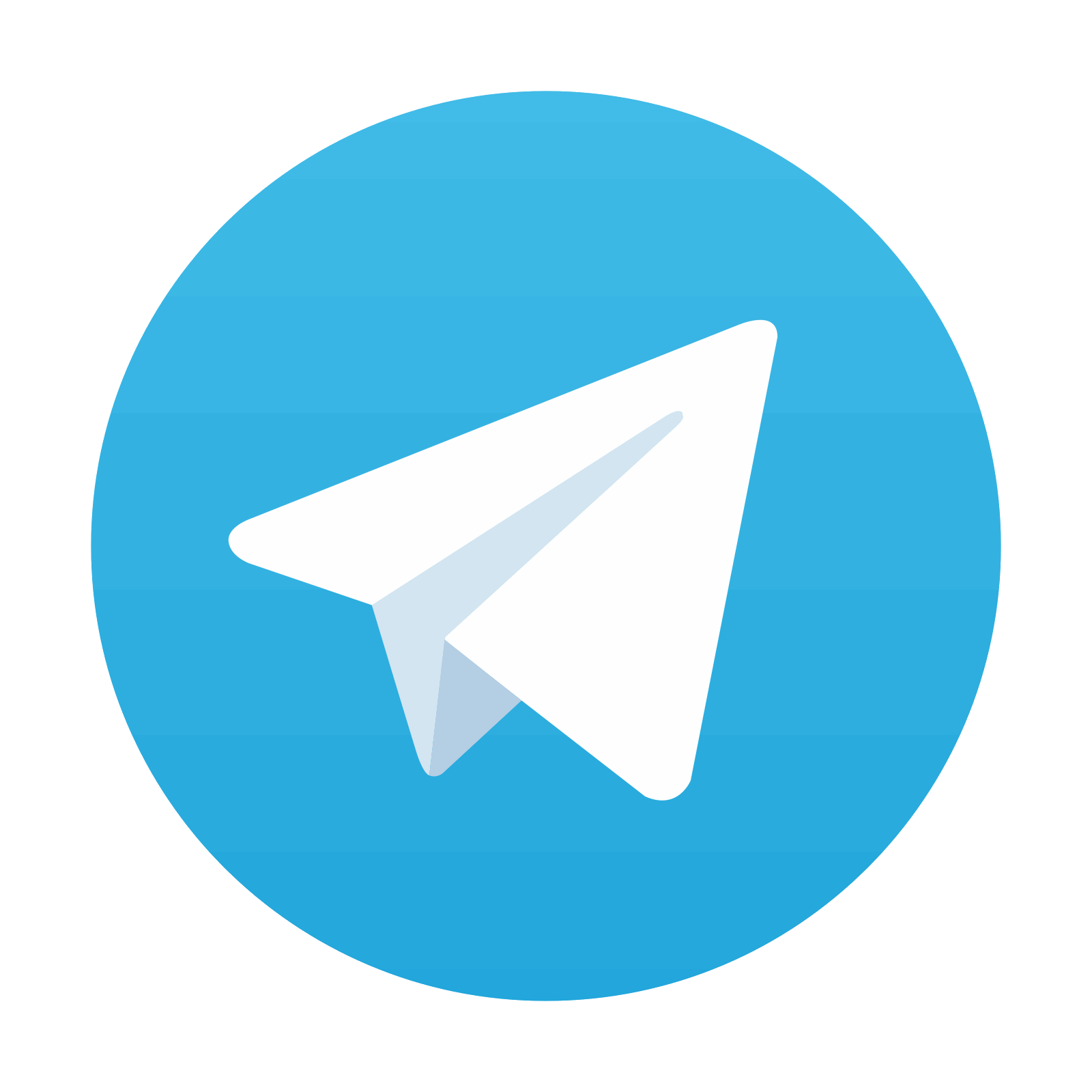
Stay updated, free articles. Join our Telegram channel

Full access? Get Clinical Tree
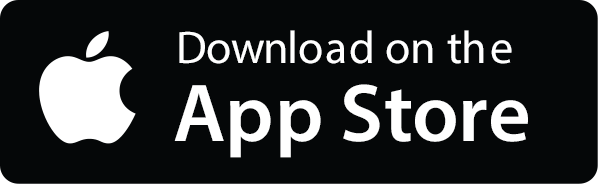
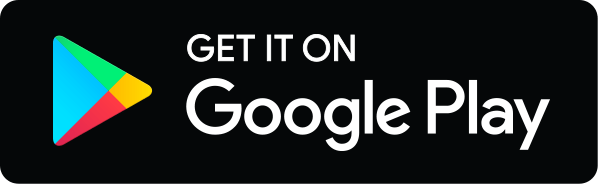
