Overview
The primary role of the gastrointestinal (GI) tract is to facilitate digestion and absorption of nutrients, but the intestines also engage in a multitude of other biologic functions, including significant immunologic and neuroendocrine activities. The intestinal surface is composed of a layer of epithelial cells that comprise villi, which provide a sizeable surface area to facilitate exposures to a vast array of food antigens, bacteria flora, and microbial metabolites.
The neonatal intestine functions during a period of major transition, including alterations in luminal and surface enzyme activities, changes in both the innate and adaptive immune systems, and major shifts in the microbiome, depending on environmental factors, such as diet and other exposures. In addition, premature infants may be still completing basic morphogenesis and development in addition to coping with the changes in their environments. There is new recognition that the neonatal intestine is exposed to microbes in utero and that this likely plays a major role in intestinal development, even before birth and subsequent exposure to the extrauterine milieu. This chapter will provide an overview of the intestinal mechanism that relates to systemic inflammation, the relationship and interactions between the intestinal microbiota and the intestinal mucosa, as well as the resultant effects on the developing nervous system.
Components and Functions of the Gastrointestinal Epithelium
The GI surface is composed of several types of cells. The epithelial layer originates from the endoderm and throughout gestation changes and differentiates through recanalization of the intestinal lumen and, later, through the development of villi and crypts. The adult-type crypt epithelium architecture is present by 30 weeks’ gestational age. Many genes that are necessary for cellular differentiation and migration have been identified ( Table 10.1 , Fig. 10.1 ). The cell types present in the epithelium include enterocytes, goblet cells, enteroendocrine cells, Paneth cells, microfold cells, lymphocytes, and tuft cells ( Table 10.2 ).
Gene | Function |
---|---|
Hox | Gut patterning along the anterior–posterior axis |
Hoxd13 | Controls final epithelial phenotype |
Hoxa13 | Controls final epithelial phenotype |
Cdx2 | Provides positional information for specification of midgut endoderm |
Parahox | Anterior-posterior gut development |
Hedgehog | Directs organogenesis |
BMP | Controls gut muscular development, Epithelial homeostasis |
FGF | Establishing gut tube domains along the A-P axis |
WNT | Epithelial proliferation and development |

Cell Type | Function |
---|---|
Enterocytes | Most numerous cell type Nutrient absorption
|
Goblet cells | Secrete the mucus layer that protects the epithelium from the luminal contents |
Enteroendocrine cells | Secrete various gastrointestinal hormones
|
Paneth Cells | Produce antimicrobial peptides
|
Lymphocytes
| Found in lymphoid follicles (Peyer patches)
|
Microfold cells
| Sample antigens from the lumen and present them to the gut-associated lymphoid tissue (GALT)
|
Cup cells | No known function |
Tuft cells | Involved in immune response |
Intraepithelial lymphocytes | Located beneath the tight junctions, in between epithelial cells
|
The intestinal epithelium contains several types of cell junctions that are important for normal functioning. These junctions appear at the 10th week of gestation and continue to develop from that point on. There are four types of junctions, and their primary purpose is to maintain the mechanical and chemical barriers of the GI lumen ( Fig. 10.2 ). Desmosomes are complexes derived from cadherin and are located on the lateral sides of the enterocyte. They function to provide mechanical strength by linking adjacent cells by the cytoskeleton. Adherens junctions are multiprotein complexes that assist with epithelial cohesion, polarity, and cell migration. Gap junctions primarily allow for intercellular chemical communication via molecules, ions, and electrical impulses. Finally, tight junctions are composed of nearly 40 different proteins and are quite possibly the most important type of junction. The tight junction protein components form a continuous ribbon around the cells near the borders of the lateral and apical membranes and serve as a barrier between the GI lumen and the subepithelial environment by preventing the passage of a certain luminal antigens, microorganisms, and toxins. The intercellular components also interact with different scaffold proteins, adapter proteins, and signaling complexes that regulate the cytoskeleton and the polarity of the cell and are involved in cell signaling and trafficking and serve to provide selective paracellular transport of solutes. These tight junctions are dynamic and can adapt to different physiochemical properties in the intestinal lumen. Disruption in the integrity of the tight junction has been associated with many disease processes, including inflammatory bowel disease, celiac disease, and type 1 diabetes.

Microbiome and Diversity
Although the development of the microbiome has been recognized as critical to the normal development of the GI tract, it has only recently been noted that colonization may begin before birth. The microbiome continues to expand, and does so shortly after birth through the first 2 years of life. Typical progression begins with colonization of primarily facultative anaerobes, such as Streptococcus , Enterobacteriaceae, and Staphylococcus species within the hours following birth; this quickly expands to include Bifidobacterium and Lactobacillus within the first 48 hours of life. By age 3 months, regionalization of different bacterial species is noted with Fusobacterium and Eubacterium colonizing the colon and Enterobacteriaceae, Streptococcus , and Clostridium found in the ileum ( Fig. 10.3 ). Despite the presence of tight junctions, bacteria and their metabolic byproducts penetrate the submucosa; the interaction between the microbiome and the gut-associated lymphoid tissue (GALT) serves to shape the development of the gut mucosal immune system. This coevolution during postnatal life allows the host and the intestinal microbiome to coexist as symbionts, without limiting the immune response to pathogens. Failure to maintain this symbiotic relationship has been associated with diseases, including obesity, inflammatory bowel disease, atopic conditions, celiac disease, and necrotizing enterocolitis (NEC) in neonates.

Special Neonatal Challenges
In the neonatal population, a variety of environmental factors, such as mode of delivery, diet, and gestational age, can result in altered microbial establishment within the GI tract ( Fig. 10.4 ). Preterm neonates are more at risk for an altered microbiome because of their medicalized environment; these infants are exposed to pathogenic bacteria more frequently as a result of prolonged hospitalization within the neonatal intensive care unit (NICU). In addition, certain therapies, such as antibiotics, compound the issue by reducing species diversity so that preterm infants are often colonized by a less diverse quotient of bacteria. These environmental challenges often produce a microbiome with less beneficial bacteria (bifidobacteria, lactobacilli) and larger populations of potential pathogens ( Klebsiella , enterococci, staphylococci, Bacteroides , and Enterobacteria ). Clinically, this may have both short- and long-term effects; a growing number of studies have correlated prolonged courses of antibiotic therapy with increased incidence of NEC, sepsis, and death.

In addition to environmental challenges, the preterm neonate has less efficient immune function and abnormal mucosal barriers within the gut that allow for translocation of pathogenic bacteria and initiation of a systemic inflammatory response. Under normal circumstances, the intestinal epithelium serves a challenging role of facilitating transport of nutrients while maintaining a barrier against microorganisms. The physical barrier is composed of the epithelial cells and their tight junctions, as previously described. The intestinal epithelial cells are active members of this barrier; Paneth cells respond to certain signals and respond with antimicrobial peptides. Intestinal epithelial cells also express certain receptors that allow recognition of ligands produced by both commensal and pathogenic bacteria; the recognition of these ligands triggers a cytokine interaction between the intraepithelial lymphocyte and the underlying GALT. The immune response of the GI mucosa comprises both adaptive immune cells, such as effector T and B lymphocytes found in the GALT, as well as innate cells, such as dendritic cells, monocytes/macrophages, and the recently identified heterogeneous group of innate lymphoid cells. The preterm neonate has qualitatively or quantitatively less of these immune cells present.
There are many signaling pathways between the involved cells types; intestinal signaling through Toll-like receptors (TLRs) has been particularly well studied. Rakoff-Nahoum et al. were the first to demonstrate that intestinal signaling through TLRs stimulated by the intestinal microbiome is necessary for homeostasis. The intestinal immune cells must find a balance between mounting appropriate defenses against pathogenic organisms and developing tolerance toward commensal organisms. Excessive activation of the immune response would cause excessive production of inflammatory cytokines, resulting in local cell death and an inflammatory response. The TLR helps maintain balance through cell signaling in response to byproducts, such as lipopolysaccharides (LPSs) produced by commensal bacteria; this pathway ultimately enhances the ability of the epithelial cells to withstand chemical and inflammatory injuries. Significant differences have been identified in the degree of TLR function and TLR subtype activity in preterm infants and full-term infants. TLR4 activity has been noted to be highest in the preterm infant. This pathway is associated with epithelial apoptosis, mucosal barrier disruption, bacterial translocation, and reduced ability to recover from injury ( Fig. 10.5 ). Paneth cells also serve a role in tolerance of intestinal bacteria by producing antimicrobial proteins and peptides; cathelicidin (LL-37) is one such amyloid precursor protein, which functions to decrease inflammatory cytokine response to LPSs. Preterm infants appear to have a shift in balance, from an anti-inflammatory cytokine and protein environment to one that is more proinflammatory. Preterm infants have a higher expression of TLR4 activity, as well as interleukin-8 (IL-8) and IL-1. Protective LL-37 levels have been noted to drop off around 2 weeks after birth in mice models. Prenatal steroids increase intestinal maturation and reduce IL-8 production (via reduction in nuclear factor-κB nuclear translocation), which may help to explain, in part, why this prenatal therapy is associated with reduction in NEC. Overall, the immature innate immune system of the preterm neonate is often insufficient to adequately protect against the numerous challenges faced by it.

There are several basic physiologic mechanisms that are not fully functional in the preterm population, compared with those in full-term infants; these include peristalsis, gastric acidity, presence of proteolytic activity, intestinal mucus, cell-surface glycoconjugates, and intercellular tight junctions. There are immunologic differences, including decreased secretory immunoglobulin A (IgA) production and less antimicrobial peptides (specifically defensin) secretion by Paneth cells. These anatomic, physiologic, and immunologic discrepancies compound each other, resulting in a less capable intestinal mucosa that is more susceptible to damage, less able to heal itself, and more at risk for bacterial translocation. Clinically, this is thought to contribute to bacterial overgrowth, dysbiosis, and NEC. In summary, the initial development of a symbiotic relationship between the intestinal mucosa and the microbiome is critical to a neonate’s health. In preterm infants, several mechanisms, such as innate immune function and mucosal integrity, function either suboptimally or with a proinflammatory shift compared with their full-term counterparts, which increases the risk of sepsis, systemic inflammatory responses, and NEC.
Total Parenteral Nutrition
Many of the common interventions that are necessary for the care of preterm infants compound their innate challenges of establishing a healthy intestinal microbial relationship. Total parenteral nutrition (TPN) and lack of enteral feeds is one such factor. It is not uncommon for the preterm neonate, particularly the very low–birth weight (VLBW) and extremely low–birth weight infant, to require parenteral nutrition. Depending on the patient’s clinical status, enteral nutrition may be withheld for days to weeks. The lack of enteral stimulation by ingested nutrients ultimately alters normal gut physiology. Many of the gastric hormones are stimulated by the presence of intraluminal peptides, fatty acids, and glucose. Hormones, such as gastrin, cholecystokinin, motilin, and vasoactive intestinal polypeptide, help regulate normal intestinal function and are critical for trophic effects on the gastric mucosa, growth and function of the exocrine pancreas, intestinal motility, and pancreatic and intestinal secretions, respectively. With lack of enteral nutritional stimulation, there is an imbalance of normal neuroendocrine hormones, with subsequent malfunction of the GI tract. This has also been correlated with decreased secretory IgA and mucosal atrophy, with increased translocation of intestinal microbes and increased likelihood of systemic inflammatory response syndrome and sepsis. Given the degree of development of the neonatal GI tract, with crypt epithelium not fully developed until 30 weeks’ gestation, the lack of enteral stimulation may be even more detrimental to this population than in adults or older children. In addition to the immature epithelium, there is typically hypomobility of the peristaltic movements in the small bowel, and this may increase bacterial adherence and overgrowth. Several randomized controlled trials have shown that rapid advancement of enteral feeds is associated with a shorter time to full feeds and more rapid attainment of birth weight without increased incidence of NEC. Logistically, parenteral nutrition requires central venous access—with prolonged use being associated with late-onset sepsis. In addition to increased risks of inflammation and infection, differences in growth related to enteral nutrition versus parenteral nutrition have been demonstrated. Stoddart and Widdowson were among the first to note that suckling pigs gain 42% of their weight in the first 24 hours and that it did not occur in the nonsuckling animals. Other researchers have expanded on these findings to demonstrate differences in intestinal mucosal growth, hepatic and superior mesenteric artery (SMA) blood flow, intestinal motility, IgA secretion, and decreased permeability in infants receiving enteral feeds. Given the need for different nutritional components (amino acids, fatty acids, glucose) to stimulate various GI hormones, it is not surprising that the substrate chosen for enteral feeds also effects the intestinal mucosa. Breast milk has epithelial growth factor, erythropoietin, insulin-like growth factor, and anti-inflammatory IL-10—all of which may provide protective benefits, such as tissue growth and development while limiting inflammation. The use of standardized feeding protocols and enteral nutrition, as opposed to relying primarily on TPN, when possible, is recommended because it has been shown to be protective.
Antibiotic Use and the Microbiome
In a retrospective review, Clark et al. found that antibiotics are the most commonly used class of medications in neonates in the NICU. Four of the 10 most commonly used mediations noted in a large national data set were antibiotics, with the top two medications (ampicillin and gentamicin) having been used twice as often as the remainder of medications. The decision to administer antibiotics to the premature neonatal population is a challenging one. Ambiguous clinical signs of infection often overlap with noninfectious, typical behaviors in the VLBW preterm population. Significant limitations in the accuracy of adjunctive diagnostic laboratory tests for sepsis may, in part, explain why so many VLBW infants receive prolonged antibiotic courses. Interestingly, the true incidence of culture-proven early-onset bacteremia and sepsis may be lower than initially thought. A study by Stoll et al. showed that blood culture–proven early-onset sepsis was relatively uncommon—only present in approximately 1% to 2% of VLBW infants. The use of antibiotics has been associated with alterations in the gut microbiome, which may have several unintended consequences. Infants who receive >5 days of antibiotic therapy have lower bacterial diversity in subsequent weeks of life and a higher abundance of Enterobacter species. Preterm infants are often exposed to more pathogenic microbes as a result of their prolonged stay in the hospital environment. The use of broad-spectrum antibiotics may select a population of resistant organisms that can exacerbate lack of biodiversity and predilection toward a pathogenic microbiome. Several studies have correlated early antibiotic courses and longer duration of antibiotic exposure with increased incidence of NEC, sepsis, and death. Although it is not suggested here that antibiotic therapy not be used in preterm neonates, it is an area that deserves further investigation in the future because evidence suggests that antibiotic therapy is not wholly benign. It is important to balance the suspicion of a true infection with the possible negative short- and long-term effects of antibiotic use in this population.
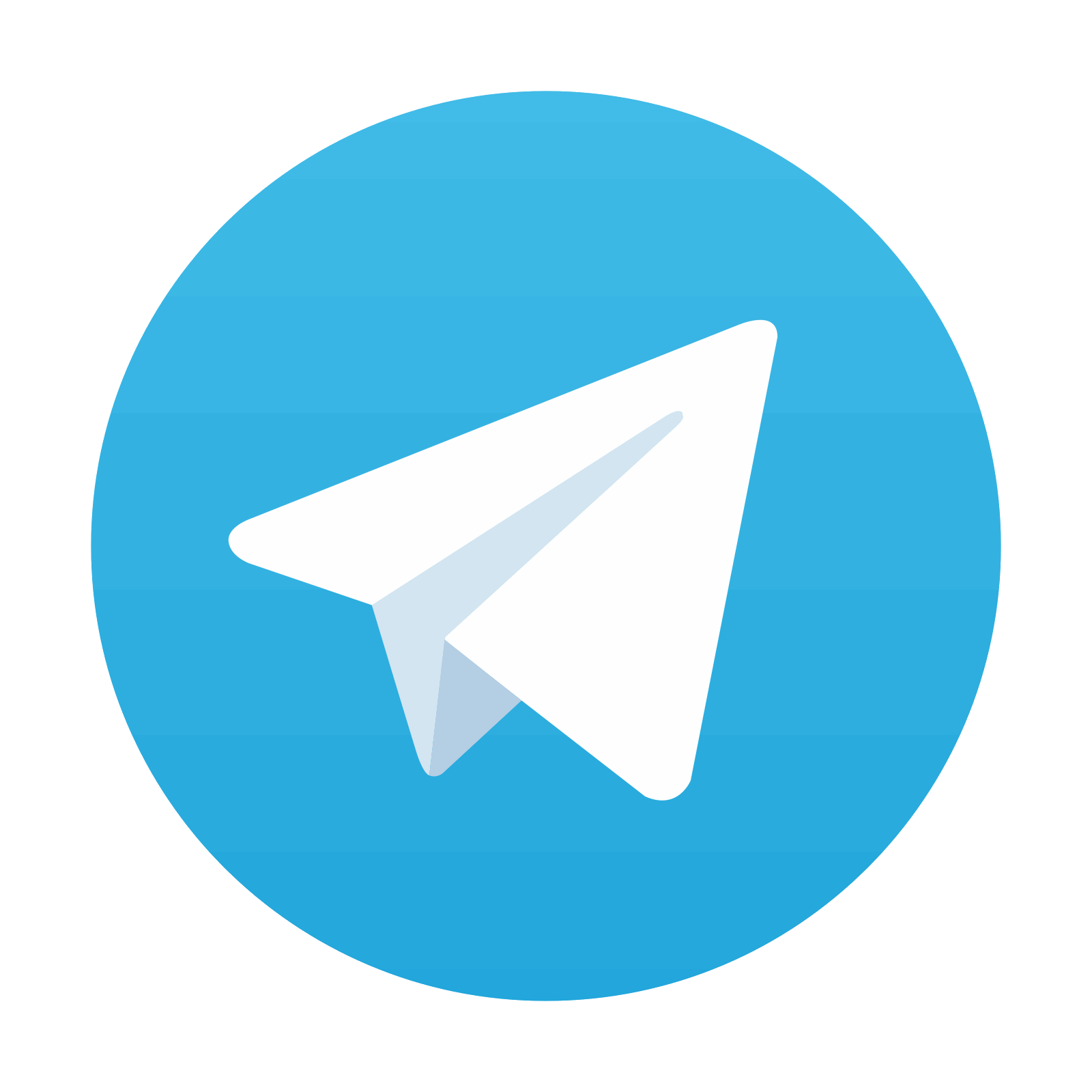
Stay updated, free articles. Join our Telegram channel

Full access? Get Clinical Tree
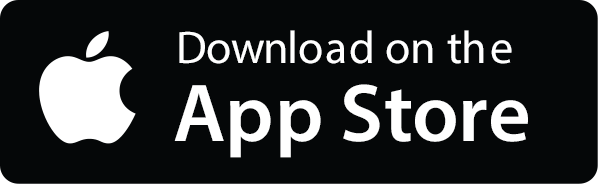
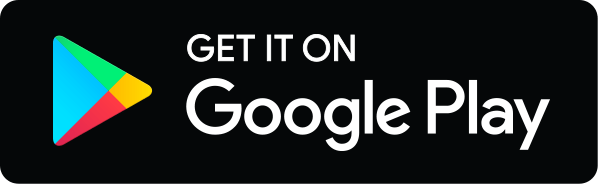